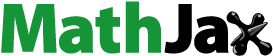
ABSTRACT
In chronic inflammatory conditions and diseases accompanied by inflammation, the main indication range of systemically administered ozone, one basic mechanism of action has emerged following the low-dose ozone concept: Interference in the diverse biological redox equilibria, and its regulation at low concentrations. the very special role of the glutathione balance and its recovery. It starts with the oxidation of GSH (active form of glutathione) by “ozone peroxides”, and after a considerable number of regulatory processes and mechanisms, finally leads to the regulation of the glutathione balance GSH ⇋ GSSG itself, the most important intracellular antioxidant system. Typically, the upregulation of GSH is demonstrated here in aging processes, in rheumatoid arthritis, the protection of liver intoxication and in post COVID patients. From this perspective, the redox bioregulator ozone and its fundamental reactions are reconsidered in this article.
Introduction
Until recently, systemically administered ozone has been considered to be an oxygen supplier and researchers focused on measuring the partial pressure of oxygen in blood and tissues, especially in diabetic patients with diabetic gangrene, then the main indication of medical ozone.
Although 2,3-diphosphoglycerate (2,3-DPG) increases in the RBC´s (Red Blood Cells) through systemic ozone treatment shifting the oxygen binding curve to the right, releasing oxygen more easily due to a reduction of the Hb-O2 affinity and thus associated with improved oxygen delivery, the partial pressure of oxygen, measured transcutaneously in peripheral hypoxemic tissue, may actually decrease initially.
The cause was soon shown to be a general activation of cell metabolism with a correspondingly increased oxygen demand, verified in the 1990s by activation of immunocompetent cells, mainly lypmphocytes (Rokitansky et al. Citation1981; Washüttl and Viebahn Citation1986. (not peer reviewed) (Hoffmann and Viebahn Citation2001; Viebahn et al. Citation1995), and (Hoffmann and Viebahn Citation2002). (These references refer to proceedings of Ozone World Congress of the International Ozone Association (IOA) and are not peer reviewed) (Bocci et al. Citation1993; Lell, Viebahn and Kremsner Citation2001).
In a search for a deeper understanding of the biochemistry and pharmacology of medial ozone, the influence on and regulation of redox balances has emerged as the main cause of action: Redox Bioregulation (Léon et al. Citation1998). Indications were more clearly specified, forms of application standardized, and concentration and dosage ranges defined and laid down in guidelines (Viebahn-Haensler, Sonia León Fernández and Fahmy Citation2012).
Redox Bioregulation starts with the oxidation of GSH (the active form of glutathione) by “ozone peroxides,” and after a considerable number of regulatory processes and mechanisms: activation of nuclear factors, such as NfkB (nuclear transcriptional factors) and Nrf2, transcription of antioxidant response elements, translation resulting in the production of antioxidant enzymes, like superoxide dismutase SOD, GSH peroxidase and reductase, catalase (CAT) and others (Sagai and Bocci Citation2011), finally leads to regulation of the glutathione balance GSH GSSG (reduced glutathione
oxidized form), the most important intracellular antioxidant system.
This is the content and intention of our publication on the basis of well measurable and well reproducible reference parameters, elaborated in preclinical and clinical trials.
Oxygen in the biological system
Aspects of oxygen in living systems as thermodynamically open systems:
The biochemistry of life is a kaleidoscope of dynamic processes, dynamically interacting equilibria in a highly complicated network.
None of the classical thermodynamic laws is valid or applicable here, not even the famous 2nd law, the entropy law. Classical thermodynamics applies only to closed or isolated systems, whereas a living, biological system is an open one exchanging energy and matter with the environment to maintain the dynamic equilibria network and biochemical structures of the organelles, schematically shown in .
Figure 1. Schematic illustration of a dynamically interacting network of biochemical equilibria and its disturbances. If individual cycles, are disturbed, this biological network is still able to repair defects and to keep the overall system viable, e.g. In cases of moderate oxidative stress. Reactivating biological processes and, if possible, restarting repair mechanisms is the aim of biological medicine.

Oxygen is one of the main energy suppliers in the biological system, ubiquitous and constantly reproduced by photosynthesis in the environment. And the high energy demand of the organism is largely met by O2- dependent oxidation in exergonic (energy delivering) redox reactions.
Essentially, these redox reactions (no oxidation without reduction) occur in the mitochondrial respiratory chain, where substrates provided via the citrate cycle are oxidatively metabolized, and in a healthy biological system, 4 electrons are transferred to an O-O molecule to form 2 O2- with H2O as end product.
2 electrons (e−) are supplied via complexes I to III, and the reduction is finally completed via cytochrome C involving cytochrome C oxidase, the “Warburg respiratory enzyme” as complex IV of the respiratory chain:
O2 +4 e− 2 O2- ; 2 O2- +4 H+
2 H2O
One example: Glucose (carbohydrate) + oxygen:
C6H12O6 +6 O2 6 H2O +6 CO2
H
−112 kcal/mol O2
673 kcal are delivered per mol of glucose, stored as biochemical energy in the form of 36 ATP per mol glucose.
Oxygen, a JanusFootnote*-headed molecule. The oxidation of substrates, such as carbohydrates, fat and proteins, with O2 covers a huge amount of energy demand as long as the living system is healthy.
In a number of chronic diseases, we are dealing with a mitochondrial dysfunction, which manifests itself e.g. in a defect of complex IV and an incomplete electron transfer to oxygen; as a result, only one electron is transferred and superoxide radicals •O-O −– are formed, the starting molecule for a variety of Reactive Oxygen Species ROS: such as hydrogen peroxide H2O2, ROOR (lipid peroxides) and other sequel products; ONO-O − with an excess of nitric oxide, also called nitrosative stress NOS: or O&NS = oxidative + nitrosative stress. A crucial end product, the hydroxyl radical •OH, reacts in a completely nonspecific manner with an extremely short half-life of 10−9seconds or less (Sies Citation1999) and is responsible for a considerable number of degenerative processes and degenerative diseases.
Reactive oxygen species (ROS)
In case of an infection by bacteria, viruses or parasites, superoxide radicals and finally •OH radicals, produced in activated lymphocytes, neutrophiles, macrophages and other phagocytic cells, are life-saving molecules due to their high redox potentials and correspondingly enormous destructive potential. Without them, a banal infection can become a lethal one. That is why this biochemical mechanism is the favored one used in a variety of chemotherapeutics in oncology: anthracyclines, platinum compounds, vinca alkaloids, alkylation agents and others (Yang et al. Citation2018). The anthracyclines, such as Doxorubicin (Mizutani et al. Citation2005), Adriamycin, Epirubicin, produce the highest level of ROS; mechanisms are discussed in Viebahn-Haensler (Citation2005).
An excess of ROS is controlled through redox bioregulation by cellular antioxidants, especially superoxide dismutase SOD, Catalase CAT, glutathione peroxidase GSHpox and others, some of which are shown in (Chow Citation1984).
Table 1. The biological antioxidants control the reactive oxygen species ROS, i.e. The oxidative stress situation. H2O2 is controlled by the glutathione system and SOD, whereas the „ozone peroxides“ R-CH(OH)-OOH is only catalyzed by glutathione GSH/GSSG, not by SOD or catalase.
In a biological system, each molecule is Janus-headed with positive and deleterious side depending on its concentration and regulation.
Reactive oxygen species ROS also act as signal molecules, able to induce the production of antioxidants and to start redox regulation in order to maintain the integrity of the cell.
The regulatory task of H2O2 as one of the most important bioregulators, well researched and documented (Sies Citation2021; Sies and Jones Citation2020). At concentrations on a biological level, oxidative eu-stress will dominate and lead to redox signaling, redox regulation and physiological processes.
When and if the H2O2 concentration becomes too high, as is the case in chronic inflammatory diseases and excessive sports such as marathons, an oxidative dis-stress will block the regulation, consequently initiating pathophysiologic processes, such as inflammatory conditions and chronic inflammatory diseases, such as age related diseases, rheumatoid arthritis, diabetic angiopathy, cancer and others.
And finally, the GSH/GSSG balance is shifted to the right at the expense of active GSH with a significantly reduced antioxidant capacity.
The biological antioxidants control the reactive oxygen species ROS, i.e. the oxidative stress situation. H2O2 is controlled by the glutathione system and SOD, whereas the ozone peroxides R-CH(OH)-OOH is only catalyzed by glutathione GSH/GSSG, not by SOD or catalase.
Typical diseases in GSH deficiency are:
chronic inflammatory diseases
cancer diseases, especially age-related
aging processes
age-related diseases
All these diseases are associated with chronic oxidative dis-stress and the redox balance is no longer controlled (Sies Citation1999).
Ozone as redox-bioregulator
“Ozone peroxides” and the glutathione balance
Interestingly enough, diseases involving GSH deficiency are exactly those typical ozone indications, which are always accompanied by high oxidative stress, low antioxidant capacity and an imbalanced redox regulation.
H2O2 and other ROS in excess block the regulation and are no longer capable of signal transduction in order to upregulate the antioxidative enzymes and recover GSH equilibrium, and here the “ozone peroxide” becomes involved and slips into the place of H2O2 replacing the biological role of hydrogen peroxide, and takes over its signal transduction as shown in principle in and the reaction of GSH with ozone peroxide in . The complete overview is given in .
Figure 2. Hydrogen peroxide as a key regulator in the biological system (1) low, biological H2O2 concentrations produce positive oxidative stress; reduced by the glutathione system it starts the regulation of physiological processes via redox signaling. H2O2 concentrations in excess, oxidative dis-stress, overstrain the glutathione balance, block the regulation and induce pathological, inflammatory processes leading to chronic inflammation (Sies and Jones Citation2020). low-dose ozone as a bioregulator (2) in chronic inflammatory diseases, one of the main indications of medical ozone, where the H2O2 concentration is of a pathological level, the glutathione balance is disturbed and the regulation is blocked. Here the “ozone-peroxide” at low concentrations can replace H2O2 and reorganize the GSH/GSSG balance by redox signaling and regulation of the enzymatic antioxidants, thus downregulating oxidative dis-stress (Viebahn-Haensler and León Fernández Citation2021). Reaction of glutathione with ozone peroxide.

Figure 3. NFkB and Nrf2 cross talking: low or moderate oxidative stress induces an inflammatory response via nuclear factor NFkB, and in a second step, the anti-inflammatory response through the regulation of enzymatic antioxidants via Nrf2 (Togi et al. Citation2021).

This biochemical mechanism is the gateway to the redox bioregulation, to be followed by appropriate parameters for each indication, works as described above only at small concentrations and forms the basis of the low-dose ozone concept (Viebahn-Haensler, Sonia León Fernández and Fahmy Citation2012). Recommended reference parameters as oxidative stress parameters are as follows: Total hydroperoxides TH, malondialdehyde MDA, nitrogen monoxide NO, -glutamyl transferase
-GT (among others) and, on the other side, the antioxidants: total SOD superoxide dismutase, GSH reduced form of glutathione and others (Viebahn-Haensler and León Fernández Citation2021).
To verify this basic biochemical situation, we focus here mainly on GSH, the active form of glutathione, which increases in favorable cases, but must never decrease during treatment; this would be a sign of overdose or in state in which systemic ozone administration is contraindicated.
Ozone peroxides versus long-chain peroxides
Formed as reaction product from O3 and unsaturated fatty acids with an isolated double bond we are dealing with a short-chain peroxide with a highly reactive -C(OH)-COOH group.
Long chain peroxides as they are formed during oxidative stress from polyunsaturated fatty acids PUFA and OH-radicals + oxygen are stable, long living and suitable parameters in the measurement of chronic oxidative stress (Corteselli et al. Citation2019; Weitzel et al. Citation1961). They react easily to form radicals starting a radical chain reaction and further oxidative stress to overwhelm the cellular antioxidant response ().
Table 2. The hydroxy hydroperoxides, here „ozone peroxides“are understood as the pharmacologically active substances in the systemic ozone application contrary to autoxidation as radical reaction process.
Following the low-dose ozone concept with a concentration range of 10 to 40 g/mL, ozone is nontoxic, behaves as bioregulator and fulfills the aim of biological medicine: The correction of disturbed biochemical balances and induction of the biological repair mechanisms in the network of an endless number of interconnected dynamic reaction equilibria, schematically shown in .
Nuclear factors
Essentially involved in the downstream regulation are the nuclear factors NFκB (Nuclear factor kappa-light-chain-enhancer of activated B cells) and Nrf2 (Nuclear factor erythroid 2-related factor 2), in order to maintain the homeostasis of a living system. Both are activated by low ozone concentrations as low or moderate oxidative stressor, NFkB as inflammatory and Nrf2 as anti-inflammatory response. This has been verified by several research groups (Galiè et al. Citation2019; Re et al. Citation2014; Sagai and Bocci Citation2011) and quite recently specified in HeLa cells as far as the ozone concentrations are concerned, and the interaction and crosstalk between Nrf2 and NFkB. Most likely the NFkB activation is the first step measured as increase in HO-1 (hemoxigenase-1) inducing Nrf2 activation as anti-inflammatory response (Togi et al. Citation2021) displayed in .
Preclinical and clinical trials. Discussion
Low-dose ozone application in the context of glutathione in typical indications with GSH deficit
In , preclinical and clinical ozone trials are listed in diseases with GSH deficiency: Nephrotoxicity (Ajamieh et al. Citation2004), CCl4 liver intoxication (Candelario-Jalil et al. Citation2001) and liver reperfusion damage (Léon Fernande Olga et al. Citation2007), aging processes (Safwat et al. Citation2014), alcohol withdrawal (Díaz-Soto et al. Citation2012), rheumatoid arthritis (León et al. Citation2016). A GSH-deficit starts at the 100% baseline. Systemically administered ozone increases reduced glutathione GSH, a sign of its intervention in redox systems and upregulation of enzymatic antioxidants, producing an at least partial recovery of GSH balance and an improvement of antioxidant capacity. Impressive results are discussed in this paper.
Aging
Aging processes are usually accompanied or even caused by mitochondrial dysfunction, the main source of ROS, and oxidative stress starting with superoxide radicals •O-O −– as described above.
As a consequence mtDNA (mitochondrial DNA) is oxidatively damaged, mutations occur, age-related diseases and others; finally, the excess of ROS leads to a change in cell response or a derangement of cellular metabolism. The antioxidant system is overloaded, antioxidant enzymes decrease, and we can measure a GSH deficit.
Here, medical ozone as redox bioregulator offers an ideal concept in a treatment of an aging process and the prevention of age related diseases.
Quite recently, this hypothesis has been proven correct in a first clinical study involving elderly patients (Fernández et al. Citation2022) and several years ago in a preclinical trial with animals (Safwat et al. Citation2014; Shehata et al. Citation2012).
Both of them are discussed here with a focus on glutathione balance.
Clinical study in aging
A randomized, controlled trial in elderly (n = 45), 60 to 70 years old patients with age-related diseases, n = 30, RA (rheumatoid arthritis) patients with comorbidities: diabetes and hypertension >5 years n = 15 (a second group, n = 15, with asthma as comorbidity >6 months, here not shown).
Basic medication: Metotrexate MTX, Ibuprofen, Folic acid.
Group I: “Control-group” basic medication only, n = 15
Group II: “ozone group” basic medication + ozone, n = 15
Systemic ozone application followed the low-dose ozone concept: 20 rectal insufflations over 4 weeks with concentrations of 20 up to 30
g/mL with an increment of 5
g/mL per week and a volume of 100–200 mL.
To reiterate the centrality of the GSH/GSSG redox balance in the application of ozone as a bioregulator, the biochemical results are reduced here to oxidative stress parameters and GSH, see : Base line: all groups at the beginning before treatment (p < 0,05).
Figure 5. (a) GSH as antioxidant defense marker and oxidative stress, (b) MDA and (c) TH) in a randomized clinical trial in elderly patients (60–70 years) with age related comorbidities, n = 30, with (ozone group) and without (baseline) ozone treatment. Control group with basic treatment only in comparison with base line. Ozone group, n = 15, before (base line) and after systemic ozone treatment. Control group after 4 weeks with basic treatment only. The measurement results (liver) indicate that the aging process in the ozone group is obviously decelerated and progresses in the control group (p < 0,05). TH: total hydroperoxides, MDA: malondialdehyde, GSH: reduced glutathione (Fernández et al. Citation2022).

Oxidative stress, here measured as MDA (malondialdehyde) and TH (total hydroperoxides, see above): compared with the base line MDA did not change, which means the process is mitigated or even stopped in the ozone-group, whereas the control group (basis medication only) shows an increase of oxidative stress parameters, both MDA and TH (as well as others, not shown here).
GSH displays the corresponding change: compared with the base line, there is a small decline, although we find a remarkable decrease in the control group.
Interestingly, within only 4 weeks, the aging process visibly progresses; we can see an increase in oxidative stress and a decrease in GSH; while in the ozone group the aging process is obviously attenuated. GSH is almost unchanged compared with the base line; so does the oxidative stress parameter MDA and TH decreases. The redox balance seems to be on its way to recovery.
The redox parameters were determined by spectrometric methods. SOD activity using kits by Randox Laboratories, Ireland, CAT through decomposition of H2O2 acc. to Boehringer, Mannheim. GSH determination followed the method of Sedlak and Lindsay (1968a).
Statistical analysis: OUTLIERS preliminary test followed by analysis of variance (ANOVA), by a homogeneity variance test (Bartlett – Box). In addition, a multiple comparison test was used (Student – Newman–Keuls test). Student’s t-test for independent samples, canonical discriminate analysis, and t-test of paired samples were applied. The results are presented as means ± standard error of the mean (S.E.M). The level of statistical significance used was at least p < 0.05.
For further information see the original publication (Fernández et al. Citation2022).
Preclinical trials in aging. Prevention
The most interesting question, of course, is whether aging processes throughout life can be delayed by systemic ozone administration (rectal insufflation 0,6 mg/kg rat, concentration 50 g/mL, ca 5 ml).
This could be demonstrated in a study on Wistar rats in 4 groups: The “age control” group consisted of 12 normally developed animals aged 15 months each; the animals in the “ozone group,” aged 3 months, received rectal ozone application from month 4 onward, at first 3 times per week, and then once per week from month 7 up to the age of 15 months. An oxygen group treated the same way as the ozone group, but with oxygen insufflation, served as another control group.
Therapy
Two additional groups -one ozone group and one oxygen group- with 12 animals per group grew up without any treatment. At 14 months of age, they were treated with 3 rectal ozone insufflations per week over a period of 4 weeks or with oxygen, respectively.
A group of adult animals (3 months) were used for the determination of the baseline (100%).
This double treatment strategy even allows us to compare the systemic ozone effect in both, prevention and in therapy.
From the considerable number of parameters describing the aging process, we here again selected glutathione GSH from the group of antioxidants together with malondialdehyde (MDA) as parameters for oxidative stress in the liver, kidney and blood or plasma (here not shown).
The effect of ozone can clearly be seen in . Preventive ozone administration keeps oxidative stress (MDA) low during aging processes and the antioxidants, here measured as GSH, at a considerably high level; all values are statistically verified at p < 0.05. The therapy on the other hand shows a much weaker effect; in GSH, we find an increase compared to age control (normal aging process) but 30% less than in the prevention study. The same tendency can be seen in oxidative stress: in the therapy group, MDA is much lower than in the control group but 70% above the baseline, whereas MDA in the prevention trial shows healthy values compared to the base line. The oxygen groups as control show a similar tendency, but to a much lesser extent (Shehata et al. Citation2012).
Figure 6. Systemic ozone application in an animal trial in aging. Comparison of preventive and therapeutic effects. Measurements in the liver. (a) GSH as antioxidative capacity. (b) MDA as oxidative stress parameter both in prevention and therapy. Age control: values of 14 months old, healthy animals after an untreated, normal aging process. Prevention. Oxygen control: oxygen by rectal insufflation 3× per week during 4 weeks. Ozone group: ozone/oxygen mixture 3× per week during 4 weeks from month 4 onwards to 14 months. Therapy. 14 months old animals, rectal ozone insufflation or oxygen (as control) 3×/per week during 4 weeks. Baseline: values of 3 months old animals (100%). Ozone concentration: 20 mg/L volume ca 5 ml, 0.6 mg/kg; 12 animals in each group (Safwat et al. Citation2014; Shehata et al. Citation2012).

As in a number of other studies e.g. on immune status, prevention with systemic ozone applications at low concentrations and dosages is a useful approach to prevent from age-related diseases.
Determination of redox parameters are described in (Shehata et al. Citation2012). Statistical analysis was performed with INSTAT II statistical software (Instat, USA). The minimal level of statistical significance p < 0.05 (ANOVA followed by Tukey – Kramer multiple comparison test.
Post COVID syndrome
Martinez-Sanchez (Citation2022) reviewed and evaluated all publications on ozone treatment of Covid patients in prevention and therapy, noting that about 220 patients have been treated with MAH, about 50 by rectal insufflation, and another 225 with ozonated saline. The pharmacological mechanisms accounting for the effectiveness against COVID-19 are summarized in Fernández et al. (Citation2021).
Since we do not interfere with an acute situation, but prefer chronic inflammation, we discuss here the post-Covid syndrome.
Tirelli et al. (Citation2021) report the results of a post-Covid non controlled trial with 100 patients in which fatigue syndrome was assessed as clinical outcome. 73% of the patients had a high benefit from treatment with respect to fatigue syndrome. No typical biochemical parameters were measured.
Therefore we refer here to a pilot study (Gil-Del-Valle et al. Citation2020), the first randomized clinical trial to include both the clinical outcome and the characteristic blood markers, all measured by spectrophotometric methods: Glutathione GSH (serum GSH by kinetic assay using GSH reductase, standard GSH from Sigma St. Louis, USA), MDA malondialdehyde (LPO-586 kit from Calbiochem, USA), SOD superoxide dismutase activity (modified pyrogallol autoxidation method), CAT catalase activity (Clairbone method), AOOP advanced oxidation protein products (Wiko-Worsat method). The statistical analysis was performed using SPSS software (Version 22, SPSS Inc., Chicago, IL, USA); Kolmogorov- Smirnov test. P value less than 0.05 was considered statistically significant. Detailed information can be found in the original publication (Gil-Del-Valle et al. Citation2020).
The number of patients was limited to 32:
Post COVID conditions (comparable to the post-vaccine syndrome) with long lasting high oxidative stress, low antioxidant capacity, proinflammatory cytokines in excess, and as a consequence, an imbalanced glutathione equilibrium is a typical indication for the low-dose ozone application. The pharmacological mechanisms accounting for the effectiveness against COVID-19 are summarized in León Fernández et al. (Citation2021).
The convalescence phase is shortened, the clinical symptoms are significantly improved, according to an open label, controlled clinical study (Gil-Del-Valle et al. Citation2020).
Here, we again focus on the highly sensitive parameter GSH to emphasize the redox-regulating effect of ozone.
Clinical study
Study design
About 32 patients, already 3 weeks virus negative, PCR negative (polymerase chain reaction) are to remain hospitalized for a further 4 weeks and receive: group I (n = 16), a standardized vitamin treatment and group II, the ozone group, (n = 16) receive an additional ozone treatment in the form of rectal insufflation. Treatment frequency: 2× per day with 12 hours in between for five days per week, in a schedule comprising 40 treatments. The concentrations started with 20 g/mL during the first week, with an increment of 5
g/mL per week up to 35
g/mL in week 4, with the volume of the insufflated ozone/oxygen mixture being increased from 150 mL over 3 weeks (corresponding to 3,000
g O3 per treatment) up to 200 mL over the last 5 days (7,000
g O3).
Results
Compared to the healthy control group (n = 20), all post COVID patients show dramatically decreased glutathione GSH and thus decreased antioxidant capacity. Accordingly, these patients are susceptible to infections (viruses, bacteria) and other comorbidities.
Four weeks of intensive treatment with a standardized vitamin complex bring only minor improvements, while in the ozone group (vitamin + ozone), a 50% increase in the active form of glutathione GSH leads to a substantial improvement in antioxidant capacity. An overall improvement (here not shown) clinical parameters included, show that 40% of patients benefit from vitamin administration and 90% from the combination vitamin complex plus ozone application, which is correspondingly reflected in the increase in GSH and decrease of MDA as oxidative stress parameter p < 0.05 ().
Figure 7. (a) GSH in post-Covid patients compared to a healthy control group. GSH in mol/g Hb. Vitamin group: n=16: 40 standardized vitamin treatments within 4 weeks, 2x per day. Ozone group: n=16; 40 vitamin + ozone treatments via rectal insufflation with increasing ozone concentrations week by week. 20 μg/mL / 25 μg/mL / 30 μg/mL / 35 μg/mL and a volume of 150 mL within 3 weeks and 200 mL in week 4. GSH was drastically reduced in all the post-Covid patients, very low increase in the vitamin group, 50 % increase in the ozone group, statistically significant with p < 0.05. (b) MDA as oxidative stress parameter decreases accordingly with p<0.05 (Gil-del-Valle et al. Citation2020).

Biotransformation – detoxification
A characteristic feature of sulfur makes its compounds an effective, indispensable regulatory and detoxification mediator in medicine and biochemistry. Sulfur reacts in a very wide variety of ways due to its possibility of also involving its 3d orbitals: remarkable for redox regulation is its ability to react radically and ionically and thus to scavenge all ROS in addition to free radicals making GSH in particular become one of the most important cellular antioxidant.
For an effective detoxification (biotransformation) to transform lipophilic toxins, antibiotics, xenobiotics and others into hydrophilic substances, making them available for elimination via the kidney and the intestinal tract, the liver requires a high level of reduced Glutathione GSH and an intact GSH/GSSG balance far on the side of GSH; in healthy cases 90% :10%, compare the 3 detoxification phases (Löffler Citation2014) in .
Figure 8. The phases of detoxification – biotransformation and the influence of ozone (based on Löffler Citation2014); modified and extended to Nrf2 and GSH.

Liver intoxication
How and to which extent systemic ozone supports this effect can be demonstrated by a classical liver intoxication with carbon tetrachloride CCl4 in an animal model () (Candelario-Jalil et al. Citation2001).
Figure 9. Prevention from liver intoxication by systemic ozone pretreatment. CCl4 intoxication in an animal model (each group n = 10) is completely controlled by 15 preventive ozone insufflations 1 mg O3/kg; (p < 0.05). MTX intoxication in patients with rheumatoid arthritis (n = 60) under methotrexate treatment. 20 rectal insufflations 4 weeks, O3: 25–40 g/mL; increment 5
g/mL per week, vol 200 mL, p < 0.05 (Candelario-Jalil et al. Citation2001; León et al. Citation2016; Oru et al. Citation2017).

CCl4 toxicity is based on CCl3-radical and CCl3OO-radical production stressing antioxidants, such as GSH, SOD and G-6PDH, which can be completely avoided by ozone pretreatment but not with oxygen: oxygen itself is a radical!
Ozone upregulates the antioxidants via nuclear factors (phase II of biotransformation/detoxification), GSH increases and free radicals or oxidants, such CCl3, CCl3 OO or other ROS can be scavenged, and the liver is protected.
In a similar way, liver intoxication was significantly reduced in patients with rheumatoid arthritis on MTX medication (Aslaner et al. Citation2015; León et al. Citation2016; Oru et al. Citation2017), shown by the GSH increase in biochemical and statistical analysis were performed as described above in the clinical trial with elderly patients. For details, please refer to the original paper (Candelario-Jalil et al. Citation2001; León et al. Citation2016).
A very similar mechanism is discussed for the biotransformation of chemotherapeutics, antibiotics or xenobiotics, and here, we find the basic mechanism and the rationale of a complementary ozone concept in chronic diseases, such as complementary oncology, chronic inflammatory conditions with the aim of liver protection from liver toxic medications.
Conclusion
Over the decades, systemic ozone therapy has completely changed: from empirical and experimental medicine to a clear, validated and evidence-based treatment concept, to well-defined indications and forms of application.
With a better knowledge of the pharmacology and biochemistry of medical ozone, the low-dose ozone concept has been developed as the result of research groups on a world-wide basis.
The prominent mechanism of medical ozone in its classical indications has emerged as redox bioregulation, which starts by the interference of the “ozone peroxide” with the most important, cellular antioxidant balance, the glutathione system, and after several regulatory processes and mechanisms, finally leads to the regulation of glutathione balance
GSH ⇋ GSSG (reduced ⇋ oxidized form).
This knowledge is a result of a large number of preclinical and clinical studies, illustrated here by two recent pilot clinical trials in elderly and post-Covid patients. Protection of the liver by ozone can be demonstrated by the same mechanism with statistically significant increase in GSH, as shown here as protection against CCl4 in an animal model and against MTX in a clinical study in RA patients.
But we are still working of a better reputation and an acceptance of the use of medical ozone in teaching medicine. Might it be helpful to change or extend the name to Redox Bioregulatory Therapy following the low-dose ozone concept?
Author contributions
All authors listed have made a substantial, direct, and intellectual contribution to the manuscript and approved it for publication.
Disclosure statement
The authors declare that the research was conducted in the absence of any commercial or financial relationships that could be construed as a potential conflict of interest.
Notes
* Janus, the two-faced Roman god
References
- Ajamieh, H. H., S. Menéndez, G. Martínez-Sánchez, E. Candelario-Jalil, L. Re, A. Giuliani, and Olga Sonia León Fernández. 2004. “Effects of Ozone Oxidative Preconditioning on Nitric Oxide Generation and Cellular Redox Balance in a Rat Model of Hepatic Ischaemia–Reperfusion.” Liver International 24 (1): 55–62. https://doi.org/10.1111/j.1478-3231.2004.00885.x.
- Aslaner, Arif, Tugrul Çakır, Betül Çelik, Ugur Doğan, Cebrail Akyüz, Ahmet Baştürk, Cemal Polat, Umut Gündüz, Burhan Mayir, and Ahmet Özer Şehirli. 2015. “The Protective Effect of Intraperitoneal Medical Ozone Preconditioning and Treatment on Hepatotoxicity Induced by Methotrexate.” International Journal of Clinical and Experimental Medicine 8 (8): 13303–9. PMID: 26550257. PMCID: PMC4612942.
- Bocci, V., E. Luzzi, F. Coradeschi, L. Paulesu, R. Rossi, E. Cardaioli, and P. Di Simplicio. 1993. “Studies on the Biological Effects of Ozone: 4. Cytokine Production and Glutathione Levels in Human Erythrocytes.” J Bio Reu Homeost Agents 7 (4): 133–138. PMID: 8023701.
- Candelario-Jalil, S., M. Al-Dalain, O. S. Fernández, S. Menéndez, G. Pérez-Davison, N. Merino, S. Sam, and H. H. Ajamieh. 2001. “Oxidative Preconditioning Affords Protection Against Carbon Tetrachloride-Induced Glycogen Depletion and Oxidative Stress in Rats.” Journal of Applied Toxicology: JAT 21 (4): 297–301. https://doi.org/10.1002/jat.758.
- Chow, Ching K. 1984. “Glutathione Peroxidase System of Ozone-Tolerant and Non-Tolerant Rats.” In Oxygen Radicals in Chemistry and Biology, edited by W. M. Saran Bors and D. Tait, 707–712. New York: Walter de Gruyter Berlin.
- Corteselli, Elizabeth M., Eugine Gibbs-Flournoy, Steven O. Simmons, Philip Bromberg, Avram Gold, and James M. Samet. 2019. “Long Chain Lipid Hydroperoxides Increase the Glutathione Redox Potential Through Glutathione Peroxidase 4.” Biochimica et biophysica acta 1863 (5): 950–959. https://doi.org/10.1016/j.bbagen.2019.03.002.
- Díaz-Soto, Marie Teresa, Angela Fraga Pérez, Jaqueline Dranguet Vaillant, A. Mallok, Renate Viebahn-Haensler, Silvia Menéndez Cepero, and Olga Sonia León Fernández. 2012. “Ozone Therapy Ameliorates Nervous System Disorders and Oxidative Stress in Patients During Ethanol Withdrawal—A Pilot Study.” Ozone: Science & Engineering 34 (6): 432–437. https://doi.org/10.1080/01919512.2012.717858.
- Fernández, León, S. Olga, Gabriel Takon Oru, Renate Viebhan Hansler, Gilberto López Cabreja, Irainis Serrano Espinosa, Juan Carlos Polo Vega, and Elizabeth García Fernández. 2021. “Medical Ozone: The Pharmacological Mechanisms Accounting for Its Effectiveness Against COVID-19/SARS-COV-2.” Journal of Medical - Clinical Research & Reviews 5 (3): 1–10. https://doi.org/10.33425/2639-944X.1199.
- Fernández, León, Olga Sonia, Gabriel Takon Oru, Renate Viebahn-Hänsler, Gilberto Lopez Cabreja, Irainis Serrano Espinosa, and Elizabeth García Fernández. 2022. “Medical Ozone Arrests Oxidative Damage Progression and Regulates Vasoactive Mediator Levels in Older Patients (60-70 Years) with Oxidative Etiology Diseases.” Front. Physiol. 3 November 2022. https://www.frontiersin.org/articles/10.3389/fphys.2022.1029805/full.
- Galiè, Mirco, Viviana Covi, Gabriele Tabaracci, and Manuela Malatesta. 2019. “The Role of Nrf2 in the Antioxidant Cellular Response to Medical Ozone Exposure.” International Journal of Molecular Sciences 20 (16): 4009. https://doi.org/10.3390/ijms20164009.
- Gil-Del-Valle, Lizette, Olga Elena López-Fernández, Joniel Arnoldo Sánchez-Márquez, Zullyt Zamora-Rodríguez, Ana Librada Carballo-Reyes, Lidia Asela Fernández-García, Mario Manuel Delgado-Guerra, et al. 2020. “Amelioration of Symptoms and Oxidative Stress in Hospitalized Convalescent Post SARS-COV-2 Patients Treated with Rectal Ozone Therapy and Nutritional Supplementation.” IJMPR 4 (6): 94–107. https://ijmpronline.com/admin/assets/article_issue/1609153795.pdf.
- Hoffmann, A., and R. Viebahn, 2001. ”The Influence of Ozone on 2,3-Diphosphoglycerate Synthesis in Red Blood Cell Concentrates.” Proceedings of the 15th Ozone World Congress, Imperial College London.
- Hoffmann, A., and R. Viebahn. 2002. “ Über den Einfluss von Ozon auf die 2,3 Diphosphoglyzerat-Synthese in Erythrozyten-Konzentraten [${\bf{\it{ }}}[$Influence of Ozone on 2,3-Diphosphoglycerate Synthesis in Red Blood Cell Concentrates] in Ozon-Handbuch. Grundlagen-Prävention-Therapie. [$[$Ozone-Handbook, Basics-Prevention-Therapy] edited by Viebahn-Hänsler and Knoch. ecomed, Landsberg, 1995–2006.
- Lell, Bertrand, Renate Viebahn, and Peter G. Kremsner. 2001. “The activity of ozone against plasmodium falciparum.” Ozone: Science & Engineering 23 (1): 89–93. https://doi.org/10.1080/01919510108961991.
- Léon Fernande Olga, S., Hussam H. Ajamijeh, Jorge Berlanga, Silvia Menendez, Renate Viebahn-Hänsler, Re Lamberto, and Ana M. Carmona. 2007. “Ozone Oxidative Preconditioning is Mediated by A1 Adenosine Receptors in a Rat Model of Liver Ischemia/Reperfusion.” Transplant International 21:39–48. https://doi.org/10.1111/j.1432-2277.2007.00568.x.
- Léon, O. S., S. Menendéz, N. Merino, R. Castillo, S. Sam, L. Pérez, E. Cruz, and V. Bocci. 1998. “Ozone Oxidative Preconditioning: A Protection Against Cellular Damage by Free Radicals.” Mediators of Inflammation 7 (4): 289–94. https://doi.org/10.1080/09629359890983.
- León, Olga Sonia, Renate Viebahn-Haensler, Gilberto López Cabreja, Erainis Serrano Espinosa, Yanet Hernández Matos, Liván Delgado Roche, Beatriz Tamargo Santos, Gabriel Takon Oru, and Juan Carlos Polo Vega. 2016. “Medical Ozone Increases Methotrexate Clinical Response and Improves Cellular Redox Balance in Patients with Rheumatoid Arthritis.” European Journal of Pharmacology 789:313–18. https://doi.org/10.1016/j.ejphar.2016.07.031.
- Löffler, Petridis. 2014. Biochemie und Pathobiochemie. [Biochemistry and Pathobiochemistry]. Berlin, Heidelberg: Springer Verlag.
- Martinez-Sanchez, Gregorio. 2022. ““Ozone Therapy for Prevention and Treatment of COVID-19“.” J Explor Res Pharmacol 7 (3): 189–194. https://doi.org/10.14218/JERP.2022.00015.
- Mizutani, Hideki, Tada-Oikawa Saeko, Yusuke Hiraku, Michio Kojima, and Shosuke Kawanishi. 2005. “Mechanism of Apoptosis Induced by Doxorubicin Through the Generation of Hydrogen Peroxide.” Life Sciences 76 (13): 1439–53. https://doi.org/10.1016/j.lfs.2004.05.040.
- Oru, Takon, Renate Viebahn-Haensler Gabriel, Gilberto López Cabreja, Irainis Serrano, Beatriz Tamargo Santos Espinosa, Juan Carlos Polo Vega, Susana Sánchez Cintas, and Olga Sonia León Fernandez. 2017. “Medical Ozone Reduces the Risk of γ-Glutamyl Transferase and Alkaline Phosphatase Abnormalities and Oxidative Stress in Rheumatoid Arthritis Patients Treated with Methotrexate.” SM Journal of Arthritis Research 1 (1): 1–7. https://doi.org/10.36876/smjar.1004.
- Re, Lamberto Gregorio Martínez-Sánchez, Marica Bordicchia, Giuseppe Malcangi, Antonella Pocognoli, Miguel Angel Morales-Segura, John Rothchild, Armando Rojas, and A. Rojas. 2014. “Is Ozone Pre-Conditioning Effect Linked to Nrf2/EpRe Activation Pathway in Vivo? A Preliminary Result.” European Journal of Pharmacology 742:158–162. https://doi.org/10.1016/j.ejphar.2014.08.029.
- Rokitansky, O., A. Rokitansky, W. Trubel, R. Viebahn, and J. Washüttl. 1981. “Ozon-Sauerstoff Therapie bei arteriellen Durchblutungsstörungen.” [“Ozone Therapy in Peripheral Arterial Circulatory Disturbances”]. Proceedings 5th Ozone World Congress of the International Ozone Association IOA-Wasser Berlin 81, Berlin.
- Safwat, M. H., M. M. El Sawalhi, M. N. Mausouf, and A. A. Shaheen. 2014. “Ozone Ameliorates Age Related Oxidative Stress Changes in Rat Liver and Kidney: Effects of Pre- and Post-Aging Administration.” BIOCHEMISTRY (Moscow) 79 (5): 450–458. https://doi.org/10.1134/S0006297914050095.
- Sagai, Masaru, and Velio Bocci. 2011. “Mechanisms of Action Involved in Ozone Therapy: Is Healing Induced via a Mild Oxidative Stress?” Medical Gas Research 1 (1): 29. https://doi.org/10.1186/2045-9912-1-29.
- Shehata, Nagwa Ibrahim, Hanan Mohamed Abd-Elgawad, Mohamed Nabil Mawsouf, and Amira Abd-Elmonem Shaheen. 2012. “The Potential Role of Ozone in Ameliorating the Age-Related Biochemical Changes in Male Rat Cerebral Cortex.” Biogerontology 13 (6): 565–581. https://doi.org/10.1007/s10522-012-9400-9.
- Sies, Helmut. 1999. “Glutathione and Its Role in Cellular Functions.” Free Radical Biology & Medicine 27 (9–10): 916–921. https://doi.org/10.1016/s0891-5849(99)00177-x.
- Sies, Helmut. 2021. “Oxidative Eustress: On Constant Alert for Redox Homeostasis.” Redox Biology 41:101867. https://doi.org/10.1016/j.redox.2021.101867.
- Sies, H., and D. P. Jones. 2020. “Reactive Oxygen Species (ROS) as Pleiotropic Physiological Signalling Agents.” Nature Reviews Molecular Cell Biology 21 (7): 363–83. https://doi.org/10.1038/s41580-020-0230-3.
- Tirelli, U. M., L. Franzini, S. Valdenassi, R. Pisconti, C. Taibi, C. Torrisi, S. Pandolfi, and S. Chirumbolo. 2021. “Fatigue in Post-Acute Sequelae of SARS-Cov2 (PASC) Treated with Oxygen-Ozone Autohemotherapy-Preliminary Results on 100 Patients.” European Review for Medical and Pharmacological Sciences 25 (18): 5871–5876. https://doi.org/10.26355/eurrev_202109_26809.
- Togi, Sumihito, Misa Togi, Satoshi Nagashima, Yuichi Kitai, Ryuta Muromoto, Jun-ichi Kashiwakura, Toshiaki Miura, and Tadashi Matsuda. 2021. “Implication of NF-Κb Activation on Ozone-Induced HO-1 Activation.” BPB Reports 4 (2): 59–63. https://doi.org/10.1248/bpbreports.4.2_59.
- Viebahn-Haensler, R. 2005. “Systemische Ozonanwendung in der Komplementären Onkologie.“[”systemic Ozone Application in Complementary oncology“.” In Ozon-Handbuch, edited by Viebahn-Haensler and Knoch, 1995–2006. Landsberg: ecomed.
- Viebahn-Haensler, Renate, and Olga Sonia León Fernández. 2021. “Ozone in Medicine. The Low-Dose Ozone Concept and Its Basic Biochemical Mechanisms of Action in Chronic Inflammatory Diseases.” International Journal of Molecular Sciences 22 (15): 7890. https://doi.org/10.3390/ijms22157890.
- Viebahn-Haensler, Renate, Olga Sonia León Fernández, and Ziad Fahmy. 2012. “Ozone in Medicine: The Low-Dose Ozone Concept—Guidelines and Treatment Strategies.” Ozone: Science & Engineering 34 (6): 408–24. https://doi.org/10.1080/01919512.2012.717847.
- Viebahn, R., J. Washüttl, G. Wasser, and U. Gutzen. 1995. “Influence of Ozone on RBC Metabolism Referring a Pilot Study: Rectal Ozone Insufflation in Elderly”. In Ozon-Handbuch, edited by Viebahn-Haenslerand Knoch, 1995–2006. Landsberg: ecomed, handbook (Hanbuch).
- Washüttl, J., and R. Viebahn. 1986. ““Biochemische Aspekte der Ozon-Sauerstoff Theapie” [Biochemical Aspects of Oxygen Ozone Therapy].” Ars Medici 5:194–199.
- Weitzel, Buddeke, E. Buddecke, F. Schneider, and H. Pfeil. 1961. “Einwirkung organischer Persauerstoff-Verbindungen auf das Mäuse-Ascitescarcinom in vivo.” Hoppe-Seyler´s Zeitschrift für physiologische Chemie 325:65–90. https://doi.org/10.1515/bchm2.1961.325.1.65.
- Yang, Haotian, Rehan M. Villani, H. Haolu Wang, Matthew J. Simpson, Michael S. Roberts, Min Tang, and Xiaowen Liang. 2018. “The Role of Cellular Reactive Oxygen Species in Cancer Chemotherapy.” Journal of Experimental & Clinical Cancer Research: CR 37 (1): 266. https://doi.org/10.1186/s13046-018-0909-x.