Abstract
Efficacious therapeutic options capable of resolving inflammatory lung disease associated with environmental and occupational exposures are lacking. This study sought to determine the preclinical therapeutic potential of lung-delivered recombinant interleukin (IL)-10 therapy following acute organic dust exposure in mice. Here, C57BL/6J mice were intratracheally instilled with swine confinement organic dust extract (ODE) (12.5%, 25%, 50% concentrations) with IL-10 (1 μg) treatment or vehicle control intratracheally-administered three times: 5 hr post-exposure and then daily for 2 days. The results showed that IL-10 treatment reduced ODE (25%)-induced weight loss by 66% and 46% at Day 1 and Day 2 post-exposure, respectively. IL-10 treatment reduced ODE (25%, 50%)-induced lung levels of TNFα (–76%, −83% [reduction], respectively), neutrophil chemoattractant CXCL1 (–51%, −60%), and lavage fluid IL-6 (–84%, −89%). IL-10 treatment reduced ODE (25%, 50%)-induced lung neutrophils (–49%, −70%) and recruited CD11cintCD11b+ monocyte-macrophages (–49%, −70%). IL-10 therapy reduced ODE-associated expression of antigen presentation (MHC Class II, CD80, CD86) and inflammatory (Ly6C) markers and increased anti-inflammatory CD206 expression on CD11cintCD11b+ cells. ODE (12.5%, 25%)-induced lung pathology was also reduced with IL-10 therapy. In conclusion, the studies here showed that short-term, lung-delivered IL-10 treatment induced a beneficial response in reducing inflammatory consequences (that were also associated with striking reduction in recruited monocyte-macrophages) following acute complex organic dust exposure.
Ethics statement
Neither human participants, data, nor tissues were used in these studies. The study was conducted and reported in accordance with ARRIVE guidelines (https://arriveguidelines.org). All animal procedures were approved by the University of Nebraska Medical Center (UNMC) Institutional Animal Care and Use Committee and were in accordance with NIH guidelines for the use of rodents.
Results
Lung-delivered IL-10 therapy reduces weight loss, serum pentraxin levels, and airway inflammatory cell influx following ODE exposure
In these experiments, mice were intratracheally-instilled with ODE at various concentrations (12.5, 25, 50%) followed by three doses of IL-10 or saline vehicle intratracheally instilled at 5, 24, and 48 hr following the single ODE exposure (schematic of experimental design: ). The results showed that treatment with IL-10 significantly reduced ODE-induced weight loss at Days 1 and 2 with the 25% ODE (p < 0.001) but not with the 12.5 and 50% ODE concentrations (). ODE increased serum levels of the murine acute phase reactant protein pentraxin-2 in a concentration-dependent manner; this response was reduced with IL-10 treatment for the 25 and 50% ODE concentrations (). There was a concentration-dependent increase in total airway cellular influx (marked by neutrophils and to a lesser degree macrophages) following ODE exposure (). Moreover, treatment with IL-10 decreased the influx of total number of BALF cells with all test ODE concentrations (). Neutrophil influx was decreased following IL-10 therapy at 25 and 50% (but not 12.5%) ODE concentrations, and BALF macrophages were reduced by IL-10 treatment for the 12.5%—but not the 25 and 50%, ODE concentrations. BALF lymphocyte levels were not significantly induced by ODE treatments. Eosinophils were rare and did not differ among treatment groups (data not shown).
Figure 1. Lung-delivered IL-10 therapy reduces weight loss, serum pentraxin-2 levels, and airway inflammatory cell influx following a one-time ODE exposure. (A) Experimental design schematic (created with BioRender.com). (B) Line graphs depict mean (± SEM) weight changes over time with ODE (12.5, 25, 50% concentration)-exposed mice treated with IL-10 vs. vehicle (Veh) vs. control (Cxn, saline treated mice, no ODE). (C) Scatter-dot plots depict mean with SEM bars among treatment groups of serum pentraxin-2 levels. (D) Total cellular influx and leukocyte cell numbers quantitated in bronchoalveolar lavage fluid (BALF). N = 4 (Cxn) and N = 5–9 (Veh, IL-10) mice/group. Statistical significance vs. Cxn (#p < 0.05, ##p < 0.01, ###p < 0.001, ####p < 0.0001); between groups (*p < 0.05, **p < 0.01, ***p < 0.001).
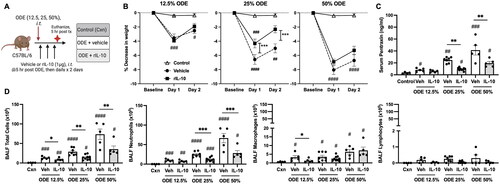
Lung-delivered IL-10 treatment reduces ODE-induced inflammatory analytes
In these mice, BALF and lung homogenates were examined for inflammatory markers consistent with airway inflammatory processes associated with agriculture-induced lung disease (American Thoracic Society Citation1998). At 48 hr post-ODE exposure, BALF levels of IL-6 and the murine chemoattractants (CXCL1 and CXCL2) were increased with all ODE concentrations; in comparison, levels of TNFα were elevated with 25 and 50%, but not 12.5%, ODE (). Levels of these airway inflammatory markers (TNFα, IL-6, CXCL1, CXCL2) were all reduced with IL-10 treatment for the 25% and 50% ODE exposures, with the exception that CXCL1 was only reduced with IL-10 treatment in the case of 25% ODE (). IL-10 treatment did not significantly alter BALF levels of these inflammatory cytokines/chemokines with hosts exposed to 12.5% ODE.
Figure 2. Lung-delivered IL-10 therapy reduces inflammatory cytokines/chemokines following a one-time ODE exposure. Levels of airway inflammatory markers determined by ELISA from (A) BALF and (B) lung homogenates. N = 4 (Cxn) and N = 5–9 (Veh, IL-10) mice/group. Statistical significance vs. Cxn (#p < 0.05, ##p < 0.01, ####p < 0.0001); between groups (*p < 0.05, ***p < 0.001, ****p < 0.0001).
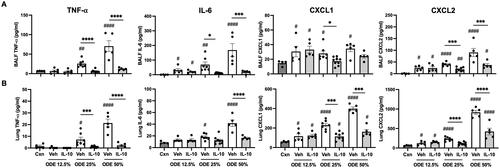
Lung homogenates exhibited a similar pattern in that levels of IL-6, CXCL1, and CXCL2 were increased in response to all ODE concentrations, while TNFα levels were increased with 25 and 50% (but not 12.5%) ODE (). Furthermore, IL-10 treatment profoundly reduced 25% and 50% ODE-induced lung homogenate TNFα as well as reduced 50% ODE-induced IL-6 and 25 and 50% ODE-induced CXCL1 and CXCL2 levels (). As expected in response to administration of IL-10, IL-10 levels were increased in both BALF and lung homogenates regardless of ODE concentration levels used in the exposures (Supplemental Figure 1).
Lung-delivered IL-10 treatment reduces ODE-induced lung neutrophil and recruited CD11cintCD11b+ monocyte-macrophage cell lung infiltrates
ODE exposure (at all concentrations) increased total lung cell infiltrates; this response was reduced by IL-10 treatment in mice exposed to 50% but not 12.5 or 25% ODE (). ODE-induced lung neutrophil infiltrates (defined as CD11c-Ly6G+) () were increased with all ODE concentration exposures, and this response was reduced by IL-10 treatment of hosts exposed to 25 and 50% ODE (). Repetitive ODE exposure is also known to increase CD11c+CD11b+ activated (Act) macrophage (MΦ) levels and induce recruited/transitioning CD11cintCD11b+ monocyte-MΦ infiltrates (Warren et al. Citation2017). In the current studies, a single ODE exposure increased CD11c+CD11b+ Act MΦ, CD11cintCD11b+ monocyte-MΦ, and CD11c-CD11b+ monocyte levels—with corresponding decreases in numbers of alveolar (Alv) CD11c+CD11b+ MΦ () in the lungs of the mice. Strikingly, there was a marked reduction in recruited/transitioning CD11cintCD11b+ monocyte-MΦ sub-populations with IL-10 treatment following exposure to any of the ODE concentrations (). IL-10 treatment did not affect levels of Alv MΦ, Act MΦ, or monocyte infiltrates following ODE exposure. Lung CD3+CD4+ and CD3+CD8+ T-cell and CD19+ B-cell infiltrates were increased by all ODE concentrations in the absence of IL-10; NK cells were increased with only the 50% ODE (Supplemental Figure 2). There were reductions in CD3+CD8+ T-cell populations due to IL-10 treatment of hosts exposed to 25 or 50% ODE (Supplemental Figure 2). Otherwise, ODE-induced lung lymphocyte infiltrates were not reduced by IL-10 treatment.
Figure 3. Lung-delivered IL-10 therapy following a one-time ODE exposure modulates total lung and myeloid cell infiltrates. (A) Scatter-dot plots depict mean (± SEM) total lung cells across treatment groups of control, ODE + vehicle, and ODE + IL-10 treatment. (B) Representative gate images for lung neutrophils in treatment groups after exclusion of debris, doublets, dead cells, and CD45- cells (with numbers of lung neutrophils [PMN; mean ± SEM] depicted in scatter-dot plots [C]). (D) Representative gate images for lung macrophage/monocyte Sub-populations in treatment groups: Alveolar macrophages (Alv MΦ) CD11c+CD11blo; Activated macrophages (Act MΦ) CD11c+CD11b+; Transitioning monocyte/macrophages (Mono-MΦ) CD11cintCD11b+; Monocytes (Mono) CD11c-CD11b+ after exclusion of debris, doublets, dead cells, CD45- cells, and neutrophils [numbers of Sub-populations depicted by scatter-dot plots] (E). Cell numbers determined by multiplying percentage of population from live CD45+ cells by total lung cells enumerated. N = 4 (Cxn) and N = 5–9 (vehicle, IL-10) mice/group. Statistical significance vs. Cxn (#p < 0.05, ##p < 0.01, ###p < 0.001, ####p < 0.0001); between groups (*p < 0.05, **p < 0.01, ****p < 0.0001).
![Figure 3. Lung-delivered IL-10 therapy following a one-time ODE exposure modulates total lung and myeloid cell infiltrates. (A) Scatter-dot plots depict mean (± SEM) total lung cells across treatment groups of control, ODE + vehicle, and ODE + IL-10 treatment. (B) Representative gate images for lung neutrophils in treatment groups after exclusion of debris, doublets, dead cells, and CD45- cells (with numbers of lung neutrophils [PMN; mean ± SEM] depicted in scatter-dot plots [C]). (D) Representative gate images for lung macrophage/monocyte Sub-populations in treatment groups: Alveolar macrophages (Alv MΦ) CD11c+CD11blo; Activated macrophages (Act MΦ) CD11c+CD11b+; Transitioning monocyte/macrophages (Mono-MΦ) CD11cintCD11b+; Monocytes (Mono) CD11c-CD11b+ after exclusion of debris, doublets, dead cells, CD45- cells, and neutrophils [numbers of Sub-populations depicted by scatter-dot plots] (E). Cell numbers determined by multiplying percentage of population from live CD45+ cells by total lung cells enumerated. N = 4 (Cxn) and N = 5–9 (vehicle, IL-10) mice/group. Statistical significance vs. Cxn (#p < 0.05, ##p < 0.01, ###p < 0.001, ####p < 0.0001); between groups (*p < 0.05, **p < 0.01, ****p < 0.0001).](/cms/asset/f7f5676a-bfc4-4444-8841-2f60d67c0d68/iimt_a_2332172_f0003_c.jpg)
Lung-delivered IL-10 therapy following ODE exposure modulates the immuno-phenotype of monocyte/macrophage sub-populations
In the next set of studies, the four monocyte (Mono)/Mɸ sub-populations (i.e. Alv MΦ, Act MΦ, Mono-MΦ, Mono) associated with a one-time ODE (25%) vs. saline (Sal) exposure were further assessed for extracellular/intracellular markers of cellular activation and immuno-phenotype (). F4/80, a marker of monocytes and macrophages, was universally-expressed on both Sal and ODE Mono populations, with a high expression demonstrated on Sal Alv MΦ and ODE Mono-MΦ sub-populations. F4/80 surface expression was down-regulated on ODE Act MΦ. CD206, an anti-inflammatory marker (also referred to as a classic ‘M2’ marker) (Roszer Citation2015), was highly-expressed on Sal Alv MΦ and nearly absent among all other monocyte/macrophage sub-populations. Ly6C, a monocyte and activation marker (Wang et al. Citation2018), demonstrated its highest expression on both Sal and ODE monocytes and its lowest expression on the Sal Alv MΦ. Ly6C expression was increased on ODE Act MΦ and ODE Mono-MΦ cells relative to that seen with Sal Alv MΦ, with ODE Mono-MΦ demonstrating increased Ly6C expression vs. ODE Act MΦ (albeit there was decreased expression of Ly6C with ODE Mono-MΦ vs. Sal and ODE Mono sub-populations). MHC Class II expression, important in antigen presentation (Roche and Furuta Citation2015), was highest on the ODE Act MΦ, but there was no difference in its expression between the Sal Alv MΦ and ODE Mono-MΦ. Whereas MHC Class II expression was lowest on monocytes, there was increased expression on ODE Mono vs. Sal Mono.
Figure 4. Lung-delivered IL-10 treatment following one-time 25% ODE exposure modulates immune phenotype of activated monocyte/macrophage Sub-populations. (A) Scatter-dot plots depict mean (± SEM bars) percent cell surface marker and intracellular cytokine (IL-10, TNFα) expression in monocyte/macrophage Sub-populations. N = 10 mice/group from two experimental runs. (B) Cell surface marker and intracellular cytokine percent expression of monocyte/macrophage Sub-populations exposed to ODE followed by treatment with vehicle (Veh) or IL-10. N = 5 mice/group from single experimental run. Statistical significance vs. Cxn (####p < 0.0001); between groups (*p < 0.05, **p < 0.01, ****p < 0.0001).
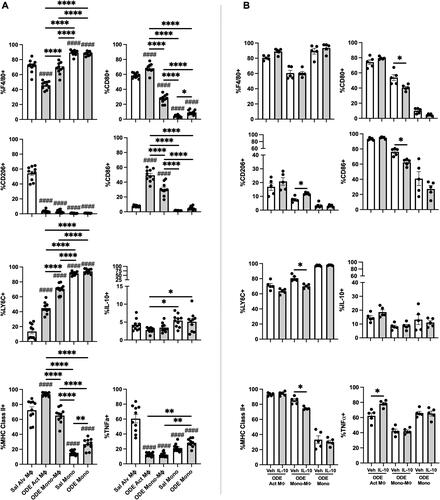
CD80 (co-stimulatory molecule) (Yang et al. Citation2022) was highly-expressed on both Sal Alv MΦ and ODE Act MΦ. The ODE Mono-MΦ sub-population demonstrated increased CD80 expression vs. both the Sal and ODE Mono populations and decreased expression vs. the Sal Alv MΦ and ODE Act MΦ. CD80 expression was increased on ODE Mono vs. Sal Mono. CD86 (co-stimulatory molecule) (Yang et al. Citation2022) expression was strikingly increased with ODE exposure in both ODE Act MΦ and ODE Mono-MΦ as compared to the Sal Alv MΦ, Sal Mon, and ODE Mono populations. A low proportion of cells across the monocyte/macrophage sub-populations exhibited intracellular IL-10 with both Sal and ODE Mono populations expressing increased IL-10 expression vs. ODE Act MΦ. As compared to Sal Alv MΦ, intracellular TNFα expression was reduced in ODE Act MΦ, ODE Mono-MΦ, Sal Mono, and ODE Mono; however, intracellular TNFα expression was increased in ODE Mono vs. in ODE Act MΦ and ODE Mono-MΦ.
The effect of IL-10 treatment on ODE-modulated immuno-phenotype markers on Act MΦ, Mono-MΦ, and Mono was also assessed (). Notably, the recruited ODE Mono-MΦ sub-population was significantly modulated by IL-10 treatment—as marked by increased expression (vs. levels seen with vehicle) of anti-inflammatory CD206 and decreased expression of Ly6C, MHC Class II, and co-stimulatory molecules CD80 and CD86. Intracellular TNFα expression was increased in ODE Act MΦ treated with IL-10 vs. in vehicle control. There were no differences in intracellular IL-10 and cell surface F4/80 expression with IL-10 treatment vs. vehicle across the other monocyte-macrophage sub-populations.
ODE exposure induces functional changes in phagocytic ability and reactive oxygen species (ROS) production of lung monocyte/macrophage Sub-populations
Phagocytic activity () and reactive oxygen species (ROS) production () induced by 25% ODE exposure in the four monocyte-macrophage sub-populations was assessed. Results are depicted as percent positivity of the specific cell population of phagocytic or ROS index using mean fluorescence intensity (MFI) of the specific cell population relative to the mean MFI of Sal Alv MΦ to normalize across experiments. The data show that there was an increase in the percentage of cells phagocytosing the fluorescein-labeled E. coli BioParticles in the case of the ODE Act MΦ and ODE Mono-MΦ relative to that of the Sal Alv MΦ. Whereas there was no difference in percent BioParticle phagocytosis between ODE Mono vs. Sal Mono, the percent phagocytosis was decreased in ODE Mono vs. Sal Alv MΦ. Phagocytic activity of individual cells, quantified by phagocytic index (specific cell MFI divided by Sal Alv MΦ MFI), demonstrated increased phagocytic activity among the ODE Act MΦ, ODE Mono-MΦ, and Sal Mono vs. Sal Alv MΦ.
Figure 5. ODE exposure induces functional changes in lung monocyte/macrophage Sub-population phagocytic ability and reactive oxygen species (ROS) production. (A) Represent-ative histograms depicting phagocytosed fluorescein-labeled E. coli BioParticles of saline (Sal) vs. ODE exposed monocyte-macrophage Sub-populations. Scatter-dot plots depict mean (± SEM) percent of cell Sub-population exhibiting BioParticle-specific fluorescence and the phagocytic index (mean fluorescence intensity (MFI) of positive BioParticle gate per Sub-population divided by MFI of Sal Alv MΦ positive BioParticle gate). (B) Representative histograms depicting ROS production determination by CellROX Deep Red fluorescence; scatter-dot plots depict percent of cell Sub-populations exhibiting CellROX Deep Red fluorescence and ROS index (MFI of positive CellROX gate per Sub-population divided by MFI of Sal Alv MΦ positive CellROX gate). N = 10 mice/group from two independent experiments. Statistical significance vs. Sal Alv MΦ denoted by ##p < 0.01, ###p < 0.001, ####p < 0.0001); between groups (*p < 0.05, **p < 0.01, ****p < 0.0001).
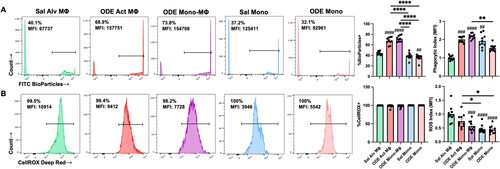
Monocyte/macrophage sub-populations exhibited ROS production (∼100%). However, differences in ROS MFI were demonstrated with reduced ROS MFI index demonstrated with ODE Mono-MΦ, Sal Mono, and ODE Mono vs. Sal Alv MΦ. Similarly, the Sal and ODE Mono sub-populations exhibited decreased ROS vs. ODE Act MΦ. There were no differences in phagocytic activity or ROS production among all four monocyte/macrophage sub-populations with IL-10 therapy relative to vehicle control following 25% ODE exposure (data not shown).
Lung-delivered IL-10 treatment reduces ODE-induced lung inflammation
Microscopic assessment of lung histopathology demonstrated that there was a reduction in ODE-induced bronchiolar and alveolar inflammation in mice that were treated with IL-10 as compared to what was seen with the vehicle controls (). Using semi-quantitative assessment, lung inflammatory scores were shown to be increased by the ODE exposure at all concentrations (vs. control) and IL-10 therapy reduced inflammation for mice exposed to 12.5 or 25%, but not 50%, ODE.
Figure 6. Treatment with IL-10 reduces ODE-induced lung inflammation. Representative H&E-stained lung section images from all treatment groups (ODE at various concentrations with IL-10 or saline vehicle treatment) and saline control. Scatter-dot plot depicts mean (± SEM) bars of semi-quantitative lung inflammatory scores per experimental treatment group. Individual values are averaged from 8 to 10 images/section/mouse. Statistical significance vs. Cxn (#p < 0.05, ##p < 0.01); between groups (*p < 0.05, **p < 0.01). Line scale is 100 μm.
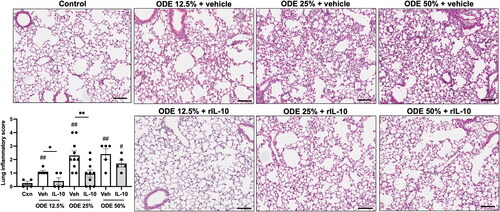
Discussion
Occupational and environmental factors are a significant cause of debilitating lung diseases worldwide (Gorguner and Akgun Citation2010; Seaman et al. Citation2015). Novel therapeutic strategies are necessary for at-risk persons, and previous murine studies have demonstrated that short-term treatment with lung-delivered IL-10 reduced adverse lung effects induced by an acute LPS exposure (Poole et al. Citation2022). Here, preclinical animal studies demonstrated that short-term, lung-delivered IL-10 administration following various concentrations of a complex agriculturally-derived ODE exposure reduced ODE-induced systemic and lung inflammatory consequences. These beneficial effects with IL-10 therapy included blunting of ODE-induced weight loss, with corresponding reductions in ODE-induced acute phase protein pentraxin-2, airway pro-inflammatory cytokine/chemokine levels, neutrophil and recruited/transitioning CD11cintCD11b+ monocyte-MΦ infiltrates, and lung pathology. The study also showed ODE exposure significantly modulated the immuno-phenotype and function of lung monocyte-MΦ sub-populations and that IL-10 then impacted this outcome. Specifically, IL-10 treatment increased anti-inflammatory CD206 expression and reduced pro-inflammatory (Ly6C) and antigen presentation (MHC Class II, CD80, CD86; M1-like) markers on the ODE-associated recruited/transitioning CD11cintCD11b+ monocyte-MΦ sub-population. Collectively, these findings demonstrated the potential therapeutic benefit of short-term lung-delivered IL-10 in the context of an acute environmental and occupational exposure.
The focus of therapeutic approaches to treat environmental and occupational-associated diseases has centered on specific agent identification and risk reduction measures and mitigation (Schwab and Poole Citation2023). With a specificity for agriculture work settings, promotion of personal respiratory protection and exposure reduction measures are recommended. By using large animal (swine) confinement facility organic dust as a prototypical dust at escalating concentrations (i.e. 12.5, 25, 50%), our studies demonstrated clear dose-response systemic and airway inflammatory effects; these outcomes underscore the importance of ongoing efforts to reduce and prevent high-level exposures. However, the use of respiratory protection devices has been extremely low in agriculture work (<1%) (Davidson et al. Citation2018), though it was recently reported that 15.5% of young adult hog producers (age 18–30 years) state that they ‘always’ wear a N95 filtering facepiece respirator (Gibbs et al. Citation2023).
Moreover, it is also recognized that dust and endotoxin (surrogate of bacterial exposures) exposures in agriculture work are highly variable and often exceed occupational exposure limits by several orders of magnitude (Donham et al. Citation1995, Citation2000; Reynolds et al. Citation1996, Citation2013; Alvarado and Predicala Citation2019). For example, a recent study reported that a high proportion of United States dairy farm workers (89%) were exposed to inhalable endotoxin exceeding the occupational exposure limit with a geometric mean exposure of 438 EU/m3, 7-times the recommended average 54-hr workweek exposure (Davidson et al. Citation2018). Endotoxin concentrations in modern swine confinement facilities across the world have reported average levels up to 443 EU/m3 and 1260 EU/m3 of total dust (Roque et al. Citation2018). Thus, these current animal modeling concentrations would have relevance among animal production workers and furthermore highlight the unmet need to explore novel treatment strategies. Importantly, these current results show a striking beneficial effect of IL-10, even under these high experimental modeling conditions.
Lung monocytes-macrophages play a critical role in mediating responses to inflammatory bioaerosol exposure and recruited/transitional monocytes-macrophages are implicated in the transition of acute inflammation to lung fibrosis in animal models and clinical investigations (Misharin et al. Citation2017; Verjans et al. Citation2018). Given the plasticity of lung monocytes/macrophages and their ability to elicit pro-resolving or injurious functions, these cells represent modifiable targets to mitigate tissue damage while hastening resolution. Here, lung-delivered IL-10 therapy profoundly reduced recruitment of the transitional CD11cintCD11b+ monocyte-Mɸ in the case of all ODE test levels used, findings consistent with observations previously-reported following acute inhalant LPS exposure (Poole et al. Citation2022). This might be explained by IL-10 exerting an inhibitory effect on monocyte adhesion to endothelial cells, thus disabling monocyte recruitment to areas of inflammation (Mostafa Mtairag et al. Citation2001). In addition, IL-10 can block monocyte activation and proliferation, as well as prevent monocyte maturation and effector capabilities (Mu et al. Citation2005).
Lung monocyte-macrophage sub-populations are characteristically heterogeneous, with functional attributes that can be referred to as M1 (inflammatory) and M2 (anti-inflammatory, pro-fibrotic)-like macrophages (Azad et al. Citation2014). CD206 is a mannose receptor ubiquitously-expressed by normal human alveolar macrophages and recognized as a classic M2 marker (Bharat et al. Citation2016). Here, its exclusive expression was observed on control (saline) alveolar macrophages (Sal Alv MΦ) and its near-absent expression on ODE-associated Act MΦ and Mono-MΦ sub-populations. Such findings reflect a shift away from an M2-like macrophage. Whereas Ly6C expression is expressed on circulating monocytes, Ly6C up-regulation (as seen with ODE Act MΦ and ODE Mono-MΦ sub-populations) can also signify an activated macrophage state with inflammatory features (M1) (Yang et al. Citation2021). Interestingly, Ly6Chi monocyte-macrophages have been characterized as pro-fibrotic in the liver (Ramachandran et al. Citation2012); in the lung, depletion of circulating Ly6Chi monocytes reduces progression of bleomycin-induced pulmonary fibrosis (Gibbons et al. Citation2011). Future studies are warranted to target these transitioning Mono-MΦ with Ly6Chi expression to fully understand their role in lung fibrosis.
Correspondingly, recruited ODE Mono-MΦ demonstrated increased antigen-presenting molecule (i.e. MHC Class II, CD80, CD86) expression as compared to lung monocytes, but expression of these molecules was not to the degree observed with the ODE Act MΦ. The exact importance of this intermediate phenotype remains to be determined, but it appears to skew toward a M1 phenotype; moreover, these antigen-presenting molecules were down-regulated with IL-10 treatment in the CD11cintCD11b+ Mono-MΦ. It should be noted that intracellular TNFα stores were reduced in ODE Act MΦ and ODE Mono-MΦ (vs. Sal Alv MΦ)—with corresponding increases in levels of extracellular TNFα airway levels—after ODE exposure. Moreover, IL-10 treatment increased intracellular TNFα stores in the ODE Act MΦ sub-population; this might be explained by IL-10 actions that inhibit release of pre-formed TNFα mediator stores (Armstrong et al. Citation1996). It is known that macrophage TNFα production and its downstream effects can be regulated by IL-10 as macrophage IL-10 activation inhibits the TNFα converting enzyme (ADAM-17) [through activation of TIMP3] to effectively shut down exposure-induced Toll-like receptor pathway activated TNFα release (Wyatt et al. Citation2020).
ODE exposure primed or enhanced phagocytic responses in the Act MΦ and Mono-MΦ sub-populations (vs. in Sal Alv MΦ). This finding extends in vitro observations that LPS (a component within ODE) increases the phagocytic ability (Islam et al. Citation2013) of lung-specific monocyte/macrophage sub-populations (Frevert et al. Citation1998). Here, IL-10 therapy did not alter or negatively affect ODE-mediated enhanced phagocytic ability, thus allowing for preservation of this important function used in host clearance of lung particulates/debris. All lung monocyte-macrophage sub-populations here exhibited normative ROS production, but the magnitude of this response (ROS index) was reduced in the more immature sub-populations (i.e. Mono-MΦ and monocytes). Correspondingly, there was no effect on ODE-dependent ROS production with IL-10 therapy.
In summary, the present findings support potential application of lung-delivered IL-10 therapy, particularly in the short-term, following an acute high-dose occupational/environmental inhalation exposure to organic dusts. Ultimately, such findings may go a long way to help reduce respiratory disease burdens in at-risk individuals.
Nevertheless, this study does have limitations. Whereas these studies may not reflect the exact human experiences in the field, the complexity intrinsic to the ODE is compositionally representative of real-world agriculture organic dust exposure. Future studies should also investigate effects of IL-10 therapy at potentially longer duration of use as well as in the setting of repeated occupational exposures and potentially as a prophylactic approach. It is noted that others have demonstrated that airway injury was reduced when human IL-10 expression was delivered by adenoviral vector treatment to the murine lung prior to LPS exposure (Garantziotis et al. Citation2006). Comprehensive assessment of the kinetics (e.g. deposition, lung cell uptake, degradation, and clearance) of IL-10 after administration in this setting is not known. Future studies should explore various approaches to enhance IL-10 drug delivery including novel nanoparticle formulations to maximize lung deposition and minimize pulmonary clearance of drugs for optimal lung-delivery strategies. Systemic IL-10 has been well-tolerated in human clinical interventions; however, anemia and thrombo-cytopenia have been reported with long-term use (Fioranelli and Grazia Citation2015). Thus, short term lung-delivery could potentially reduce untoward effects.
Acknowledgements
The authors acknowledge members of the Tissue Sciences Facility at the Department of Pathology and Microbiology (University of Nebraska Medical Center, Omaha, NE) for assist-ance with tissue processing and staining. We thank Victoria B. Smith, Holly Britton, and Craig Semerad in the Flow Cytometry Research Core Facility at the University of Nebraska Medical Center for aid in flow cytometry studies. Schematics were created using www.BioRender.com.
Disclosure statement
Author J.A.P. received research reagent from AstraZeneca (no monies) and has been a site investigator for allergy and asthma clinical studies for Takeda, GlaxoSmithKline, Regeneron, and AstraZeneca (no monies). Authors A.D.S., R.G., A.J.N., A.G., D.J.R., T.A.W. do not declare any competing interests.