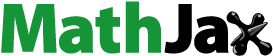
Abstract
Introduction
The aim of this study was to assess the safety of bronchoscopic microwave ablation (MWA) of peripheral lung parenchyma using the NEUWAVE™ FLEX Microwave Ablation System, and robotic-assisted bronchoscopy (RAB) using the MONARCH™ Platform in a swine model.
Methods
Computed tomography (CT)-guided RAB MWA was performed in the peripheral lung parenchyma of 17 Yorkshire swine (40–50 kg) and procedural adverse events (AEs) documented. The acute group (day 0, n = 5) received 4 MWAs at 100 W for 1, 3, 5, and 10 min in 4 different lung lobes. Subacute and chronic groups (days 3 and 30, n = 6 each) received one MWA (100 W, 10 min) per animal.
Results
The study was completed without major procedural complications. No postprocedural AEs including death, pneumothorax, bronchopleural fistula, hemothorax, or pleural effusions were observed. No gross or histological findings suggestive of thromboembolism were found in any organ. One 3-Day and one 30-Day swine exhibited coughing that required no medication (minor AEs), and one 30-Day animal required antibiotic medication (major AE) for a suspected lower respiratory tract infection that subsided after two weeks. CT-based volumetric estimates of ablation zones in the acute group increased in an ablation time-dependent (1–10 min) manner, whereas macroscopy-based estimates showed an increasing trend in ablation zone size.
Conclusion
The NEUWAVE FLEX and MONARCH devices were safely used to perform single or multiple RAB MWAs. The preclinical procedural safety profile of RAB MWA supports clinical research of both devices to investigate efficacy in select patients with oligometastatic disease or primary NSCLC.
1. Introduction
Percutaneous thermal ablation in which heat is generated by electromagnetic energy is an accepted treatment modality for patients with primary and metastatic lung malignancies. Microwave ablation (MWA) and radiofrequency ablation (RFA) of lung lesions are two percutaneous ablation procedures currently in widespread clinical use [Citation1]. MWA may be a more effective thermal ablation approach than RFA to treat pulmonary tumors because the lungs exhibit a higher penetration of microwaves than radiofrequency energy due to the high impedance path presented by inflated lung tissue [Citation2]. Diversion of the heat current during ablation in organs with high blood perfusion, or when ablation probes are located near prominent vascular heat sinks, is more pronounced with RFA than MWA [Citation3–5] resulting in larger MWA zones, less irregular MWA edges, and shorter MWA procedural times [Citation2,Citation5–7].
The high incidence of pleural complications (e.g., pneumothorax, pleural effusions) linked to percutaneous hyperthermic ablation methods [Citation8–11] has triggered the search for safer therapeutic approaches for lung tumors. Robotic-assisted bronchoscopy (RAB) has been shown to improve the reaching and targeting of artificial tumors in peripheral lung regions compared to conventional thin bronchoscopes [Citation12,Citation13]. Furthermore, two feasibility studies using RAB showed either no serious AEs [Citation14] or an AE rate (3.7%) comparable to conventional bronchoscopy [Citation15]. The emergence of RAB to safely sample peripheral pulmonary lesions has driven the combined use of key technologies including flexible microwave probes, electromagnetic navigation bronchoscopy (ENB), augmented fluoroscopy, cone beam computed tomography (CBCT), and RAB systems to enable transbronchial ablation of peripheral pulmonary lesions safely and effectively [Citation16–19]. Thus, bronchoscopic microwave ablation (MWA) is a promising minimally invasive approach that has emerged as a safe alternative to percutaneous approaches to reduce the incidence of pleural complications [Citation18,Citation19].
The purpose of this study was to evaluate the safety of a RAB MWA system, the NEUWAVE™ FLEX Microwave Ablation System (NEUWAVE FLEX, Ethicon, Madison, WI) and a robotic bronchoscope, the MONARCH™ Platform, in the peripheral lung parenchyma of healthy swine. RAB MWA was performed with the NEUWAVE FLEX while the MONARCH Platform was utilized for airway access and navigation, and bronchoscopic visualization. The primary endpoint was incidence of procedural complications including pneumothorax, hemothorax and bronchopleural fistulas. Additional findings from volume measurements of the ablation zone obtained from CT scans and gross pathology sections are reported.
2. Materials and methods
2.1. Test Materials
The medical devices tested included NEUWAVE FLEX, a flexible, CO2-cooled MWA system designed for use with endoscopic working channels, and the MONARCH Platform, a robotic bronchoscope (). MWA ablation was performed with a NEUWAVE FLEX ablation probe while MONARCH was utilized to gain airway access and visualization for microwave ablation probe delivery (). MONARCH enables electromechanical, user-controlled navigation of a flexible bronchoscope using a hand-held controller. The MONARCH Platform integrates a preoperative CT segmentation into a graphical user interface to intraoperatively display the bronchoscope’s tip location relative to the preoperative scan anatomy. Microwave energy is delivered by NEUWAVE FLEX through a single channel to the ablation probe, and a touch-screen user interface controls the system and enables the user to define ablation settings (). MONARCH’s working channel lumen diameter is 2.1 mm, and the outer diameters of scope and sheath are 4.4 mm and 6.0 mm, respectively [Citation20], whereas the NEUWAVE FLEX ablation probes are French gauge 6 with outer diameters of less than 2 mm [Citation21].
Figure 1. Medical devices and probes utilized during the RAB MWA in swine lungs. (A) The MONARCH™ Platform, a robotic bronchoscopy system, consists of a navigation tower and a robotic cart (a). The MONARCH navigation tower includes a display for CT-guided bronchoscopic navigation and visualization of the lung airways, whereas the MONARCH cart houses the robotic arms that control the bronchoscope. The NEUWAVE™ FLEX Microwave Ablation System (b) consists of a touch screen display as the user interface, a microwave power amplifier, and a CO2-based cooling system. (B) NEUWAVE FLEX ablation probe inserted through the MONARCH working channel. (C) Fluoroscopic image of a MWA procedure performed in one of the animals included in the study (ID: 1) showing the contour of the probe inserted into the working channel. (D) Screenshot of the NEUWAVE touchscreen user interface depicting ablation settings.

2.2. Study design
The primary endpoint of the study was the incidence of procedural complications after RAB MWA of normal peripheral lung parenchyma in swine. The experimental design consisted of 3 groups of swine for acute (Non-Survival, n = 5), subacute (3 days, n = 6), and chronic (30 days, n = 6) safety assessments. Swine were selected for this investigation based on the similarities to humans in physiology, immunology, and genetics [Citation22,Citation23], and their extensive use in medical device development and testing of lung ablative therapies [Citation24–27]. The number of animals used reflects historical data from previous acute animal studies conducted by the study sponsor in swine (n = 5–6), where multiple liver abrasion lesions per animal were performed and several topical hemostats tested [Citation28,Citation29]. This approach is consistent with current guidelines in animal research aimed at reducing the use of animals [Citation30].
Four preliminary MWA studies (n = 22) were conducted to select power and ablation times, verify lung tissue staining after IV delivery of 2,3,5-triphenyltetrazolium chloride (TTC), assess device performance and procedural safety, and optimize procedural workflow. All animals in the 4 preliminary studies in the acute, subacute, and chronic follow ups survived the single or multiple MWAs without procedural or post-ablation clinical complications.
Secondary endpoints included volumetric measurements of the ablation zones determined in CT scans and gross pathology tissue slices. The study was conducted in compliance with good laboratory practices (GLP) as defined in 21 CFR Part 58: GLP for nonclinical laboratory studies.
2.3. Animals
Castrated male Yorkshire swine weighing 40–50 kg were used in the study. Animals were evaluated upon arrival by a veterinarian, ear-tagged and acclimated to the testing facility for at least 5 days before any ablation procedure was conducted. Animals were individually or group-housed, fed once a day with standard pig chow and had access to water ad libitum. Housing and husbandry conformed to the standards of the Guide for the Care and Use of Laboratory Animals [Citation30]. The study was conducted by the CRF Skirball Center for Innovation (SCI, Orangeburg NY, USA) in their AAALAC International accredited facility. Animal protocols were reviewed and approved by SCI’s Institutional Animal Care and Use Committee (IACUC). Complete blood count and serum chemistry were obtained during the acclimation period, on the day of the procedure (Day 0), before and after the bronchoscopic procedure, and 3, 7, 14 and 30 days after the procedure. Arterial blood gases were analyzed on Day 0, before and after the procedure, and at post-ablation days 3 and 30. Clinical assessments were continued after the procedures were completed up to terminal follow-ups (3 and 30 days).
2.4. Swine RAB/MWA model
Animals were anesthetized with intramuscular injections of a combination of tiletamine (3 mg/kg), zolazepam (3 mg/kg) (Telazol®), and ketamine (5 mg/kg), followed by endotracheal intubation and maintenance of anesthesia by inhalation of isoflurane (1–5%) mixed with 100% oxygen (∼1.5 L/min). Mechanical ventilation (10–20 mL/kg, 10–15 respirations/minute) was used throughout the procedure. A peripheral vein was catheterized for delivery of fluids and drugs as needed. Fluids (0.9% saline) were administered intravenously (IV) at a rate of 5–10 mL/kg/h throughout the bronchoscopic procedure. Blood pressure was measured invasively in a peripheral artery (e.g., auricular, median, or saphenous) using a catheter connected to a transducer. Heart and respiratory rates, electrocardiography, CO2, body temperature, and mean arterial pressure were monitored continuously. The fluid infusion rate was adjusted as needed to maintain physiologic blood pressure levels prior to initiating the bronchoscopic procedure. Animals received analgesic medication (non-steroidal anti-inflammatory drugs) prior to completion of the procedure and continued for several days post-ablation as needed.
Preprocedural CT images were obtained and imported into the MONARCH Platform’s software to create a bronchoscopic navigational plan and guidance to the target region. Briefly, a preoperative non-contrast CT scan was performed with a 2 × 128-slice multi-detector CT scanner (Siemens Healthineers Somatom Definition Flash) using a standard dose exposure and a thin slice protocol (1.5 mm thickness, 1.2 pitch, 0.5 s/rotation, 100–120 kV, 200 mA). Animals were imaged in supine position. During breath hold, a volumetric preprocedural CT scan extending from the thoracic inlet to the upper abdomen was completed immediately prior to ablation. The CT scans were then imported into the MONARCH Pre-Op Planning Application for the operator to create the navigation plan to the selected ablation site. The procedural objective was to use the MONARCH bronchoscope to navigate beyond the inner 1/3 of a lung into a subsegmental (sublobar), third-order bronchus to ablate normal pulmonary tissue located in the outer 2/3 s of the lung. Therefore, all ablations in the current study were consistently performed endobronchially, from within the peripheral airways, because no target tumors were present in the swine lungs. In the clinical setting, however, the ablation probe may penetrate the bronchial wall to reach a target tumor. Once the bronchoscope was positioned in the planned ablation site inside a subsegmental bronchus, the NEUWAVE FLEX ablation probe was inserted through the MONARCH working channel (). The probe tip was advanced under fluoroscopy guidance out of the bronchoscope and further into the sublobar bronchus planned ablation site (). The bronchoscope was retracted out of the planned ablation site and intraprocedural cone beam CT (CBCT) high quality images (30 s, 400 projection images per scan) were acquired with a Siemens Cios Spin Mobile C-Arm during breath hold to confirm the final probe location. Probe position was adjusted if needed and an updated CBCT image acquired prior to initiating ablation. CBCT was repeated at the end of the ablation treatment to document probe position relative to the ablation zone and assess for any procedural complication while the animal was still intubated.
Non-contrast and contrast-enhanced postprocedural CT images were obtained for all animals immediately following ablation completion of all sites at day 0 and at terminal follow-ups for animals in the subacute (3-Day) and chronic (30-Day) groups. Contrast-enhanced CT retained the same non-contrast CT parameters except for the administration of a nonionic, iodine-based, contrast agent (ISOVUE-370) as follows: 40–70 mL of contrast media (320–370 mg/mL) was delivered IV at a flow rate of 3–4 mL/s followed by 50 mL of saline at the same rate. Initiation of scanning was synchronized to the arrival of the contrast material to the pulmonary trunk using automated peak enhancement detection.
Each animal in the acute, Non-Survival group had 4 ablations of 1-, 3-, 5-, and 10-min duration, whereas animals in the subacute and chronic groups received a single 10-min ablation procedure to evaluate the safety of the longest possible single ablation time allowed by the system. All ablations were performed at the maximum system power setting (100 W at the user interface) to assess safety under maximal power output. [Citation21]. NEUWAVE FLEX, the noncommercial investigational device used in the current study, has an estimated efficiency of 45% due to the combined power attenuation from the delivery and antenna cables. Ablation areas in the Non-Survival group were selected on CT images based on anatomical feasibility and performed in a randomized alternate pattern between the left cranial and right middle lobes for 1- and 3-min ablations, and the left and right caudal lobes for 5- and 10-min ablations. A single area of the right or left caudal lobes was randomly selected for 10-min ablations in the 3- and 30-Day groups.
2.5. Gross pathology and tissue processing for histopathology
After CT scans were acquired, a bolus of heparin (10,000 U) and 25 mL of a 10% solution of TTC were administered IV to aid in distinguishing non-viable from viable tissue in the ablation zones [Citation31,Citation32]. Animals were euthanized while under anesthesia (acute group) and at post-ablation days 3 and 30 by an IV injection of 10 mL pentobarbital sodium (390 mg/mL) and phenytoin (50 mg/mL) (Euthasol). On termination day, necropsy and gross pathology were conducted and pulmonary ablation sites collected and processed for histopathological evaluation and volumetric assessments of the ablation sites. Explanted lungs were filled through the trachea with 10% formalin at a pressure of 20 cm H20 (14.7 mm Hg) to the approximate size of the lungs at full inspiratory volume. The trachea was then ligated, and lungs immersed in 10% formalin using 5-gallon (22.7 L) sealable containers for 24–48 h before tissue trimming. Trimmed tissue sections from the ablation sites were embedded in paraffin blocks, and thin sections obtained and processed for H&E staining. Histopathological analysis was conducted by a veterinary pathologist.
2.6. Histological scoring of the MWA sites
The extent of alveolar edema, inflammation, hemorrhage, and vascular thrombosis in ablation treatment sites was scored using semiquantitative methods. Ablation sites were independently scored in low and high-power photomicrographs by the study pathologist by estimating the percent lesion area associated with the histopathological variable. For example, ablation sites were scored as follows for alveolar edema: 0: no edema present; 1: occasional areas of edema present (‘minimal’); 2: edema associated with up to 25% of the lesion area (‘mild’); 3: edema associated with 25–50% of the lesion area (‘moderate’); 4: edema associated with 50–75% of the lesion area (‘moderate to marked’); and 5: edema associated with 75–100% of the lesion area (‘marked’). The same scoring system was used to assess the presence of inflammation (inflammatory cells present by percent lesion area) and hemorrhage (percent of lesion area). Vascular thrombosis was scored as follows: 0: no intravascular thrombosis present in the lesion; 1: occasional/rare foci of intravascular thrombosis (‘minimal’); 2, 3, 4 and 5: intravascular thrombosis associated with 5–10% (‘mild’) 10–20% (‘moderate’), 20–40% (‘moderate to marked’), and >40% (‘marked’) of blood vessels within the lesion, respectively.
2.7. Adverse events (AEs)
Safety was defined as the absence of unreasonable risk of illness or injury in the form of AEs associated with the use of the devices for their intended use and conditions of use according to FDA 21 CFR 860.7 (d) for investigations using laboratory animals [Citation33]. AEs were assessed according to the standardized Society of Interventional Radiology (SIR) classification system [Citation34,Citation35]. SIR defines a major complication (grades C to F) as an event that leads to substantial morbidity and disability and increases the level of care (e.g., placement of a chest tube following pneumothorax, antibiotic therapy in addition to prophylactic antibiotics). All other complications are considered minor and graded A (no therapy required) or B (nominal therapy). Peri- and post-procedural clinical assessments were performed daily by a staff veterinarian for all animals in the 3 experimental groups.
2.8. Volumetric measurements of the ablation zone by CT and gross pathology
Contrast-enhanced postprocedural CT images obtained immediately following ablation completion of all sites at Day 0 and at terminal follow-up for subacute (3-Day) and chronic (30-Day) sites were used to assess volumes of the MWA zones (see Section 2.4). In CT scans, the acute ablation treatment zone consists of radiologically defined ‘ground-glass’ opacities (GGOs), areas of heterogeneously increased attenuation observed in the lung parenchyma surrounded by a hyperdense rim. Increased attenuated areas of soft tissue adjacent to the ablation site were also measured for the 3-Day and 30-Day time points due to the expected contraction/involution of the ablated tissues [Citation35]. Volumetric measurements based on an ellipsoid approximation ( L: length, Dmax: maximum diameter, Dmin: minimum diameter) were used to dimensionally characterize the ablation zone in CT images.
Following formalin-fixation, lung tissues containing the ablation sites were serially sectioned at approximately 5 mm thickness and digital images of the cranial and caudal surfaces of each section obtained. The caudal surface of the ablation zone within each 5-mm section was measured with a calibrated ruler. The outer edge of the ablation zone was defined by the inner border of a hyperemic rim in Non-Survival (acute) and 3-Day (subacute) animals, and by the inner border of a fibroproliferative rim in 30-Day animals. Dmin, Dmax, and Length were obtained from the serial sections and used to estimate the ablation site volume using the ellipsoid formula.
2.9. Statistical analysis
Descriptive statistics including minimum, first quartile (Q1), median, third quartile (Q3), maximum, mean, and standard deviation (SD) are provided for all datasets of CT- and gross pathology-determined linear measurements and ablation zone volumes. Since the primary endpoint of the study was safety, no formal statistical hypothesis was tested.
3. Results
3.1. Clinical evaluation
One animal of the 3-Day group (ID:7) exhibited cough on post-procedural day 1, and a 30-Day swine (ID:13) had a single episode of dry cough during recovery from sedation for blood collection at day 3 (). Both animals recovered the same day without therapy. Another 30-Day animal (ID: 15) had recurrent episodes of coughing and abnormal respiratory sounds that started 6 days after ablation. Clinical and hematologic evaluations were consistent with a lower respiratory tract infection. Antimicrobial therapy (tulathromycin) was initiated 6 days after ablation for this animal with the resolution of all clinical signs two weeks later (). Clinical Pathology. No alterations of the complete blood count (CBC) or serum clinical chemistry panel were found in any swine included in the study, except for leukocytosis and mild neutrophilia in a 30-Day swine (ID: 15). Deviations from reference intervals in the rest of swine were consistent with minor changes in individual animals and had no clinical impact. RAB MWA had no effect on gas exchange/ventilation as shown in results from blood gas analyses performed on ablation/treatment day and at termination day.
Table 1. AEs, radiologic (CT), pathologic, and molecular (PCR) assessments of lung tissue.
3.2. CT imaging
Post-ablation CT images revealed GGOs in all ablation sites in the lungs of Non-Survival (), and 3- and 30-Day swine (). Decreased opacities and varying degrees of atelectasis were observed in the ablation zone at the terminal follow-up time points (3- and 30 days) (). Cavitation, defined as presence of gas within the ablation areas, was observed in axial planes of CT images in three 3-Day (3/6, 50%), and two 30-Day (2/6, 33.3%) animals (). No signs of cavitation were observed in CT images of Non-Survival animals or in Day 0 post-ablation CT scans of 3- and 30-Day swine.
Figure 2. Representative post-ablation contrast-enhanced CT imaging findings in a Non-Survival (acute) animal. Ablations were performed at 100 W in the left cranial (A, 1 min), right middle (B, 3 min), left caudal (C, 5 min), and right caudal (D, 10 min), regions of the lung. Coronal plane images are shown. Ablation zones exhibit GGO areas of heterogeneously increased attenuation (white arrows).

Figure 3. Representative post-ablation, contrast-enhanced CT images for 3-Day (A,B) and 30-Day (C,D) animals. MWAs (100 W, 10 min) were performed in the left caudal regions of the lungs in both animals. Regions where ablations were performed show typical GGO at day 0 in lungs of 3-Day (A) and 30-Day (C) animals. At Day 3, significant tissue density and some atelectasis were observed in the ablation region on Day 3 (B), whereas contraction/involution is shown in the ablation region on Day 30 (D).

3.3. Gross pathology
3.3.1. MWA target sites
MWA treatment sites were uniformly palpable within the lung parenchyma and identifiable as well-demarcated zones of dark, red discoloration. The Non-Survival and 3-Day ablation treatment sites exhibited outer (dark discoloration) and inner (light discoloration) areas (). After fixation in formalin, the lesions exhibit a central lighter colored region surrounded by dark discoloration (). Chronic, 30-Day ablation treatment sites were firm, well-demarcated fibrotic regions of light tan/white discoloration. Post-ablation lung voids (cavitation) were found at the treatment sites of 3- and 30-Day animals. Three and 2 ablation sites at 3 (3/6, 50%) and 30 days (2/6, 33.3%), respectively, exhibited central cavities (). MWA sites of Non-Survival animals showed no signs of cavitation.
Figure 4. Necropsy photographs (A,B) and H&E histological images (C–H) of swine lungs displaying MWA treatment sites for the three experimental groups. (A) Left caudal lung lobe of a 3-day animal (ID: 9) before formalin fixation showing the treatment site on the pleural surface as a well-demarcated zone of dark red discoloration. (B) Tissue slices after formalin fixation of the right caudal lung lobe of an animal in the Non-Survival (acute) group (ID: 3) subjected to a 5-min ablation (100 W). Within the formalin-fixed lung parenchyma, the lesion is characterized by a central lighter-colored region surrounded by a zone of dark discoloration. The red and white lines indicate the MWA zone’s Length and minimum diameter (Dmin), respectively. A line orthogonal to Length and Dmin represents the maximum diameter (Dmax). (C,D) Low power images (C: 2×; D: 4×) of histological sections of a MWA site (100 W, 3-min) in the right middle lung lobe of a Non-Survival animal (ID:1). The ablation lesion is characterized by an outer zone of hyperemia (yellow star) with an internal region of degeneration/necrosis of the alveolar parenchyma (black stars). There is also degeneration of peribronchial connective tissue (brown stars). Interlobular septa are expanded due to edema (blue stars). (E,F) Low magnification (E: 2×; F: 10×) images of two regions of a MWA site in the left caudal lung lobe of a 3-Day swine (ID: 6) showing areas of coagulative necrosis (black stars), and normal tissue (green stars). The center of the image in panel E shows a region of variable hyperemia, moderate hemorrhage (yellow stars), and disorganized and degenerated alveolar tissue. Interlobular septa in both images are expanded due to edema (blue stars). (G,H) Low (G: 1.25×) and high (H: 20×) power images of a MWA treatment site in the right caudal lung lobe of a 30-Day swine (ID: 14). The low-power image in G displays a central zone of coagulative necrosis (black stars), and a large, circumferential, coalescing granulomatous lesion (red stars). A rim of fibrous tissue (arrows) demarcates the granulomatous lesion from the adjacent, normal lung parenchyma (green stars). The higher power photomicrograph in panel H displays a section of the central zone of coagulative necrosis in the ablation site (black stars), the granulomatous lymphocytic infiltrate adjacent to the necrotic core (red star), and a region of coccoid bacterial colonies (white star), which were detected in the MWA sites of four 30-Day animals.

3.3.2. Nontarget regions
There were no gross findings suggestive of thromboembolism or infarction in any non-target organ including brain, kidneys, liver, and myocardium for the 17 animals included in the study. No thermal damage to large airways proximal to the MWA site including the trachea, main bronchi, or lobar bronchi was found in any animal. Similarly, gross pathology of other organs and structures close to the navigation pathway (e.g., pericardium, heart, esophagus) revealed no thermal damage.
3.4. AEs
No clinical or radiologic (CT scans) evidence of major AEs or procedural complications leading to increased morbidity or disability were observed in Non-Survival, 3-Day or 30-Day animals, including pneumothorax, hemothorax, bronchopleural fistula, hemorrhage, hemoptysis, pleural effusion, thrombosis of midsize or large arteries in any organ (e.g., kidney, liver, brain, spleen), phrenic nerve paralysis, chronic pain, or death before terminal follow up at 3 and 30 days (). All other pneumonic findings revealed by histopathological analyses including pulmonary lesions in the MWA site, and bronchopneumonia in nontarget regions were considered non-clinical minor complications (SIR level A), as they did not increase the level of animal care required [Citation35] (). A minor procedural incident in one animal (ID:7) consisted of high reflected power during ablation as a result of spontaneous proximal migration of the ablation probe. The incident was detected by the NEUWAVE FLEX System and power delivery was automatically halted. Following repositioning of the probe and ablation completion, the animal recovered from anesthesia without complications.
Coughing exhibited by a 3-Day (ID: 7) and a 30-Day (ID:13) animal was classified as a minor AE (SIR level A) since both animals fully recovered without therapy (). However, a 30-Day swine (ID: 15) with recurrent episodes of coughing required antibiotic therapy and was thus classified as a major AE (C). The animal fully recovered two weeks later. The 3 swine that exhibited coughing (one 3-Day and two 30-Day animals) were positive for Mycoplasma hyopneumoniae. Therefore, 3 out of 17 animals (18%) exhibited clinical signs consistent with mycoplasma pneumonia confirmed by molecular testing ().
3.5. Histopathology
3.5.1. MWA target sites
Non-survival treatment sites were histologically characterized as hyperemia and coagulative necrosis (). Alveolar edema was a common finding in the ablation sites of the three experimental groups (; and ), and edema was commensurate to ablation duration in the Non-Survival group (not shown). ‘Mild’ and ‘moderate’ alveolar edema was present in 2 10-min ablation sites each, respectively, whereas a single 10-min ablation site exhibited ‘moderate to marked’ edema in the Non-Survival group (). In the 3-Day group, ‘mild’, ‘moderate’ and ‘moderate to marked’ edema was present in 2 sites for each score category (). ‘Mild’ and ‘moderate’ edema was observed in one and two sites, respectively, in the chronic, 30-Day group. Three sites of the 30-Day group showed ‘moderate to marked’ edema ().
Figure 5. Histopathological scoring of alveolar edema (A), inflammation (B), hemorrhage (C), and vascular thrombosis (D) at the MWA sites. Only ablation sites treated for 10 min are depicted for the Non-Survival group. Thus, all ablation treatment sites shown received 100 W power for 10 min. Alveolar edema was common in the 3 groups; inflammation was observed in 3- and 30-Day ablation sites, whereas hemorrhage and thrombosis were present mainly in 3-Day ablation sites.

Additional histopathological findings in and adjacent to the MWA treatment sites in 3- and 30-Day animals included inflammation, hemorrhage, and vascular thrombosis (; ). No inflammatory cells were found in any ablation site of Non-Survival animals (; and ). Variable degrees of inflammation consisting mainly of infiltrating neutrophils and macrophages were observed in ablation treatment sites of the 3-Day group, whereas the inflammatory infiltrate in ablation sites of the 30-Day group included lymphocytes and macrophages, and neutrophils to a lesser degree ( and ). In the 3-Day group, ‘mild’ and ‘moderate’ inflammation was found in 2 and 3 ablation sites, respectively. A single 3-Day site exhibited ‘marked’ inflammation. ‘Mild’ and ‘moderate’ degrees of inflammation were present in 2 and 4 ablation sites, respectively, of the 30-Day group ().
Hemorrhage was a rare finding in the MWA sites of the Non-Survival group, as only ‘minimal’ hemorrhage was observed in a single 5-min ablation site (). In the 3-Day group, ‘mild’ and ‘moderate’ hemorrhage was present in 2 and 3 ablation sites, respectively ( and ). No hemorrhage was observed in any ablation site of the 30-Day group (). No thrombus formation was found in any of the 20 ablation treatment sites (5 swine, 4 ablations per animal) in the acute, Non-Survival group, or in the 6 ablation sites of the chronic, 30-Day group (). In the 3-Day group, ‘minimal’ and ‘mild’ thrombosis, represented by fibrin-rich thrombi present in small vessels, were observed in 2 and 3 ablation sites, respectively (). No signs of thromboembolism downstream of the MWA sites were found.
Bacterial colonies (cocci) were present in the necrotic central zones and cavities of three 3-Day (3/6, 50%) and four 30-Day swine (4/6, 67%) (; ). No bacterial colonies were found in lungs of Non-Survival animals (). Pulmonary granulomatous inflammatory lesions isolated by fibrosis were observed in all ablation treatment sites of the 30-Day group (). These lesions were mostly centered on dilated bronchi/bronchioles. Ablation sites in the 30-Day group were well demarcated from adjacent normal lung parenchyma by a circumferential band of fibrous tissue that surrounded the lesion. Thus, fibrosis served to effectively isolate ablated tissue from healthy tissue.
3.5.2. Nontarget pulmonary regions
There was no histopathological evidence of thromboembolism or infarction in nontarget regions. Lesions consistent with broncho pneumonic infections of nontarget lung lobes were observed in 3 animals of the 3- and 30-Day groups each (3/6, 50%) ().
3.6. Linear dimensions and volumetric measurements of the ablation zone
Animal-level linear dimensions (Length, Dmin and Dmax) and volume determinations for each MWA site obtained from CT scans and gross pathology slices are shown in and , respectively. Descriptive statistics (mean ± SD) for all measurements within each experimental group using both methods (CT and pathology) are summarized in . Full descriptive statistics including the 5 number summary (minimum and maximum values, Q1, median, Q3, interquartile range), and the mean ± SD for all groups using both methods are detailed in Supplementary Tables S1 and S2.
Table 2. Animal-level CT imaging volume measurements of the MWA sites.
Table 3. Animal-level gross pathology measurements of the MWA sites.
Table 4. Descriptive statistics for CT imaging and gross pathology measurements of the MWA sites.
3.6.1. CT-based volumetric measurements
Volumetric estimates showed an increase in ablation volume as ablation time increased, indicating a time-dependency of the ablation procedure over the ablation time range examined (1–10 min) (; ). CT-based measurements exhibited an increase in ablation volume at days 3 and 30 compared to post-ablation measurements at procedural day 0 in the same animal groups (; ). Post-ablation volumes in swine of the 3-Day group were higher compared to 30-Day animals consistent with an involution of the ablation site at 30 days post-ablation (; ).
Figure 6. (A) MWA volume (cm3) determinations based on CT as a function of ablation time in acute, Non-Survival animals. Four ablations at 1-, 3-, 5-, and 10-min duration were performed on each of the 5 animals. Volumetric measurements showed an increase in ablation volume as ablation time increased, indicating a time-dependency of the MWA procedure over the ablation time range examined (100 W, 1–10 min). Average CT ablation zone volumes at 3, 5 and 10 min showed 1.8-, 2.7- and 4.8-fold increases, respectively, compared to the average ablation zone volume at an ablation duration of 1 min (n = 5). (B) MWA volume (cm3) based on CT scans at days 0, 3 and 30 for animals in the subacute (3-Day, n = 6) and chronic (30-Day, n = 6) groups. Ablation measurement increased at 3 days and involuted/contracted at 30 days post-ablation. (C) MWA volume (cm3) determinations by gross pathology as a function of ablation time in acute, Non-Survival animals. (D) MWA volume (cm3) measurements by gross pathology at days 0, 3 and 30 for the subacute (3-Day) and chronic (30-Day) groups of animals. Ablation measurement increased at 3 days and contracted at 30 days post-ablation. Box plots display the five-number summary for each set of data (minimum, Q1, median, Q3, and maximum). Crosses within box plots represent mean values.

3.6.2. Macroscopy-based volumetric measurements
MWA volumes of acute ablation sites showed an increasing trend in zone size from one to 10 min ablation time (; ). However, ablation zone sizes measured by gross pathology overlapped considerably in Non-Survival animals. Compared to ablation zones in Non-Survival animals at day 0, post-ablation volumes in the subacute 3-Day group increased in size () due to a circumferential expansion of a cellular response triggered by thermal ablation that includes cell death and proliferation of resident cells, recruitment of circulating inflammatory cells and local remodeling of ischemic and hypoxic tissue.
3.6.3. CT-based/macroscopy-based measurement ratios
Mean values of CT-based linear measurements for Dmin, Dmax, and Length ranged from 1.5 to 2.4, 1.7 to 2.5, and 1.9 to 4.1 times larger than pathology-based dimensions, respectively, for the 3 experimental groups examined: acute (Non-Survival, 10-min ablation), subacute (3-Day), and chronic (30-Day) (). Since the ellipsoid volume formula is a multiplication of all linear dimensions, these differences translated into large discrepancies in volumes. The average CT ablation zone volume for the acute, subacute, and chronic groups was 4.9, 5.9, and 21.8 times greater, respectively, than volume measurements obtained macroscopically in pathology tissue slices ().
4. Discussion
4.1. Safety profile
The current study demonstrated the safety of CBCT-guided bronchoscopic MWA of peripheral healthy swine lung tissue by integrating the use of MONARCH, a RAB platform, and a flexible microwave ablation system (NEUWAVE FLEX). Clinical observations on the physical health of the animals, and radiological (CT and CBCT) and histopathological analysis of lungs and MWA sites were consistent with preliminary data from pilot studies. Ablation probes were navigated close to critical large organs and vascular structures including the heart, great vessels, esophagus, diaphragm, hilar vessels, and within large airways including the trachea and main bronchi, without any major procedural complication. RAB (MONARCH) coupled with intraprocedural CBCT to navigate to the planned ablation site and a tightly controlled delivery of microwave energy resulted in no damage to vital structures within the lung, or in the mediastinal vicinity (e.g., pericardium). The minor procedural incident that resulted from spontaneous proximal migration of the ablation probe triggered a device safety feature designed to mitigate the risk of inadvertent tissue damage. In this animal (ID: 7), the probe was in the right caudal lobe near the right dome of the diaphragm, where diaphragm motion is the greatest. Therefore, large diaphragmatic excursions and deformation of the right caudal lobe may have contributed to the proximal migration of the ablation probe. The NEUWAVE FLEX System is equipped with multiple safety features including a continuous reflected power measurement and threshold to stop energy delivery and prevent tissue injury. To further mitigate the risk of probe migration in the clinical setting, periodic fluoroscopic images during ablation are required to confirm probe placement. Bronchoscopic navigation through the trachea, right or left bronchi, and lobar bronchi was successful in all lung lobes; and the probes supported multiple use in a single procedural session, one ablation in subacute and chronic animals, and four ablations in acute swine. All ablations in subacute and chronic animals were performed at 100 W for 10 min, a treatment duration that has been reported to exhibit high reproducibility [Citation36].
Except for a single major complication in a 30-Day animal with a lower respiratory tract infection (ID: 15, SIR grade C), no major AEs were observed in any animal immediately after the procedure was completed, or during the postprocedural observation periods of 3 and 30 days. Thus, the peripheral bronchoscopic MWA procedure was safe and well tolerated by the 17 swine included in the study. Taken together, the low incidence of AEs documented for RAB and its improved ability to reach peripheral lung regions [Citation12–15], and the positive safety profile of MWA reported in the present study, including the absence of pleural complications typically associated with percutaneously delivered microwave energy, underlines the clinical significance of RAB MWA as a novel approach that combines powerful emerging technologies to safely treat pulmonary lesions.
4.2. Volumetric measurements
Increasing microwave ablation duration in four different lung lobes in a single animal increased ablation volumes in a time-dependent manner. The sequence of events triggered by the delivery of MWA energy includes cell death by coagulative necrosis, an injury-driven inflammatory response, and a tissue remodeling phase with the removal of cellular debris followed by the deposition of extracellular matrix proteins and fibrosis [Citation1,Citation35,Citation37]. Therefore, lesion size at the MWA site was commensurate to the energy delivered and the pathological changes associated with ablation lesion formation and healing.
A conceptual model representing the underlying post-ablation pathobiology of the MWA site for the 3 timepoints included in the study is shown in . The model depicts dimension data and aspect ratios proportional to the original measurements. The immediate acute effects of MWA include a core of coagulative necrosis and thermally fixed tissue surrounded by edema of tissue adjacent to the ablation zone (, left panel). As the zone continues to enlarge in the first few days following ablation, the development of perilesional edema, inflammation, and hemorrhage continues (, central panel). The full extent of coagulative necrosis in the ablation site core may occur days after the delivery of microwave energy. Histopathological analysis of the chronic 30-Day follow-up lesions consistently showed inflammation and a granulomatous lymphocytic infiltrate surrounding the ablation zone (), also depicted in the working model of the ablation site (, right panel).
Figure 7. Schematic representation of the MWA site as determined from GGO areas in CT sections and TTC-stained areas in gross pathology slices. Ablation settings were identical for the 3 experimental groups shown (100 W, 10 min). Measurements for the Non-Survival group correspond to Day 0, whereas those for the day-3 and day-30 groups were obtained on Day 3 and Day 30, respectively. Ablation volume was determined with the same ellipsoidal formula for the CT- and Pathology-based sets of measurements. MWA zones were radiologically defined in CT scans as homogeneous GGO areas, whereas gross and histopathological analysis of the MWA site distinguished several concentric areas. A central thermal fixation zone surrounded by an area of coagulative necrosis was observed in formalin-fixed tissue slices in the MWA sites of all groups. These regions, represented by A in the left and central panels, were surrounded by edema in the ablation sites of Non-Survival animals (B on the left panel), and by a larger area of edema, inflammation, and hemorrhage in the ablation sites of 3-Day animals (B on the middle panel). Edema and inflammation were less marked in the MWA sites of 30-Day animals; however, a granulomatous infiltrate adjacent to the area of edema and inflammation (C on the right panel) surrounding the MWA site was observed in all chronic swine. In the swine model used in the present study, areas labeled B in the Non-Survival and 3-Day groups, and B and C in the 30-Day group may have contributed to an overestimation of the GGO areas and thus the ablation zone in CT images of the MWA sites. The model’s schematics shown are scale drawings that kept the aspect ratio of the original CT- and pathology-based measurements (Length and Dmax). Data are expressed as mean ± SD. CT: computed tomography, Dmax, maximum diameter, GGO: ground-glass opacity, Vol: volume.

MWA of lung parenchyma triggers an asynchronous set of cellular and molecular responses occurring over several days post-ablation (e.g., cell death, cell proliferation, synthesis and reabsorption of extracellular matrix proteins). As a result, the size of the ablation site varied widely between animals in the 3-Day group (), a timepoint when a myriad of cellular repair mechanisms is at play. Post-ablation day 3 showed higher CT-based volumetric average values compared to day 30, consistent with involution of the ablation site at 30 days following resolution of the acute inflammatory response, myofibroblast proliferation, and scar tissue formation as reported in a myocardial microwave ablation model [Citation38].
In our study, CT-based measurements were significantly larger than gross pathology determinations (; ), in agreement with previous thermal ablation reports of overestimation of gross pathology ablation dimensions by CT and CBCT [Citation39,Citation40]. The disproportion between CT and gross pathology measurements may be accounted for by several factors such as the inclusion in the CT-based measurements of tissues adjacent to the ablation zone that may appear radiologically similar in GGOs but were not part of the ablation zone when examined by gross pathology. Furthermore, while measurements in CT scans reflect MWA site volumes in inflated lungs, pathology measurements were conducted in partially deflated, formalin-fixed lungs. Tissue dimensions are altered during processing for morphometric [Citation41,Citation42] or stereological analysis of lungs [Citation43]. Formalin fixation causes shrinkage of normal and tumor tissue and may even affect accurate staging of NSCLC tumors [Citation44].
In the current study, gross pathology measurements of acute, subacute, and chronic lesions were restricted to the MWA’s core of thermal fixation and coagulative necrosis (), whereas CT-based volume determinations included the edematous, inflammatory rim peripheral to the ablation core that results from MWA () since no clear boundary between the two was observed in GGO areas, as previously reported [Citation2]. An RFA study in swine has previously reported that differences between the maximum diameter measured on CT images and that measured macroscopically in pathology slices became larger as the follow-up examinations progressed [Citation40]. The authors postulated that obstructive pneumonitis found in the periphery of ablated areas of swine 4 weeks post-ablation was included as part of the ablated lesion on CT images [Citation40]. In our study, differences between CT and pathology measurements also became larger in the chronic, 30-Day group, compared to the subacute and acute groups. Thus, the granulomatous inflammation observed in the periphery of the ablation zone of the 30-Day swine in the current study may have been included in the GGO areas measured on CT images. Further support for this premise is provided by clinical studies showing that GGO areas in CT scans of post-ablation tumors are larger than prior to ablation because in CT images the ablation zone and peripheral layers of inflammation and granulation tissue are recognized as part of the ablated lesion [Citation45,Citation46].
In summary, we postulate that CT-based measurements overestimated the ablation zone due primarily to the inclusion of areas of edema in acute lesions (Non-Survival group), edema, inflammation, and hemorrhage in subacute lesions (3-Day group), and inflammation and a granulomatous lymphocytic infiltrate in chronic lesions (30-Day group) (). In addition, tissue fixation and volumetric determinations in partially deflated lungs may have contributed to the discrepancies by underestimating the pathology-based volumes of the MWA treatment site. Nonetheless, similar magnitude of changes in MWA site volumes was observed with both CT- and pathology-based methods, and both techniques documented a volumetric involution of the MWA site at 30 days as reported in preclinical and clinical studies [Citation35,Citation47,Citation48].
4.3. Cavitation
Post-ablation voids at the treatment sites are referred to as ‘cavitations’ in the MWA, RFA and cryoablation literature [Citation49–51]. Healthy, adult lung is a quiescent organ. However, lung tissue possesses the ability to repair, regenerate and remodel in response to injury [Citation52,Citation53]. Despite these remarkable abilities, cavitation formation may reflect the limited regenerative capacity of lung tissue to replace thermally ablated, necrotic tissue with healthy pulmonary tissue. A total of five ablation sites in the subacute (3-Day) and chronic (30-Day) groups exhibited central cavities. MWA treatment sites of Non-Survival (acute) animals showed no signs of cavitation because the cellular and tissue repair mechanisms that remove the necrotic core were not yet activated. The incidence of cavitation varies widely between published animal (10%) [Citation24] and clinical (43%) [Citation49–51,Citation54] studies, and it has been reported to reduce in size without additional intervention in normal ablated animal tissue [Citation24]. Clinical data suggests that cavitation incidence is higher in patients with primary lung cancer, a lesion near the chest wall, or pulmonary emphysema [Citation50]. However, most cavitations in the ablation treatment site resolve within 12 months [Citation51], and cavitations that develop after MWA of lung malignancies have been associated with improved survival rates [Citation49].
4.4. Ablation site inflammation and thrombosis
Mild to moderate alveolar edema was common in ablation sites of the three experimental groups, whereas varying degrees of inflammation were observed in 3- and 30-Day MWA sites. The primary inflammatory cell types involved in the subacute, 3-Day group were neutrophils and monocytes/macrophages, whereas lymphocytes represented a prominent cell type in 30-Day ablation sites. The presence of lymphocytic foci within the organizing granulomas at the ablation treatment site of 30-Day animals suggests that a model-specific chronic immune response may have been triggered by thermal ablation. The local tissue-destruction capacity of microwave energy and the subsequent host immune response may be similar to the effects of RFA on the immune system, including the release of proinflammatory cytokines and chemokines to the general circulation [Citation55]. In our study, it is uncertain whether the pulmonary granulomatous lesions in and around the MWA sites of 30-Day swine were exacerbations of a preexisting asymptomatic respiratory infection or the result of infections triggered by the RAB MWA procedure with subsequent involvement of secondary pathogens.
Bacterial colonies (cocci) absent in lungs of Non-Survival animals albeit present in necrotic zones and cavities of 3-Day (3/6, 50%) and 30-Day swine (4/6, 67%) suggest that bacterial colonization of the MWA treatment site with opportunistic pathogens may have contributed to the secondary development of infectious lesions. Clinical human pulmonary infection rates after MWA are rather low, ranging from no infections [Citation56] to up to 7.5% [Citation57–60], highlighting the limitations of the animal model used in our study. Bacterial colonization of ablation sites and cavities may be the result of invasion by symbiotic microorganisms from the microbiome of the upper or lower respiratory tract trapped upon cavity formation [Citation61]. Once trapped within an avascular cavity, opportunistic pathogens proliferated in its inner surface, an auspicious microenvironment for bacterial attachment and growth. The good general health of most animals following thermal ablation, the normal white blood cell counts (CBC with differential) and blood gas analyses, and the lack of respiratory distress and pulmonary infection signs (e.g., coughing, rapid and shallow breathing) in most animals suggest that cavitation formation, bacterial colonization, and secondary pneumonic bacterial infections bounded by fibrotic tissue were restricted to focal parenchymal areas and had no clinical impact on ventilation and respiratory function.
Mild to moderate hemorrhagic areas in ablation sites and minimal to mild vascular thrombosis were nearly exclusively observed in ablation treatment sites of 3-Day animals. Thus, the transitory peaks of the inflammatory, hemorrhagic, and thrombotic responses occurred 3 days after RAB MWA, as reported after RFA of pulmonary malignancies and in animal models of wound healing [Citation62,Citation63]. Neither hemorrhage nor vascular thrombosis was observed in 30-Day ablation sites indicating that both vascular events triggered by thermal ablation were resolved at or before post-ablation day 30.
4.5. Study limitations
This preclinical GLP study was a safety evaluation of two medical devices and thus sample sizes were small (5–6 per group). While the use of swine is becoming a standard for testing of lung ablative therapies [Citation24–26], the model is not devoid of limitations. Despite the physiological, anatomical, immunological and genetic similarities between humans and swine [Citation22,Citation23], porcine lungs are smaller than their human counterparts, and lung collateral ventilation, a set of pathways that bypass alveoli and seem to modulate ventilation perfusion in a variety of pulmonary disease states, is limited in swine [Citation64,Citation65]. Navigating smaller porcine airways and ablating sites in smaller lungs without major procedural complications using RAB and flexible ablation probes designed for human airways highlights the safety of the devices used.
4.5.1. Pulmonary infections
The primary pathogen of enzootic pneumonia, a chronic respiratory disease in pigs, is M. hyopneumoniae [Citation66]. In our study, 3 animals were positive for M. hyopneumoniae and bacterial cultures from lung samples of a 3-Day animal (ID: 13) also tested positive for Streptococcus suis and Trueperella pyogenes. Thus, the model propensity to endemic infectious diseases and potential exacerbation of secondary infections, and the potential need for extended use of prophylactic antibiotics should be taken into consideration when using this animal model. Further studies are warranted to investigate the need of prophylactic antibiotic therapy in the clinical setting before performing bronchoscopic MWA.
4.5.2. Ablation zone dimensions
Discrepancies in ablation dimensions between CT- and macroscopy-based measurements reflect the limitations of the animal model (perilesional tissue responses) and CT to discriminate between the vastly different underlying pathobiology of acute, subacute, and chronic post-ablation tissue responses. Additional CT imaging and pathology assessment tools should be explored and developed to define the ablation zone more accurately and draw closer correlative measures between clinical and gross pathology observations.
4.5.3. Technical success (TS) and technique efficacy (TE)
TS and TE are determined in CT scans taken immediately after the procedure and 30 days later, respectively. TS and TE determine how close post-ablation measurements are to a set of pre-ablation tumor dimensions. Therefore, preprocedural ablation treatment site margins cannot be obtained in the absence of a target tumor. To address this limitation, lung tumor-mimic models in swine have been used to evaluate the ablation zone, however, the model’s reproducibility is limited, and the physical and biological properties of the material mimicking lung lesions may be different from those exhibited by tumors [Citation67].
5. Conclusion
Single and multiple RAB MWA procedures were safely performed with the NEUWAVE FLEX and MONARCH devices. The safety profile of both devices used for RAB MWA supports their use in clinical trials to treat patients with oligometastatic disease or primary NSCLC.
Supplemental Material
Download PDF (214.3 KB)Acknowledgements
The authors thank Berit DelGuercio for support on tissue processing for histopathological analyses, and Vivienne Mendoza for administrative and document management assistance.
Disclosure statement
The following authors: HDL, DJ, TM, JPM, and GC are employees of Ethicon, Inc.; KR, and LM are employees of NeuWave Medical, Inc. both Johnson & Johnson MedTech companies. PL, SP, and DW are consultants for Ethicon, Inc.
Data availability statement
The authors confirm that the data supporting the findings of this study are available within the article and its supplementary materials.
Additional information
Funding
References
- Ahmed M, Brace CL, Lee FT, Jr., et al. Principles of and advances in percutaneous ablation. Radiology. 2011;258(2):351–369.
- Brace CL, Hinshaw JL, Laeseke PF, et al. Pulmonary thermal ablation: comparison of radiofrequency and microwave devices by using gross pathologic and CT findings in a swine model. Radiology. 2009;251(3):705–711.
- Lu DS, Raman SS, Vodopich DJ, et al. Effect of vessel size on creation of hepatic radiofrequency lesions in pigs: assessment of the "heat sink" effect. AJR Am J Roentgenol. 2002;178(1):47–51.
- Yu NC, Raman SS, Kim YJ, et al. Microwave liver ablation: influence of hepatic vein size on heat-sink effect in a porcine model. J Vasc Interv Radiol. 2008;19(7):1087–1092.
- Bhardwaj N, Strickland AD, Ahmad F, et al. A comparative histological evaluation of the ablations produced by microwave, cryotherapy and radiofrequency in the liver. Pathology. 2009;41(2):168–172.
- Planché O, Teriitehau C, Boudabous S, et al. In vivo evaluation of lung microwave ablation in a porcine tumor mimic model. Cardiovasc Intervent Radiol. 2013;36(1):221–228.
- Durick NA, Laeseke PF, Broderick LS, et al. Microwave ablation with triaxial antennas tuned for lung: results in an in vivo porcine model. Radiology. 2008;247(1):80–87.
- Hiraki T, Gobara H, Fujiwara H, et al. Lung cancer ablation: complications. Semin Intervent Radiol. 2013;30(2):169–175.
- Izaaryene J, Cohen F, Souteyrand P, et al. Pathological effects of lung radiofrequency ablation that contribute to pneumothorax, using a porcine model. Int J Hyperthermia. 2017;33(7):713–716.
- Streitparth T, Schumacher D, Damm R, et al. Percutaneous radiofrequency ablation in the treatment of pulmonary malignancies: efficacy, safety and predictive factors. Oncotarget. 2018;9(14):11722–11733.
- Huang G, Yang X, Li W, et al. A feasibility and safety study of computed tomography-guided percutaneous microwave ablation: a novel therapy for multiple synchronous ground-glass opacities of the lung. Int J Hyperthermia. 2020;37(1):414–422.
- Chen AC, Gillespie CT. Robotic endoscopic airway challenge: REACH assessment. Ann Thorac Surg. 2018;106(1):293–297.
- Chen AC, Pastis NJ, Machuzak MS, et al. Accuracy of a robotic endoscopic system in cadaver models with simulated tumor targets: ACCESS study. Respiration. 2020;99(1):56–61.
- Rojas-Solano JR, Ugalde-Gamboa L, Machuzak M. Robotic bronchoscopy for diagnosis of suspected lung cancer: a feasibility study. J Bronchology Interv Pulmonol. 2018;25(3):168–175.
- Chen AC, Pastis NJ, Jr., Mahajan AK, et al. Robotic bronchoscopy for peripheral pulmonary lesions: a multicenter pilot and feasibility study (BENEFIT). Chest. 2021;159(2):845–852.
- Lau K, Lau R, Baranowski R, et al. Late-breaking abstract – bronchoscopic microwave ablation of peripheral lung tumors. European Respiratory Society International Congress 2021. 2021; ERS, Virtual.
- Periyasamy S, Wilson D, Cipolla G, et al. Assessement of transbronchial microwave ablation zone using mobile C-arm CBCT: in vivo porcine model results. SIO 2021 international conference, society for integrative oncology, Baltimore, MD, 2021.
- Chan JWY, Lau RWH, Ngai JCL, et al. Transbronchial microwave ablation of lung nodules with electromagnetic navigation bronchoscopy guidance-a novel technique and initial experience with 30 cases. Transl Lung Cancer Res. 2021;10(4):1608–1622.
- Ghosn M, Elsakka AS, Ridouani F, et al. Augmented fluoroscopy guided transbronchial pulmonary microwave ablation using a steerable sheath. Transl Lung Cancer Res. 2022;11(2):150–164.
- Auris_Health_Inc. MONARCH platform. User manual. Redwood City (CA): Auris Health, Inc.; 2020.
- NeuWave_Medical_Inc. NEUWAVE FLEX MC microwave ablation system. User reference manual. Madison (WI): NeuWave Medical, Inc.; 2021.
- Walters EM, Prather RS. Advancing swine models for human health and diseases. Mo Med. 2013;110(3):212–215.
- Meurens F, Summerfield A, Nauwynck H, et al. The pig: a model for human infectious diseases. Trends Microbiol. 2012;20(1):50–57.
- Kodama H, Ueshima E, Gao S, et al. High power microwave ablation of normal swine lung: impact of duration of energy delivery on adverse event and heat sink effects. Int J Hyperthermia. 2018;34(8):1186–1193.
- Banovac F, Cheng P, Campos-Nanez E, et al. Radiofrequency ablation of lung tumors in swine assisted by a navigation device with preprocedural volumetric planning. J Vasc Interv Radiol. 2010;21(1):122–129.
- Kohno M, Hashimoto R, Oiwa K, et al. Initial experience with transbronchial cryoablation as a novel local treatment for malignant peripheral lung lesions. BMJ Open Resp Res. 2018;5(1):e000315.
- Judge EP, Hughes JM, Egan JJ, et al. Anatomy and bronchoscopy of the porcine lung. A model for translational respiratory medicine. Am J Respir Cell Mol Biol. 2014;51(3):334–343.
- MacDonald MH, Wang AY, Clymer JW, et al. An in vivo comparison of the efficacy of hemostatic powders, using two porcine bleeding models. MDER. 2017;10:273–279.
- MacDonald MH, Tasse L, Wang D, et al. Evaluation of the hemostatic efficacy of two powdered topical absorbable hemostats using a porcine liver abrasion model of mild to moderate bleeding. J Invest Surg. 2020;34(11):1198–1206.
- National_Research_Council. Guide for the care and use of laboratory animals. 8th ed. Washington (DC): The National Academies Press; 2011.
- Yokoyama K, Nakagawa H, Shah DC, et al. Novel contact force sensor incorporated in irrigated radiofrequency ablation catheter predicts lesion size and incidence of steam pop and thrombus. Circ Arrhythm Electrophysiol. 2008;1(5):354–362.
- Nakagawa H, Ikeda A, Sharma T, et al. Comparison of in vivo tissue temperature profile and lesion geometry for radiofrequency ablation with high power-short duration and moderate Power-Moderate duration: Effects of thermal latency and contact force on lesion formation. Circ Arrhythm Electrophysiol. 2021;14(7):e009899.
- Determination of Safety and Effectiveness. FDA 21 CFR, Chapter I, Subchapter H, part 860.7 (d). Title 21 was last amended 11/25/2022. In: n.A.a.R. Administration (Ed.). 2021.
- Sacks D, McClenny TE, Cardella JF, et al. Society of interventional radiology clinical practice guidelines. J Vasc Interv Radiol. 2003;14(9):S199–S202.
- Ahmed M, Solbiati L, Brace CL, et al. Image-guided tumor ablation: standardization of terminology and reporting criteria–a 10-year update. Radiology. 2014;273(1):241–260.
- Habert P, Di Bisceglie M, Hak JF, et al. Percutaneous lung and liver CT-guided ablation on swine model using microwave ablation to determine ablation size for clinical practice. Int J Hyperthermia. 2021;38(1):1140–1148.
- Simon CJ, Dupuy DE, Mayo-Smith WW. Microwave ablation: principles and applications. Radiographics. 2005;25(suppl_1):S69–S83.
- de Gouveia RH, Melo J, Santiago T, et al. Comparison of the healing mechanisms of myocardial lesions induced by dry radiofrequency and microwave epicardial ablation. Pacing Clin Electrophysiol. 2006;29(3):278–282.
- Meram E, Longhurst C, Brace CL, et al. Comparison of conventional and cone-beam CT for monitoring and assessing pulmonary microwave ablation in a porcine model. J Vasc Interv Radiol. 2018;29(10):1447–1454.
- Yamamoto A, Nakamura K, Matsuoka T, et al. Radiofrequency ablation in a porcine lung model: correlation between CT and histopathologic findings. AJR Am J Roentgenol. 2005;185(5):1299–1306.
- Lum H, Mitzner W. Effects of 10% formalin fixation on fixed lung volume and lung tissue shrinkage. A comparison of eleven laboratory species. Am Rev Respir Dis. 1985;132(5):1078–1083.
- Schneider JP, Ochs M. Alterations of mouse lung tissue dimensions during processing for morphometry: a comparison of methods. Am J Physiol Lung Cell Mol Physiol. 2014;306(4):L341–L350.
- Saad M, Ruwanpura SM. Tissue processing for stereological analyses of lung structure in chronic obstructive pulmonary disease. Methods Mol Biol. 2018;1725:155–162.
- Park HS, Lee S, Haam S, et al. Effect of formalin fixation and tumour size in small-sized non-small-cell lung cancer: a prospective, single-centre study. Histopathology. 2017;71(3):437–445.
- Steinke K, King J, Glenn D, et al. Radiologic appearance and complications of percutaneous computed tomography-guided radiofrequency-ablated pulmonary metastases from colorectal carcinoma. J Comput Assist Tomogr. 2003;27(5):750–757.
- Yasui K, Kanazawa S, Sano Y, et al. Thoracic tumors treated with CT-guided radiofrequency ablation: initial experience. Radiology. 2004;231(3):850–857.
- Park CS, Liu C, Hall SK, et al. A thermoelastic deformation model of tissue contraction during thermal ablation. Int J Hyperthermia. 2018;34(3):221–228.
- Kodama H, Ueshima E, Howk K, et al. Temporal evaluation of the microwave ablation zone and comparison of CT and gross sizes during the first month post-ablation in swine lung. Diagn Interv Imaging. 2019;100(5):279–285.
- Wolf FJ, Grand DJ, Machan JT, et al. Microwave ablation of lung malignancies: effectiveness, CT findings, and safety in 50 patients. Radiology. 2008;247(3):871–879.
- Okuma T, Matsuoka T, Yamamoto A, et al. Factors contributing to cavitation after CT-guided percutaneous radiofrequency ablation for lung tumors. J Vasc Interv Radiol. 2007;18(3):399–404.
- Chaudhry A, Grechushkin V, Hoshmand M, et al. Characteristic CT findings after percutaneous cryoablation treatment of malignant lung nodules. Medicine. 2015;94(42):e1672.
- Beers MF, Morrisey EE. The three R's of lung health and disease: repair, remodeling, and regeneration. J. Clin. Invest. 2011;121(6):2065–2073.
- Kotton DN, Morrisey EE. Lung regeneration: mechanisms, applications and emerging stem cell populations. Nat Med. 2014;20(8):822–832.
- Huang G, Ye X, Yang X, et al. Invasive pulmonary aspergillosis secondary to microwave ablation: a multicenter retrospective study. Int J Hyperthermia. 2018;35(1):71–78.
- Fietta AM, Morosini M, Passadore I, et al. Systemic inflammatory response and downmodulation of peripheral CD25 + Foxp3+ T-regulatory cells in patients undergoing radiofrequency thermal ablation for lung cancer. Hum Immunol. 2009;70(7):477–486.
- Egashira Y, Singh S, Bandula S, et al. Percutaneous high-energy microwave ablation for the treatment of pulmonary tumors: a retrospective single-center experience. J Vasc Interv Radiol. 2016;27(4):474–479.
- Liu J, Huang W, Wu Z, et al. The application of computed tomography-guided percutaneous coaxial biopsy combined with microwave ablation for pulmonary tumors. J Cancer Res Ther. 2019;15(4):760–765.
- Han X, Yang X, Huang G, et al. Safety and clinical outcomes of computed tomography-guided percutaneous microwave ablation in patients aged 80 years and older with early-stage non-small cell lung cancer: a multicenter retrospective study. Thorac Cancer. 2019;10(12):2236–2242.
- Healey TT, March BT, Baird G, et al. Microwave ablation for lung neoplasms: a retrospective analysis of long-term results. J Vasc Interv Radiol. 2017;28(2):206–211.
- Zhou X, Li H, Qiao Q, et al. CT-guided percutaneous minimally invasive radiofrequency ablation for the relief of cancer related pain from metastatic non-small cell lung cancer patients: a retrospective study. Ann Palliat Med. 2021;10(2):1494–1502.
- Santacroce L, Charitos IA, Ballini A, et al. The human respiratory system and its microbiome at a glimpse. Biology. 2020;9(10):318.
- Clasen S, Krober SM, Kosan B, et al. Pathomorphologic evaluation of pulmonary radiofrequency ablation: proof of cell death is characterized by DNA fragmentation and apoptotic bodies. Cancer. 2008;113(11):3121–3129.
- Landén NX, Li D, Ståhle M. Transition from inflammation to proliferation: a critical step during wound healing. Cell Mol Life Sci. 2016;73(20):3861–3885.
- Hedenstierna G, Hammond M, Mathieu-Costello O, et al. Functional lung unit in the pig. Respir Physiol. 2000;120(2):139–149.
- Terry PB, Traystman RJ. The clinical significance of collateral ventilation. Annals ATS. 2016;13(12):2251–2257.
- Maes D, Segales J, Meyns T, et al. Control of Mycoplasma hyopneumoniae infections in pigs. Vet Microbiol. 2008;126(4):297–309.
- Prud’homme C, Teriitehau C, Adam J, et al. Lung microwave ablation - an in vivo swine tumor model experiment to evaluate ablation zones. Int J Hyperthermia. 2020;37(1):879–886.