ABSTRACT
Due to low degradability of plastic materials, high usage, and low cost, microplastics (MPs) is becoming ubiquitous environmental pollutant. Wastewater treatment plants (WWTP) are one of main sources, where most of the MPs from wastewaters ends in waste sludge. Anaerobic digestion is one of most promised techniques of waste sludge management. The aim of this paper was to evaluate the impact of polyethylene terephthalate (PET) and polypropylene (PP) microplastics in the waste sludge with and without ozonation as possible pretreatment, on biogas and methane production with OxiTop® method. In the first set of experiments, PET inhibited (up to 6% at 0.1 g L−1) or promoted (up to 3% at 1 g L−1) methane production but increased cumulative biogas production (up to 38% at 0.5 g L−1). PP inhibited methane production (up to 5% at 0.5 g L−1) but its impact on biogas production was dependent upon concentration of MPs added. In the second set of experiments, pretreatment of non-contaminated waste sludge by ozonation (3.54 gozone h−1) inhibited methane yield. Longer time of ozonation of PP-contaminated waste sludge reduced inhibition of methane yield, while in the case of PET-contaminated waste sludge, it was increased.
Introduction
Plastic pollution is increasing worldwide, since products, made of plastics have been produced in large quantities and widely used. Due to low degradability of plastic materials, its high usage, and low cost, plastic is becoming a ubiquitous environmental pollutant. Waste plastics are after being used, discarded in the environment, and could be found in water, soil, air, and all other ecosystems, where they are fragmented into smaller particles (Borrelle et al. Citation2020). Different types of size classifications were proposed. Particles sized from 0.1 µm to 5 mm are usually classified as MPs and smaller than 0.1 µm as nanoplastics (NPs) (Blair, Waldron and Gauchotte-Lindsay Citation2019; Lv et al. Citation2019; Padervand et al. Citation2020; X. Zhang, Chen and Li Citation2020a). However, there is no unified definition yet, resulting in making extraction, identification, and quantification of MPs limited giving non-comparable data. MPs could enter environment through effluents of wastewater treatment plants (WWTPs) that receives domestic and industrial wastewater and rainwater (Eerkes-Medrano, Thompson and Aldridge Citation2015; Sun et al. Citation2019).
Treatment in the WWTP could reach up to 99.9% of MPs removal (Sun et al. Citation2019; Talvitie, Mikola, Koistinen, et al. Citation2017), but significant amount of MPs is still discharged by effluents due to large volume of wastewater being treated (Murphy et al. Citation2016). MPs removal efficiency depends on the type of processes used in the WWTP, and it is also related to the presence of different types and shapes of MPs (Wu et al. Citation2021). Primary treatment significantly contributed to the MPs removal and could reach up to 98% (Talvitie, Mikola, Setälä, et al. Citation2017; Yang et al. Citation2019). Secondary treatment does not contribute to the removal of MPs by degradation but mainly by adsorption, thus MPs removal in this stage of WWTP usually does not reach more than 20% (Murphy et al. Citation2016). Treatment systems with tertiary stage could reach up to 99.9% (Sun et al. Citation2019; Talvitie, Mikola, Setälä, et al. Citation2017). Most of the MPs are retained in sludge (Gatidou, Arvaniti and Stasinakis Citation2019; Xu et al. Citation2019; Ziajahromi et al. Citation2017). Researchers reported different concentrations of MPs in the waste sludge due to the different units used. Some reported about 305.34 ± 166.85 µg of particles (10500 µm) per g of dry weight (DW) and 264.32 ± 304.72 µg of particles (larger than 500 µm) per g DW (Rasmussen et al. Citation2021), other 507 particles per liter of activated sludge, 1180 particles per liter of excess sludge and even up to 58,000 particles per kg− of sludge cake (Nakao et al. Citation2021). The most commonly detected in WWTP are polyester (PES), polypropylene (PP), polyethylene terephthalate (PET) and polyamide (PA) (Sun et al. Citation2019). Amount of waste sludge, formed during wastewater treatment is increasing (Z. W. He et al. Citation2019). Since waste sludge is mainly composed of organic matter (Z. W. He et al. Citation2019), anaerobic digestion is one of most promised techniques of waste sludge treatment to reduce sludge volume, and its odor. At the same time waste sludge is stabilized and CH4 formed in biogas can be used to produce energy (Ma et al. Citation2018; X. Zhang, Chen and Li Citation2020b). However, process of anaerobic digestion can be hindered by some pollutants, such as antibiotics (Alenzi et al. Citation2021), heavy metals (Lee and Lee Citation2019), and other micro- and nanoparticles (Lee and Lee Citation2019; Wei, Zhang, et al. Citation2019). MPs have large specific surface and strong hydrophobicity; thus, it can potentially adsorb specific pollutants present in the wastewater (X. Li et al. Citation2018). To improve durability and flexibility of plastics, additives (PAs) like antioxidants, plasticizers, and flame-retardants are also added during manufacturing process (Hermabessiere et al. Citation2017). Many of them are toxic and could leach out from plastic particles and impact different processes in treatment plants as well as in the environment (Hermabessiere et al. Citation2017). Adsorption and desorption of compounds on MPs depend on type and size of polymers and their composition, aging of MPs and its exposure to environmental factors (Hatinoğlu and Sanin Citation2021; Mohsen et al. Citation2019; Noro and Yabuki Citation2021; Xiong et al. Citation2019).
Not many studies investigated possible impacts of MPs retained in the sludge on different processes, such as anaerobic digestion. Some studies reported that MPs may have certain impact on anaerobic digestion of sludge (Wei, Huang, Sun, Wang, et al. Citation2019), but their synergistic effects with other pollutants are still unknown. Some types of MPs, like PVC, could leach out some pollutants, like Bisphenol A (BPA) and thus inhibit anaerobic digestion (Wei, Huang, Sun, Wang, et al. Citation2019). Wei, Citation2019 (Wei, Huang, Sun, Wang, et al. Citation2019) discovered, that BPA leaching from PVC affected sludge solubilization and thus impacted on methane production. During solubilization process, particulate organic matter in waste activated sludge are destroyed and converted to soluble organic substances. It was discovered that release of BPA corresponded with increased levels of MPs. Li, Citation2023 (J. Li, Dagnew and Ray Citation2023) confirmed increase in methane production for up to 43% with microfibers abundance of 20, 100, and 1000 mg L−1 and suggested that effect of MPs on anaerobic digestion depends on concentration, chemical composition, and type of pre-treatment. Chen et al. (Citation2023) observed that lower added concentration of polycarbonate (PC) 10–60 particles gTS−1 improved methane production, while concentration of 200 PC particles gTS−1 inhibited methane production. By leaching BPA of PC, reactive oxygen species (ROS) production was affected by viability of biomass and activity of enzyme. Regulated ROS production affected anaerobic digestion and also microbial community. In other study, Chen et al. (Citation2021) discovered that all used concentrations (5–50 particles gTS−1) of polyamide enhanced methane production for up to 39.5% and reaching the highest at 10 particles gTS−1. It was also observed that caprolactam (CPL) leaching from PA6 binds with enzyme molecules and affecting the catalytic activity of the enzyme.
Wei et al. (Citation2021) discovered that entry route of MPs in the WWTP also effects aerobic digestion of waste sludge. Entry route of MPs impacts on solubilization of waste activated sludge and inhibition on hydrolysis. PET microplastics also reduced microbial community.
Ozonation seems to be a promising method for waste sludge processing leading to solubilization of cells make them accessible to anaerobic microorganisms (Dogruel et al. Citation2020; Schollée, Hollender and McArdell Citation2021). It changes not only properties of the sludge but also physicochemical properties of MPs polymers. Due to ozone’s ability to release intracellular material into the medium and to oxidize organic matter, biodegradability of the sludge is improved and potential of MPs degradation increased simultaneously (Hatinoğlu and Sanin Citation2021). J. Zhang et al. (Citation2016) discovered that ozonation of sludge caused disintegration of sludge and destruction of microbial cells. He also discovered that Cu and Zn had a higher direct toxicity after ozonation compared to un-ozonated sludge, while Shao et al. (Citation2020) studied impact on polyethylene (PE) oxidation. He discovered that ozonation affected specific surface area and also functional groups of the PE surface. Zhu et al. (Citation2022) compared raw and oxidized PET and after ozonation discovered impact on rougher surfaces, on releasing additives inside PET and also higher adsorption capacity onto PET.
The aim of this paper is to determine the impact of PET and PP as two of the most common types of MPs present in the waste sludge, on biogas and methane production in anaerobic digestion. Inhibition of various types of MPs on biogas and methane yield was evaluated, with and without ozonation as possible sludge treatment to improve biogas production. It was assumed that formed biogas consists only of CH4 and CO2, since other gases are present only in traces.
Materials and methods
Samples and contamination of waste sludge
Different types of MP (PP and PET) in various concentrations (0.110 g L−1), commonly identified in waste sludge, were used to evaluate methane yield and inhibition on biogas production with and without ozonation as pretreatment method. Plastic waste of PP and PET, the most common types of MPs identified in WWTPs were used (Horton et al. Citation2021; Okoffo et al. Citation2019) and cut by hand on smaller particles. PP, with density of 0.8550.946 g cm−3, was cut out of yogurt cup and PET, with density of 1.38 g cm−3, out of uncolored potable water bottle. After cutting, particles were sieved and those smaller than 1 mm were selected for experiments.
For the biogas production measurement experiments, aerobic and anaerobic sludge were collected at the municipal WWTP with combined sewage system, and secondary treatment (capacity of 360.000 PE). Daily-treated sewage volume is ≈82.200 m3 during dry season and ≈103.500 m3 during the rainy season (Doo Citation2019). Aerobic sludge was collected from aeration tank during aeration, to ensure agitation and its homogenous composition. Anaerobic sludge was taken from digester of WWTP. Anaerobic sludge was sampled according to ISO 11,734:1995 into an expandable bottle with a wide neck. Prior conduction of the experiments, aerobic sludge was purged with air at room temperature (22 ± 2 °C) for two days to eliminate remained organic compounds, while anaerobic sludge was conditioned at 37 ± 1°C in oxygen-free atmosphere. Sludge samples were filtered through black ribbon and dried to a constant mass at 105 ± 2 °C to determine concentration of total suspended solids (gTSS L−1). When measuring biogas production, 50 mL of aerobic sludge was added in the glass bottle, with average concentration of 3.2 ± 1.8 gTSS L−1. Concentration of the added anaerobic sludge was 1.5 ± 0.1 gTSS L−1.
To evaluate biogas production and methane yield, two different set of experiments were performed. First set of experiments was conducted without ozonation as waste sludge pretreatment method. Only biogas production of anaerobic sludge was measured with addition of different types of MPs (0.110 g L−1 of PP and PET) and compared to anaerobic sludge without MPs (as control sample) added. In the second set of experiments, ozonation of aerobic sludge as pretreatment was conducted and four different samples were compared: a) non-ozonated aerobic sludge, b) non-ozonated-contaminated aerobic sludge with known concentration and type of MP, c) ozonated non-contaminated aerobic sludge, and d) ozonated-contaminated aerobic sludge with known concentration and type of MP. 1 g L−1 of PET and PP was added in the sludge samples. Each experiment was run in duplicate and repeated twice.
Inhibition of biogas production
To determine the inhibition of MPs on anaerobic process in the first set of experiments, the production of biogas of anaerobic sludge with different added concentrations and types of MPs was monitored. The inhibition was determined by modified standard procedure (SIST EN ISO 11734 (Citation1995 2017)), OxiTop® method, where biogas production was measured in terms of increased pressure (hPa) in a closed system. Mixture of anaerobic sludge (maintaining fixed concentration of gTSS L−1), 0.5 mL of glucose as substrate (c = 0.833 mol L−1) and 2 mL of buffer solution for maintaining constant pH were filled up to 100 mL in glass bottles. PET and PP sized <1 mm were added (0.1 to 10 g L1).
In the second set of experiments, mixture of 50 mL aerobic sludge (non-ozonated; non-ozonated contaminated with known type and concentration of MPs; ozonated non-contaminated; ozonated contaminated with known type and concentration of MPs), 0.5 mL of glucose as substrate (c = 0.833 mol L−1), 2 mL of buffer solution for maintaining constant pH and anaerobic sludge (maintaining fixed concentration of 1.5 gTSS L−1) were filled up to 100 mL in glass bottles. PET and PP sized <1 mm were added (0.1 to 10 g L1).
In both experiments, control samples without added MPs were run to evaluate the impact of added MPs on biogas production. All experiments were run in duplicates (n = 2) and repeated twice. Biogas production (hPa) was measured in sealed 250 mL glass bottles at constant temperature 37 ± 1°C for 8 days. When biogas (composed mainly of CH4 and CO2) is produced, pressure increases. After adding 2 mL of 6.0 M NaOH (on the 7th day of experiment) through side rubber of the bottles, formed CO2 is absorbed. The remained pressure is due to the CH4 formation and with differences of pressures, percentage of both gases in the biogas were calculated. Standard deviations of biogas measurement results varied from ±0% to ±15.5%.
Ozonation of waste aerobic sludge
Known concentrations of MPs (0.110 g L−1), type PP and PET mixed in 100 mL of aerobic sludge were purged with ozone in 250-mL glass ozone batch bubble column reactor (). Wedeco H16 system was used. Operating pressure of ozone reactor was set to 0.5 bar and gas flow to 30 L h−1 at normal temperature and pressure (0 °C, 1 atm. Concentration of the ozone in the gaseous phase was 118 gozone Nm−3 (NTP), which resulted in ozone production of 3.54 g h−1 (0.059 g min−1). Samples for determination of biogas production potential were redrawn at 10, 20, and 30 min. All experiments were run in duplicates (n = 2) and repeated twice.
FTIR analysis of the samples
Particles of the MPS were prior and after experiments analyzed by Fourier transform infrared spectroscopy (FTIR) to examine possible impacts of ozonation and exposure to anaerobic microorganisms during biogas production experiments on composition and surface structure of plastic particles. After biogas production measurement experiments, MPs were collected from OxiTop bottles and air-dried for two days to constant mass. Infrared spectra of the samples were recorded using a Perkin Elmer Spectrum Two FTIR Spectrometer equipped with Spectrum Two Universal ATR (Single Reflection Diamond). Wavenumber was in the range from 4000 cm−1 to 400 cm−1 (resolution 2 cm−1, 10 scans).
Statistical analysis
The data presented in this study were expressed as the mean values (±SD, standard deviation) of replicates. The data presented on graphs were expressed as the mean values ±SE (standard error). The significance of the data was analyzed using unpaired t-test. Values p < 0.05 were considered statistically significant in this study.
Results
Biogas production
In average, 53.0%±2.4% of CH4 was formed during performed experiments. This is consistent with results of other studies. Lekše et al. (2022) reached 45 ± 9% of CH4 formed in their experiment, while Zhang and Wang (Citation2021) measured around 45% of CH4 in the biogas.
presents percentages (Vol.%) of CH4 and CO2 that were produced during experiments, where anaerobic sludge was contaminated with PET and PP without ozonation, including control sample. In general, both types of MPs particles (PET and PP) mainly decreased methane yield in comparison to control sample. The results showed that methane yield at 0.1 g L−1 of PET added significantly decreased methane yield by 6%, while 1 g L−1 significantly (p < 0.05) promoted methane yield in comparison with control sample and reached 56.0 ± 0.4%. Other concentrations of PET express no differences in comparison with the control (p > 0.05). PP significantly decreased methane yield at 0.5 g L−1 and reached 48.0 ± 1.6%, while other concentrations had no significant impact (p > 0.05).
Figure 2. Formed CH4 ± SE and CO2 in comparison to control sample, according to added concentration of a) PET and b) PP [%].* indicates statistically significant differences (p < 0.05) in comparison to control sample.
![Figure 2. Formed CH4 ± SE and CO2 in comparison to control sample, according to added concentration of a) PET and b) PP [%].* indicates statistically significant differences (p < 0.05) in comparison to control sample.](/cms/asset/e7a45ef7-0a26-4128-ae6c-599906d573a5/bose_a_2332286_f0002_b.gif)
Based on the results of experiments, it seems that PP and PET mainly decreased methane production (). Pittura in his research (Pittura et al. Citation2021) discovered that methane production was barely affected with concentration of 18 g TS −1, of PP, while 50 g TS −1 of PP severely decreased methanogenic activity and reached 4% 58% decrease in methane yield. Müller et al. (Citation2018) had concluded that PP has high sorption capacity for some compounds, such as ethyl benzene and xylene that could through leaching affect methane production. Y.-T. Zhang et al. (Citation2020) found that concentration from 75,300 particles of PET L−1 decreased methane yield by 17.2%28.4%.
As seen in , cumulative biogas production was in average higher at almost all added concentrations of PET, but it significantly increased at 0.5 g L−1 (p < 0.05), while no significant difference in comparison with control sample was noticed at other added concentrations. PP in concentration of 1.5 g L−1 significantly (p < 0.05) promoted biogas production compared to average cumulative biogas production in control sample (15%). Other applied concentrations of MPs mainly decreased cumulative biogas production, but not significantly. It was not possible to correlate concentrations of added PP and PET to biogas production, but large impact of both types of MPs on anaerobic digestion was confirmed in terms of biogas as also methane production. It can be caused by different harmful contaminants adsorbed on MPs particles including additives in the particles impacting microorganisms while digested during anaerobic processes. (Wei, Huang, Sun, Dai, et al. Citation2019; Wei, Huang, Sun, Wang, et al. Citation2019; Wei, Zhang, et al. Citation2019) discovered that exposure of PET to anaerobic microorganisms could lead to leaching of some toxic substances like di-n-butyl phthalate and could cause the generation of ROS that cause reduction of cell viability and inhibition of hydrolysis, acidification as also methanogenesis. It was also discovered that the presence of PET has significant effect on microbial activity, since PET reduced the population of fermentative bacteria and cause decrease in hydrogen production, that could be caused by previously mentioned DBP, leaching from PET or ROS, that is stimulated by PET (Y.-T. Zhang et al. Citation2020) and causing death of cells by high oxidative stress, followed by inhibition of methane production. PP has strong adsorption capacity to adsorb antibiotics and other contaminants. It was discovered that it could adsorb antibiotics (J. Li, Zhang and Zhang Citation2018), polycyclic aromatic hydrocarbons (PHAs), fuel (Müller et al. Citation2018) and heavy metals, such as Pb, Cu, Cd, Co (Gao et al. Citation2019; X. Li et al. Citation2019) that are common in wastewater and in waste sludge. When heavy metals reach certain concentration, they could become inhibitory. Adsorbed contaminants, as well as MPs have impact to anaerobic digestion (Wang et al. Citation2020).
Table 1. Cumulative biogas production [hPa] ± SE of PP- and PET-contaminated anaerobic sludge in concentrations 0.1 10 g L−1, compared to control sample. * indicates statistically significant differences (p < 0.05) in comparison with control sample.
The impact of ozonation on methane and biogas production of PP- and PET-contaminated sludge
The impact of ozonation on methane and biogas production of PP- and PET-contaminated sludge, with concentration 1 g L−1 of MPs were determined using 3.54 g h−1 dose of ozone. Ozonation times for 10, 20, and 30 min for both types of MPs were used. Methane and biogas production of four different samples (aerobic sludge, aerobic sludge contaminated with PP/PET, ozonated aerobic sludge and ozonated aerobic sludge contaminated with PP/PET) were compared.
As seen in , methane yield for sludge contaminated with PP and PET was significantly promoted when compared to control sample. PET promoted it up to 8%, while PP promoted it for up to 3%. 10 min of ozone pretreatment (3.54 g h−1 of ozone) seem to decrease methane yield (p > 0.05). After 10 min of ozonation, amount of ozone introduced reached 0.59 g equals to 5.9 gozone gMP−1. The results showed that 10 min ozonation of PP-contaminated ozonated sludge significantly decreased (p < 0.05) methane production, up to 45%. No significant impact was noticed with ozonation of PET-contaminated sludge, in comparison with control sample.
Figure 3. Methane yields ± SE (a) and biogas inhibition ± SE (b) of contaminated (1 g L−1) and ozonated sludge at dose 3.54 g h−1 for 10 min compared to control sample for [%]. * indicates statistically significant differences (p < 0.05) in comparison with control sample.
![Figure 3. Methane yields ± SE (a) and biogas inhibition ± SE (b) of contaminated (1 g L−1) and ozonated sludge at dose 3.54 g h−1 for 10 min compared to control sample for [%]. * indicates statistically significant differences (p < 0.05) in comparison with control sample.](/cms/asset/73ea179e-d10f-4200-8e9c-e5dae7a43011/bose_a_2332286_f0003_oc.jpg)
presents cumulative biogas production for experiments with 10 min ozonation of PP- and PET-contaminated aerobic sludge (c = 1 g L−1). It can be seen that the addition of PP significantly (p < 0.05) promoted biogas production (up to 12%), while with PET added, no significant difference was noticed. On the other hand, ozonation of non-contaminated sludge decreased biogas production up to 35%, while ozonation of contaminated sludge decreased it even up to 61% (PP), Ozonation of PETcontaminated sludge also significantly decreased biogas production up to 14% in comparison with control sample.
As seen in , no significant methane yield for PP- or PET-contaminated sludge was noticed. 20 min of ozonation of sludge, with 3.54 g h−1 dose of ozone, significantly decreased methane yield. After 20 min of ozonation, amount of ozone introduced in the system reached 1.18 g leading to 11.8 gozone gMP−1. Methane yield significantly decreased up to 18% when PP-contaminated sludge was ozonated. Compared to control, methane yield also significantly decreased for 6% with 20 min of ozonation of PET-contaminated sludge.
Figure 4. Methane yields ± SE (a) and biogas inhibition ± SE (b) of contaminated (1 g L−1) and ozonated sludge at dose 3.54 g h−1 for 20 min, compared to control sample [%]. * indicates statistically significant differences (p < 0.05) in comparison with control sample.
![Figure 4. Methane yields ± SE (a) and biogas inhibition ± SE (b) of contaminated (1 g L−1) and ozonated sludge at dose 3.54 g h−1 for 20 min, compared to control sample [%]. * indicates statistically significant differences (p < 0.05) in comparison with control sample.](/cms/asset/c7da4a0f-abb8-4627-b6f8-7b7fb3b00703/bose_a_2332286_f0004_oc.jpg)
presents cumulative biogas production for experiment with 20 min ozonation of PP- and PET-contaminated aerobic sludge (c = 1 g L-1). It can be seen that addition of PET and PP did not affect the biogas production. Ozonation alone significantly decreased cumulative biogas production up to 39%, the same was also noticed when PET- and PP-contaminated sludge were ozonized. Biogas was reduced for 17% and up to 19% for PP and PET, respectively.
As seen in , methane yield for PP- and PET-contaminated sludge, was promoted in comparison with control sample. PET significantly promoted methane yield up to 8% and PP up to 3%. After 30 min of ozonation, amount of ozone reached 17.7 gozone gMP−1. Applied dose of ozone (3.54 g h−1) significantly decreased methane yield up to 18% and up to 11% for PP and PET, respectively.
Figure 5. Methane yields ± SE (a) and biogas inhibition ± SE (b) of contaminated (1 g L−1) and ozonated sludge at dose 3.54 g h−1 for 30 min, in comparison with control sample [%]. * indicates statistically significant differences (p < 0.05) in comparison with control sample.
![Figure 5. Methane yields ± SE (a) and biogas inhibition ± SE (b) of contaminated (1 g L−1) and ozonated sludge at dose 3.54 g h−1 for 30 min, in comparison with control sample [%]. * indicates statistically significant differences (p < 0.05) in comparison with control sample.](/cms/asset/ee481b24-7d8c-4711-a317-eff43e8163e2/bose_a_2332286_f0005_oc.jpg)
presents cumulative biogas production for experiment with 30 min ozonation of PP and PET-contaminated aerobic sludge (c = 1 g L−1). It can be seen, that added PP significantly promoted biogas production (up to 12%), while PET did not impact biogas production. Ozonation of the sludge without MPs significantly decreased it up to 33%. Ozonation of the contaminated sludge significantly decreased biogas production up to 41% (PP), while in the case of PET-contaminated sludge it decreased up to 24% in comparison with control sample.
Time of ozonation and type of MPs seems to affect methane yield and biogas production as seen in (PP-contaminated sludge) and (PET-contaminated sludge). 10 min of ozonation decreased methane yield and 20 min of ozonation increased it, results are comparable with the results of ozonation experiments with PVC-contaminated sludge of Lekše et al., 2022. Longer time of ozonation (30 min) seems to reduce inhibition of biogas production and increase methane yield and could be assumed, that longer time of ozonation increase the ratio of gozone gMP−1 and thus increase oxidation capacity of the introduced ozone. Ozone degraded or at least deactivated out of the MPs leached compounds and lead to lower inhibition of the anaerobic microorganisms.
Figure 6. Methane yield ± SE (a) and biogas inhibition ± SE (b) of PP-contaminated and ozonated sludge at 10, 20, and 30 minutes of ozonation. * indicates statistically significant differences (p < 0.05) in comparison with control sample.
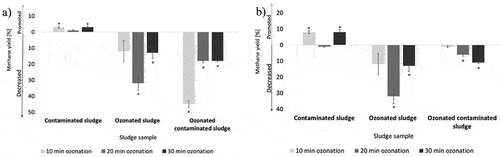
Ozonation of PP-contaminated sludge seems to cause the highest decrease of biogas production after 10 min of ozonation, while longer ozonation (20 or 30 min) reduced the inhibition of biogas production (). Methane production of PET-contaminated sludge seems to be correlated with time of ozonation, where longer time of ozonation decreased methane yield. It could be assumed, that with time, more in PET MPs contained compounds are released from MPs and impact methane production. Based on results of ozonation of PP-contaminated sludge, it could be concluded that at shorter time (t = 10 min) of ozonation compounds, adsorbed on MP, started leaching from MPs and starting to inhibit methane production. It could also be assumed, that longer time of ozonation (t = 2030 min) increased leaching and amount of compounds that are reducing methane production. Increased concentration of these compounds in the solution even decreased inhibition, compared with shorter time of ozonation. Ozonation of MPs-contaminated sludge in some cases decreased methane inhibition in comparison with ozonated sludge.
FTIR spectra
MPs particles (PP and PET) used in experiment were identified using FTIR analysis. Non-treated particles, particles after biogas production as well as ozonated particles after biogas production were investigated (). Particles were collected after biogas production measurements at concentration of 10 g L−1 and ozonated particles were sampled after 30 min of ozonation at 3.54 g h−1 dose of ozone. As seen in spectra in Figure below, it seems that anaerobic digestion and ozonation followed by anaerobic digestion did not affect composition of PP and PET particles. Rashid, Tsuru and Ishikawa (Citation2015) ozonated PET for 6 h at 37°C, using gas pressure of 0.15 MPa, gas flow 2 L min−1 and electric current 2 A in distilled water. By O3 oxidation, different functional groups, including carboxyl group (OH), were formed on PET surface. G.-J. He et al. (Citation2015) used 10 mg L−1 ozone concentration and flow rate 5 L min−1 to ozonation of PP by higher temperatures (200, 220, and 240°C). Comparing to untreated PP, new band at around 1720 cm−1 was formed, which corresponds to C=O bond and 841 cm −1, corresponding to CH3 group. Their presence might suggest the presence of carboxylic acid, ester and aldehyde or ketone functional groups.
Discussion
PP and PET in general decrease methane production or has no obvious difference. Higher addition of PET generally promoted biogas production and decreased methane yield at 0.1 g L−1 and increased it at 1 g L−1. PP inhibited methane yield at 0.5 g L−1 and had no obvious difference at other concentrations of MPs, while correlation between concentration of MPs and biogas production is not straightforward. Some researchers discovered toxic effects of MPs leachates on different organisms. H. X. Li et al. (Citation2016) reported leachates from PET and PP increased the mortality of certain microorganisms and thus change the microbial community. Wei, Zhang, et al. (Citation2019) also discovered that addition of 60 particles g−1 of PET impacted on microbial community and decreased the abundances of hydrogen producers (Bacteroides sp., Tissierella sp. and Fonticella sp.), while hydrogen consumers (Methanosaeta sp., Methanobacterium sp. and Acetoanaerobium sp.) were barely observed. Change of certain type of microorganisms (anaerobic microorganisms) could lead to decreased production of methane or biogas.
Ozonation, used as possible pretreatment method for waste sludge prior to anaerobic digestion, seems to decrease methane yield and cumulative biogas production. Results of experiment are consistent with results from study of Lekše et al, 2022, where impact of ozonation as pretreatment of PVC-contaminated waste aerobic sludge was determined. In the study was shown that 20 min ozonation time increased biogas inhibition of contaminated as well as non-contaminated sludge, using 3.54 g h−1 dose of ozone, while using higher dose of ozone-promoted biogas production. Various processes can change MPs, including ozonation. According to J. Zhang et al. (Citation2016) discovery, ozonation could impact on physicochemical characteristics of sludge and higher direct toxicity of heavy metals and bioavailability, possibly affecting on microbial activity and biogas production. Microbial activity could also be affected by enhanced releasing of additives from MPs after ozonation (Zhu et al. Citation2022).
MPs could contain certain compounds, heavy metals, antibiotics etc., that could affect microorganism’s activity. It could be assumed that 3.54 g h−1 dose of ozone and concentration of added MP (1 g L−1) used it this paper was too low to cause leaching of compounds to promote methane yield. J. Li, Zhang and Zhang (Citation2018) also discovered that PP had very strong adsorption capacity for antibiotics which can later impact anaerobic digestion process. It is also important to note that MPs have strong adsorption potential for heavy metals and it has been discovered that moderate level of heavy metals is able to promote methane production, while excessive level of heavy metals could lead to inhibition of methane production (Jagadabhi et al. Citation2019).
Conclusion
Main findings of the conducted research were as follows:
PP MPs inhibit methane production during anaerobic digestion (0.11.0 g L−1), while concentrations of 1.510 g L−1 seem to not have significant impact and promote biogas production (0.110 g L−1, except 1.5 g L−1).
PET MPs inhibits methane production during anaerobic digestion at 0.1, 0.5, and 10 g L−1. 1 g L−1 of PET promotes methane yield, while at 1.5 g L−1, no significant impact was noticed.
Ozonation PP- and PET-contaminated waste sludge could inhibit methane yield. Decrease depends on time of the ozonation. With increased time of ozonation PP-contaminated sludge methane yield was decreased, while methane yield of PET-contaminated sludge correlated with time of ozonation and reached the highest decrease at 30 min of ozonation (up to 11%).
Ozonation of PP-contaminated sludge decreased methane production, compared to ozonation of non-contaminated sludge (except at 20 min). It can be assumed that ozonation of PP-contaminated sludge caused leaching of compounds, that impacted on microbial community and thus affected methane production.
Ozonation of PET-contaminated sludge improved methane production, compared to non-contaminated sludge. According to Wei, Citation2019 ((Wei, Zhang, et al. Citation2019)), we could assume, that concentration of added PET was too low to cause impact on microbial community.
FTIR analysis confirmed that composition of PP and PET MPs was not affected during ozonation nor anaerobic digestion.
Additional experiments should be accomplished to confirm our preliminary findings. More doses of ozone should be investigated as well as different concentrations of MPs added in waste sludge. It was concluded that MPs have significant impact on biogas production and methane yield. Many types, sizes, and shapes of polymers need to be investigated. The special attention has to be paid to possible compounds adsorbed to MPs that could leach and affect methane/biogas production to completely understood effects of MPs on anaerobic digestion.
Supplemental Material
Download JPEG Image (295.8 KB)Supplemental Material
Download JPEG Image (295.2 KB)Supplemental Material
Download MS Word (200 KB)Supplemental Material
Download (45 KB)Acknowledgments
The authors are very grateful to Nina Kukovičič and Taja Černic for their laboratory assistance. Contribution and assistance of dr. Ula Rozman and assist. prof. dr. Marija Zupančič from Faculty of Chemistry and Chemical Technology, University of Ljubljana, are greatly acknowledged as well as the help with statistics of Dr. Igor Boševski.
Disclosure statement
No potential conflict of interest was reported by the author(s).
Data availability statement
The data that support the findings of this study are available from the corresponding author, upon reasonable request.
Supplementary material
Supplemental data for this article can be accessed online at https://doi.org/10.1080/01919512.2024.2332286.
Additional information
Funding
References
- Alenzi, A., C. Hunter, J. Spencer, J. Roberts, J. Craft, O. Pahl, A. Escudero, et al. 2021. “Pharmaceuticals Effect and Removal, at Environmentally Relevant Concentrations, from Sewage Sludge During Anaerobic Digestion.” Bioresource Technology 319:124102–124102. https://doi.org/10.1016/j.biortech.2020.124102.
- Blair, R. M., S. Waldron, and C. Gauchotte-Lindsay. 2019. “Average Daily Flow of Microplastics Through a Tertiary Wastewater Treatment Plant Over a ten-Month Period.” Water Research 163:114909. https://doi.org/10.1016/j.watres.2019.114909.
- Borrelle, S. B., J. Ringma, K. L. Law, C. C. Monnahan, L. Lebreton, A. McGivern, E. Murphy, et al. 2020. “Predicted Growth in Plastic Waste Exceeds Efforts to Mitigate Plastic Pollution.” Science 369 (6510): 1515–1518. https://doi.org/10.1126/science.aba3656.
- Chen, H., M. Tang, X. Yang, Y. F. Tsang, Y. Wu, D. Wang, Y. Zhou, et al. 2021. “Polyamide 6 Microplastics Facilitate Methane Production During Anaerobic Digestion of Waste Activated Sludge.” Journal of Chemical Engineering 408:127251–127261. https://doi.org/10.1016/j.cej.2020.127251.
- Chen, H., Z. Zou, M. Tang, X. Yang, and Y. F. Tsang. 2023. “Polycarbonate Microplastics Induce Oxidative Stress in Anaerobic Digestion of Waste Activated Sludge by Leaching Bisphenol -A.” Journal of Hazardous Materials 443:130158. https://doi.org/10.1016/j.jhazmat.2022.130158.
- Dogruel, S., Z. Cetinkaya Atesci, E. Aydin, and E. Pehlivanoglu-Mantas. 2020. “Ozonation in Advanced Treatment of Secondary Municipal Wastewater Effluents for the Removal of Micropollutants.” Environmental Science and Pollution Research International 27 (36): 45460–45475. https://doi.org/10.1007/s11356-020-10339-5.
- Doo, J. P. V. K. S. 2019. “Tehnološki podatki.” https://www.vokasnaga.si/o-druzbi/centralna-cistilna-naprava-ljubljana/tehnoloski-podatki.
- Eerkes-Medrano, D., R. C. Thompson, and D. C. Aldridge. 2015. “Microplastics in Freshwater Systems: A Review of the Emerging Threats, Identification of Knowledge Gaps and Prioritisation of Research Needs.” Water Research 75:63–82. https://doi.org/10.1016/j.watres.2015.02.012.
- Gao, F., J. Li, C. Sun, L. Zhang, F. Jiang, W. Cao, L. Zheng, et al. 2019. “Study on the Capability and Characteristics of Heavy Metals Enriched on Microplastics in Marine Environment.” Marine Pollution Bulletin 144:61–67. https://doi.org/10.1016/j.marpolbul.2019.04.039.
- Gatidou, G., O. S. Arvaniti, and A. S. Stasinakis. 2019. “Review on the Occurrence and Fate of Microplastics in Sewage Treatment Plants.” Journal of Hazardous Materials 367:504–512. https://doi.org/10.1016/j.jhazmat.2018.12.081.
- Hatinoğlu, M. D., and F. D. Sanin. 2021. “Sewage Sludge As a Source of Microplastics in the Environment: A Review of Occurrence and Fate During Sludge Treatment.” Journal of Environmental Management 295:113028–113028. https://doi.org/10.1016/j.jenvman.2021.113028.
- He, Z. W., W. Z. Liu, C. C. Tang, B. Liang, Z. C. Guo, L. Wang, Y.-X. Ren, et al. 2019. “Performance and Microbial Community Responses of Anaerobic Digestion of Waste Activated Sludge to Residual Benzalkonium Chlorides.” Energy Conversion and Management 202:112211–112220. https://doi.org/10.1016/j.enconman.2019.112211.
- Hermabessiere, L., A. Dehaut, I. Paul-Pont, C. Lacroix, R. Jezequel, P. Soudant, G. Duflos, et al. 2017. “Occurrence and Effects of Plastic Additives on Marine Environments and Organisms: A Review.” Chemosphere (Oxford) 182:781–793. https://doi.org/10.1016/j.chemosphere.2017.05.096.
- He, Z. W., C. C. Tang, W. Z. Liu, Y. X. Ren, Z. C. Guo, A. J. Zhou, L. Wang, et al. 2019. “Enhanced Short-Chain Fatty Acids Production from Waste Activated Sludge with Alkaline Followed by Potassium Ferrate Treatment.” Bioresource Technology 289:121642. https://doi.org/10.1016/j.biortech.2019.121642.
- He, G. J., T. T. Zheng, D. M. Ke, X. W. Cao, X. C. Yin, and B. P. Xu. 2015. “Impact of Rapid Ozone Degradation on the Structure and Properties of Polypropylene Using a Reactive Extrusion Process.” RSC Advances 5 (55): 44115–20. https://doi.org/10.1039/c5ra06652b.
- Horton, A. A., R. K. Cross, D. S. Read, M. D. Jürgens, H. L. Ball, C. Svendsen, J. Vollertsen, and A. C. Johnson. 2021. “Semi-Automated Analysis of Microplastics in Complex Wastewater Samples.” Environmental Pollution (1987) 268 (Pt A): 115841–115851. https://doi.org/10.1016/j.envpol.2020.115841.
- ISO 11734:1995. 2017. Water Quality-Evaluation of the “Ultimate” Anaerobic Biodegradability of Organic Compounds in Digested Sludge-Method by Measurement of the Biogas Production. Geneve: International Organisation for Standardisation.
- Jagadabhi, P. S., P. Kaparaju, A. VäVäIsänenänen, and J. Rintala. 2019. “Effect of Macro- and Micro-Nutrients Addition During Anaerobic Mono-Digestion of Grass Silage in Leach-Bed Reactors.” Environmental Technology 40 (4): 418–429. https://doi.org/10.1080/09593330.2017.1393462.
- Lee, Y. J., and D. J. Lee. 2019. “Impact of adding metal nanoparticles on anaerobic digestion performance – A review.” Bioresource Technology 292:121926–121926. https://doi.org/10.1016/j.biortech.2019.121926.
- Li, X., L. Chen, Q. Mei, B. Dong, X. Dai, G. Ding, E. Y. Zeng, et al. 2018. “Microplastics in Sewage Sludge from the Wastewater Treatment Plants in China.” Water Research 142:75–85. https://doi.org/10.1016/j.watres.2018.05.034.
- Li, J., M. Dagnew, and M. B. Ray. 2023. “Microfibers in anaerobic digestion: Effect of ozone pretreatment.” Journal of Environmental Management 346:118792. https://doi.org/10.1016/j.jenvman.2023.118792.
- Li, H. X., G. J. Getzinger, P. L. Ferguson, B. Orihuela, M. Zhu, and D. Rittschof. 2016. “Effects of Toxic Leachate from Commercial Plastics on Larval Survival and Settlement of the Barnacle Amphibalanus Amphitrite.” Environmental Science & Technology 50 (2): 924–931. https://doi.org/10.1021/acs.est.5b02781.
- Li, X., Q. Mei, L. Chen, H. Zhang, B. Dong, X. Dai, C. He, et al. 2019. “Enhancement in Adsorption Potential of Microplastics in Sewage Sludge for Metal Pollutants After the Wastewater Treatment Process.” Water Research 157:228–237. https://doi.org/10.1016/j.watres.2019.03.069.
- Li, J., K. Zhang, and H. Zhang. 2018. “Adsorption of Antibiotics on Microplastics.” Environmental Pollution (1987) 237:460–467. https://doi.org/10.1016/j.envpol.2018.02.050.
- Lv, X., Q. Dong, Z. Zuo, Y. Liu, X. Huang, and W.-M. Wu. 2019. “Microplastics in a Municipal Wastewater Treatment Plant: Fate, Dynamic Distribution, Removal Efficiencies, and Control Strategies.” Journal of Cleaner Production 225:579–586. https://doi.org/10.1016/J.JCLEPRO.2019.03.321.
- Ma, H., Y. Guo, Yu Qin, and Y.-Y. Li. 2018. “Nutrient Recovery Technologies Integrated with Energy Recovery by Waste Biomass Anaerobic Digestion.” Bioresource Technology 269:520–531. https://doi.org/10.1016/j.biortech.2018.08.114.
- Mohsen, M., Q. Wang, L. Zhang, L. Sun, C. Lin, and H. Yang. 2019. “Heavy Metals in Sediment, Microplastic and Sea Cucumber Apostichopus Japonicus from Farms in China.” Marine Pollution Bulletin 143:42–49. https://doi.org/10.1016/j.marpolbul.2019.04.025.
- Müller, A., R. Becker, U. Dorgerloh, F.-G. Simon, and U. Braun. 2018. “The Effect of Polymer Aging on the Uptake of Fuel Aromatics and Ethers by Microplastics.” Environmental Pollution (1987) 240:639–646. https://doi.org/10.1016/j.envpol.2018.04.127.
- Murphy, F., C. Ewins, F. Carbonnier, and B. Quinn. 2016. “Wastewater Treatment Works (WwTW) As a Source of Microplastics in the Aquatic Environment.” Environmental Science & Technology 50 (11): 5800–5808. https://doi.org/10.1021/acs.est.5b05416.
- Nakao, S., K. Akita, A. Ozaki, K. Masumoto, and T. Okuda. 2021. “Circulation of Fibrous Microplastic (Microfiber) in Sewage and Sewage Sludge Treatment Processes.” The Science of the Total Environment 795:148873–148873. https://doi.org/10.1016/j.scitotenv.2021.148873.
- Noro, K., and Y. Yabuki. 2021. “Photolysis of Polycyclic Aromatic Hydrocarbons Adsorbed on Polyethylene Microplastics.” Marine Pollution Bulletin 169:112561–112561. https://doi.org/10.1016/j.marpolbul.2021.112561.
- Okoffo, E. D., S. O’Brien, J. W. O’Brien, B. J. Tscharke, and K. V. Thomas. 2019. “Wastewater Treatment Plants As a Source of Plastics in the Environment: A Review of Occurrence, Methods for Identification, Quantification and Fate.” Environmental Science Water Research & Technology 5 (11): 1908–1931. https://doi.org/10.1039/C9EW00428A.
- Padervand, M., E. Lichtfouse, D. Robert, and C. Wang. 2020. “Removal of Microplastics from the Environment. A Review.” Environmental Chemistry Letters 18 (3): 807–828. https://doi.org/10.1007/s10311-020-00983-1.
- Pittura, L., A. Foglia, Ç. Akyol, G. Cipolletta, M. Benedetti, F. Regoli, A. L. Eusebi, et al. 2021. “Microplastics in Real Wastewater Treatment Schemes: Comparative Assessment and Relevant Inhibition Effects on Anaerobic Processes.” Chemosphere (Oxford) 262:128415–128415. https://doi.org/10.1016/j.chemosphere.2020.128415.
- Rashid, A. N., K. Tsuru, and K. Ishikawa. 2015. “Effect of Calcium-Ozone Treatment on Chemical and Biological Properties of Polyethylene Terephthalate.” Journal of Biomedical Materials Research Part B, Applied Biomaterials 103 (4): 853–860. https://doi.org/10.1002/jbm.b.33260.
- Rasmussen, L. A., L. Iordachescu, S. Tumlin, and J. Vollertsen. 2021. “A Complete Mass Balance for Plastics in a Wastewater Treatment Plant - Macroplastics Contributes More Than Microplastics.” Water Research (Oxford) 201:117307–117307. https://doi.org/10.1016/j.watres.2021.117307.
- Schollée, J. E., J. Hollender, and C. S. McArdell. 2021. “Characterization of Advanced Wastewater Treatment with Ozone and Activated Carbon Using LC-HRMS Based Non-Target Screening with Automated Trend Assignment.” Water Research (Oxford) 200:117209–117209. https://doi.org/10.1016/j.watres.2021.117209.
- Shao, Y., X. Zhou, X. Liu, and L. Wang. 2020. “Pre-Oxidization-Induced Change of Physicochemical Characteristics and Removal Behaviours in Conventional Drinking Water Treatment Processes for Polyethylene Microplastics.” RSC Advances 10 (68): 41488–41494. https://doi.org/10.1039/D0RA07953G.
- Sun, J., X. Dai, Q. Wang, Mark C.M. van Loosdrecht, and B.-J. Ni. 2019. “Microplastics in Wastewater Treatment Plants: Detection, Occurrence and Removal.” Water Research 152:21–37. https://doi.org/10.1016/j.watres.2018.12.050.
- Talvitie, J., A. Mikola, A. Koistinen, and O. Setälä. 2017. “Solutions to Microplastic Pollution – Removal of Microplastics from Wastewater Effluent with Advanced Wastewater Treatment Technologies.” Water Research 123:401–407. https://doi.org/10.1016/j.watres.2017.07.005.
- Talvitie, J., A. Mikola, O. Setälä, M. Heinonen, and A. Koistinen. 2017. “How Well Is Microlitter Purified from Wastewater? – a Detailed Study on the Stepwise Removal of Microlitter in a Tertiary Level Wastewater Treatment Plant.” Water Research 109:164–172. https://doi.org/10.1016/j.watres.2016.11.046.
- Wang, Y., D. Wang, N. Yi, Y. Li, B. J. Ni, Q. Wang, H. Wang, et al. 2020. “Insights into the Toxicity of Troclocarban to Anaerobic Digestion: Sludge Characteristics and Methane Production.” Journal of Hazardous Materials 385:121615–121624. https://doi.org/10.1016/j.jhazmat.2019.121615.
- Wei, W., X. Chen, L. Peng, Y. Liu, T. Bao, and B. J. Ni. 2021. “The Entering of Polyethylene Terephthalate Microplastics into Biological Wastewater Treatment System Affects Aerobic Sludge Digestion Differently from Their Direct Entering into Sludge Treatment System.” Water Research (Oxford) 190:116731–116742. https://doi.org/10.1016/j.watres.2020.116731.
- Wei, W., Q.-S. Huang, J. Sun, X. Dai, and B. J. Ni. 2019. “Revealing the Mechanisms of Polyethylene Microplastics Affecting Anaerobic Digestion of Waste Activated Sludge.” Environmental Science & Technology 53 (16): 9604–9613. https://doi.org/10.1021/acs.est.9b02971.
- Wei, W., Q. S. Huang, J. Sun, J. Y. Wang, S. L. Wu, and B. J. Ni. 2019. “Polyvinyl Chloride Microplastics Affect Methane Production from the Anaerobic Digestion of Waste Activated Sludge Through Leaching Toxic Bisphenol-A.” Environmental Science and Technology 53 (5): 2509–2517. https://doi.org/10.1021/acs.est.8b07069.
- Wei, W., Y.-T. Zhang, Q.-S. Huang, and B.-J. Ni. 2019. “Polyethylene Terephthalate Microplastics Affect Hydrogen Production from Alkaline Anaerobic Fermentation of Waste Activated Sludge Through Altering Viability and Activity of Anaerobic Microorganisms.” Water Research 163:114881. https://doi.org/10.1016/j.watres.2019.114881.
- Wu, M., W. Tang, S. Wu, H. Liu, and C. Yang. 2021. “Fate and Effects of Microplastics in Wastewater Treatment Processes.” The Science of the Total Environment 757:143902–143913. https://doi.org/10.1016/j.scitotenv.2020.143902.
- Xiong, P., X. Yan, Q. Zhu, G. Qu, J. Shi, C. Liao, G. Jiang, et al. 2019. “A Review of Environmental Occurrence, Fate, and Toxicity of Novel Brominated Flame Retardants.” Environmental Science & Technology 53 (23): 13551–13569. https://doi.org/10.1021/acs.est.9b03159.
- Xu, X., Y. Jian, Y. Xue, Q. Hou, and L. Wang. 2019. “Microplastics in the Wastewater Treatment Plants (WWTPs): Occurrence and Removal.” Chemosphere 235:1089–1096. https://doi.org/10.1016/j.chemosphere.2019.06.197.
- Yang, L., et al. 2019. “Removal of Microplastics in Municipal Sewage from China’s Largest Water Reclamation Plant.” Water Research (Oxford) 155:175–181. https://doi.org/10.1016/j.watres.2019.02.046.
- Zhang, X., J. Chen, and J. Li. 2020a. “The Removal of Microplastics in the Wastewater Treatment Process and Their Potential Impact on Anaerobic Digestion Due to Pollutants Association.” Chemosphere 251:251. https://doi.org/10.1016/j.chemosphere.2020.126360.
- Zhang, X., J. Chen, and J. Li. 2020b. “The Removal of Microplastics in the Wastewater Treatment Process and Their Potential Impact on Anaerobic Digestion Due to Pollutants Association.” Chemosphere (Oxford) 251:126360–126360. https://doi.org/10.1016/j.chemosphere.2020.126360.
- Zhang, Y. T., W. Wei, Q.-S. Huang, C. Wang, Y. Wang, and B.-J. Ni. 2020. “Insights into the Microbial Response of Anaerobic Granular Sludge During Long-Term Exposure to Polyethylene Terephthalate Microplastics.” Water Research (Oxford) 179:115898–115898. https://doi.org/10.1016/j.watres.2020.115898.
- Zhang, J., J. Zhang, Yu Tian, N. Li, L. Kong, Li Sun, M. Yu, and W. Zuo. 2016. “Changes of Physicochemical Properties of Sewage Sludge During Ozonation Treatment: Correlation to Sludge Dewaterability.” Journal of Chemical Engineering 301:238–248. https://doi.org/10.1016/j.cej.2016.04.151.
- Zhu, X., C. Hao, M. Zhang, and B. Lan. 2022. “Enhanced Adsorption of Bromoform Onto Microplastic Polyethylene Terephthalate Exposed to Ozonation and Chlorination.” Molecules (Basel, Switzerland) 28 (1): 259–278. https://doi.org/10.3390/molecules28010259.
- Ziajahromi, S., P. A. Neale, L. Rintoul, and Frederic D.L. Leusch. 2017. “Wastewater Treatment Plants As a Pathway for Microplastics: Development of a New Approach to Sample Wastewater-Based Microplastics.” Water Research 112:93–99. https://doi.org/10.1016/j.watres.2017.01.042.