Figures & data
Figure 1. The genomic organization of snoRNAs across eukaryotic model organisms. (a) SnoRNAs can be encoded as independently transcribed units in intergenic regions, either alone as mono-intergenic snoRNAs or as co-transcribed intergenic snoRNA clusters. They can also be embedded within host genes, usually within their introns, relying on their host gene transcription to be expressed. Mono-intronic snoRNAs are encoded alone in their intron whereas some snoRNAs co-exist within the same intron, thereby forming intronic snoRNA clusters. Some snoRNA genes also overlap exon sequences in host genes, either alone as mono-exonic snoRNAs or with others thereby forming exonic snoRNA clusters. The exonic snoRNAs are marked with an asterisk, because they are quite rare and might be artifacts due to genomic annotation errors (e.g. an overlapping exon in the form of a retained intron might not actually exist, therefore making intronic snoRNAs appear as ‘exonic’). (b) The distribution of genomic localization of snoRNAs across animal species is represented as a grouped stacked bar chart. The species are sorted from left to right in decreasing order of the total number of snoRNAs annotated in that species, with the total number being represented in parentheses above the bar charts. The right bar for each species represents the stacked proportion of snoRNAs localized in one of the different organizations displayed in (a). The left bar represents the stacked proportions of snoRNAs of each type (C/D box, H/ACA box or unknown type) that compose each genomic organization represented in the right bar. The distribution of host gene biotype (i.e. protein-coding gene, non-coding gene or intergenic snoRNA) for all snoRNAs in a given species is represented as a donut chart above its respective bars. The represented animal species are the platypus (Ornithorhynchus anatinus), human (Homo sapiens), rat (Rattus norvegicus), mouse (Mus musculus), macaque (Macaca mulatta), frog (Xenopus tropicalis), worm (Caenorhabditis elegans), fruit fly (Drosophila melanogaster), zebrafish (Danio rerio) and chicken (Gallus gallus). (c) Same as in (b), except that the chosen species are from the plant, fungal and protist eukaryotic kingdoms. The species are still ordered by decreasing total number of annotated snoRNAs, but the sorting was applied within individual kingdoms. The represented plant species are the wheat (Triticum aestivum), thale cress (Arabidopsis thaliana) and rice (Oryza sativa); the represented fungal species are the budding yeast (Saccharomyces cerevisiae), Candida albicans, fission yeast (Schizosaccharomyces pombe) and Neurospora crassa; the represented protist species are Dictyostelium discoideum, Tetrahymena thermophila and Giardia lamblia. Of note, no snoRNA is annotated in G. lamblia, resulting in the empty bar and donut charts. The methodology relevant to the results presented in this figure is detailed in the Methods in the Supplementary Material.
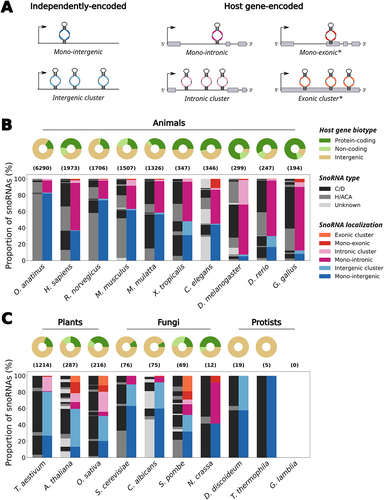
Figure 2. The maturation steps of different host-derived snoRNA hybrids. Host gene transcripts can harbour one or multiple (shaded) snoRNAs in their intron (see (a)). In the case of a snoRNA encoded alone in its intron, its canonical maturation requires the transcription and splicing of its host gene, which results in a snoRNA-containing lariat as well a mature host transcript. The lariat is typically debranched and its ends are then degraded by exonucleases up to the snoRNA ends which are bound by core proteins, thereby protecting the mature snoRNP from further cleavage (see (b)). When two snoRNAs are encoded in the same intron, the same maturation steps can lead to the formation of snoRNA-ended hybrids called sno-lncRNAs including SLERT (see (f) and (g)), a H/ACA sno-lncRNA, as well as the lncRNA LNC-SNO49AB (see (c)). SLERT was shown to interact with DDX21 and regulate cell proliferation, whereas LNC-SNO49AB was observed to bind with ADAR1 and promote its dimerization and activity. Other sno-lncRNAs have also been found to interact with splicing factors. On the other hand, atypical branch points (cytidines instead of adenosines) can hinder lariat debranching, thus leading to the formation of stable lariats bearing a snoRNA (slb-snoRNAs) which can be actively transported to the cytoplasm (see (e)). In addition, transcript readthrough of host genes can lead to the production of nuclear 5’ snoRNA-ended and 3’ polyadenylated lncRNAs (SPAs) that were shown to interact with splicing factors (see (d)). One example of SPA was also shown to be exported to the cytoplasm (SNORD86 cSPA) (see (i)). The retention of introns harbouring a H/ACA box snoRNA leads to the generation of snoRNA retaining transcripts (snoRTs), which are exported to cytoplasm and vary in length with regards to their 5’ end (see (j)). Finally, knocking down splicing factors was shown to induce splicing defects including the formation of hybrid mRNA-snoRNA (hmsnoRNAs) transcripts, which were observed to be either degraded or stabilized in the cytoplasm (see (h)). Of note, dotted lines represent the possible path that a host-derived snoRNA extension can take, whereas full lines represent an obligatory step in its maturation pathway. The asterisk that marks the name of certain snoRNA-containing hybrids (i.e. initial host transcript, mature snoRNP, slb-snoRNA and hmsnoRNA) signifies that the snoRNA represented in the hybrid can either be a C/D box or H/ACA box snoRNA, although only C/D box snoRNAs are represented in those cases for clarity.
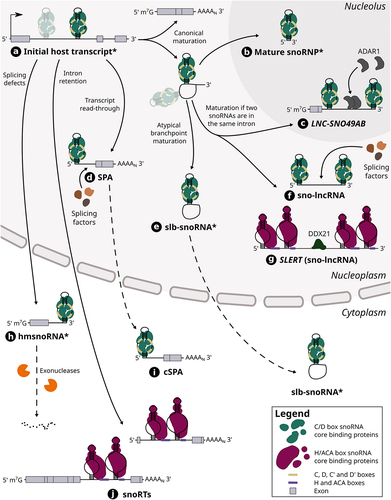
Supplementary_tables_S1_S2_Methods.pdf
Download PDF (1.6 MB)Data availability statement
The authors confirm that the data supporting the findings of this study are available within the article and its supplementary materials.