Abstract
Background
Data on outcome for patients in different body mass index (BMI) categories in young adults with acute lymphoblastic leukemia (ALL) are scarce. We explored survival and toxicities in different BMI categories in young adults with ALL.
Material and methods
Patients aged 18–45 years, diagnosed with ALL between July 2008 and June 2022 in the Nordic countries, Estonia, or Lithuania, and treated according to the NOPHO ALL2008 protocol, were retrospectively enrolled and classified into different BMI categories. Endpoints were overall survival (OS), event-free survival (EFS) and cumulative incidence of relapse as well as incidence rate ratio (IRR) of severe predefined toxic events, and treatment delays.
Results
The group comprised 416 patients, of whom 234 (56%) were stratified to non-high-risk (non-HR) treatment. In the non-HR group, patients with severe obesity, BMI ≥35 kg/m2 had worse EFS due to relapses but there was no effect on toxicity or treatment delays compared with the healthy-weight patients. There was no association between BMI category and OS, overall toxicity, or treatment delays in the patients with high-risk treatment.
Conclusion
Severe obesity is associated with worse EFS in young adults treated according to the non-HR arms of the NOPHO ALL2008 protocol. Poorer outcome is explained with a higher risk of relapse, possibly due to under treatment, and not caused by excess therapy-related mortality.
Background
As the prevalence of overweight and obesity increases, patients with unhealthy body mass index (BMI) are more frequently encountered in hematology and oncology. Obesity has been associated both with a generally increased risk of cancer, an increased incidence of acute lymphoblastic leukemia (ALL), as well as increased mortality after diagnosis of ALL [Citation1–4]. Obese children with ALL have lower overall survival (OS) and an increased risk of relapse [Citation5–10]. In two pediatric studies, obesity was associated with increased risk of relapse and decreased event-free survival (EFS), but only in children older than 10 years, suggesting higher vulnerability with increasing age [Citation7,Citation10]. In adults, studies of obesity and survival or relapse are scarce but suggest that obese patients have poorer outcomes [Citation11,Citation12].
With improved survival in ALL, focus has shifted towards therapy-related toxicity to decrease morbidity and mortality. Severe toxicity often leads to therapy interruptions and protocol deviations, which may compromise treatment outcome [Citation13,Citation14]. Obesity might lead to under- or overtreatment, for example due to off-protocol dose-reductions in patients with extreme body weight, pharmacokinetics differences, and increased chemotherapy resistance. High BMI has been associated with a higher risk of hepatic toxicity, pancreatitis, hyperglycemia, hypertonia, hyperlipidemia, and neutropenic fever, as well as thromboembolism and osteonecrosis in older children [Citation7,Citation15–23]. Studies on the impact of unhealthy BMI on risk of severe treatment-related complications in young adults with ALL are limited. Stock et al. observed that hepatic toxicity, hyperglycemia, and thrombotic toxicities during induction were more frequent in obese compared to non-obese young adults with ALL [Citation12]. Furthermore, in adults undergoing allogeneic hematopoietic stem cell transplantation (HSCT), underweight has been associated with poorer survival [Citation24,Citation25].
We previously showed that obese pre-adolescents and adolescents (10– <18 years) with ALL have a higher relapse risk and worse outcome when treated with the non-high-risk (non-HR) NOPHO (Nordic Society of Paediatric Haematology and Oncology) ALL2008 protocol than their non-obese counterparts [Citation10,Citation20]. The same treatment protocol has been used for young adults. The purpose of this study was to explore the impact of obesity on outcome and the occurrence of toxicity in young adults with ALL treated according to the Nordic/Baltic ALL treatment protocol, NOPHO ALL2008.
Material and methods
Study group
Patients aged 18–45 years, diagnosed with Philadelphia-negative B cell-precursor (BCP) or T-cell ALL between July 2008 and June 2020 in the Nordic (Sweden, Norway, Denmark, Finland) and Baltic (Estonia and Lithuania) countries and treated with the pediatric NOPHO ALL2008 protocol, were identified from the prospective NOPHO ALL2008 registry and retrospectively included in this multicenter registry cohort study. Follow-up ended in March 2023. Patients with Down syndrome (n = 3), pre-treatment (n = 5) and previous cancer (n = 1) were excluded, as were patients with missing registry data on BMI (n = 16), outcome (n = 2), or treatment (n = 6) (Supplementary Figure 1). The patients were classified into five different BMI categories: underweight (<18.5 kg/m2); healthy weight (normal weight; 18.5– <25 kg/m2); overweight (25– <30 kg/m2); obese (class I obesity; 30– <35 kg/m2); and severely obese (class II obesity; ≥35 kg/m2) [Citation26].
Treatment protocol
The treatment protocol has been described previously [Citation27–29]. Patients were assigned to standard risk (SR), intermediate risk (IR), or high risk (HR) treatment, based on leukemia characteristics at diagnosis and minimal residual disease (MRD) in bone marrow during or after induction therapy (day 29). Non-HR (SR and IR) patients were defined as those with BCP ALL with white blood cell count > 100 × 10^9/L at diagnosis or T-cell immunophenotype and <25% blasts at day 15 and MRD <0.1% at day 29, or any patient with MRD <5% on day 29 and <0.1% day 79 without unfavorable cytogenetics (11q23 (KMT2A) rearrangement and/or hypodiploidy). HR patients were defined as those with BCP ALL with white blood cell count >100 × 10^9/L at diagnosis or T-cell immunophenotype and MRD >0.1% at day 29, or any patient with poor response (MRD >25% on treatment day 15, >5% day 29 or ≥0.1% day 79) or unfavorable cytogenetics (11q23 (KMT2A) rearrangement and/or hypodiploidy). HSCT was indicated by poor response (day 29 MRD ≥5%, or day 79 or post-block B1 ≥ 0.1%). The patients received the same treatment as pediatric patients (<18 years). Shortly, three-drug four-week induction treatment for patients with SR and IR ALL included steroids (dexamethasone or prednisolone), weekly vincristine, intrathecal methotrexate x4 and daunorubicin day 1 and 21. Patients then proceeded to consolidation (based on oral mercaptopurine, PEG-asparaginase, and high-dose methotrexate) followed by one (SR) or two (IR) delayed intensifications in the post-induction ALL therapy. Patients with HR-ALL without HSCT received seven to nine intensive chemotherapy blocks after induction before delayed intensification. Oral mercaptopurine/methotrexate maintenance therapy was continued to 2.5 years from diagnosis. For dosing of all systemic chemotherapy, body surface area (BSA) was used except for a capping dose of 2 mg for vincristine. In the protocol guidelines for dose management of severely obese patients, dose adjustments were not recommended.
Toxicity registration
The NOPHO ALL2008 registry included weight and height at diagnosis, information on treatment response (MRD), start date of each treatment phase, and reports on predefined severe adverse events (SAEs) prospectively [Citation28]. Toxicities were registered during and after induction (day 29) and thereafter every third month for all patients, as described previously and defined in the legend of [Citation20]. As the intensive HR block treatment with or without HSCT differs significantly from the non-HR treatment, the HR-group was analyzed separately.
Table 1. Incidence rate ratio (IRR) of registered severe adverse events across the BMI categories in non-high-risk patients.
Statistical analyses
Endpoints included EFS, cumulative incidence of relapse, OS, and treatment-related mortality (TRM) in unhealthy BMI categories compared to healthy-weight patients. Survival analysis for the HR patients were performed both together with the non-HR patients and separately. Additional outcomes included incidence rate ratio (IRR) of the measured SAEs, and treatment delays, in the different unhealthy BMI categories compared with the healthy-weight group. Treatment delays were measured as time until start of the first maintenance in non-HR patients and until start of treatment block 4 in HR-patients.
Multivariable Cox proportional hazards regression models adjusted for age at diagnosis, sex, and risk group were used to compare relapse, EFS, TRM, and OS in different BMI categories. Adjustment for HSCT as a time-dependent co-variable was made in patients who underwent HSCT in first complete remission. EFS was calculated from the time of diagnosis to relapse, secondary malignancy, death, or end of follow-up for patients without events. The Kaplan-Meier method was used for estimation of EFS, OS, and the probability of cumulative incidence of relapse, in different BMI categories. Cumulative incidence of relapse was calculated and visualized with secondary malignancy and death as competing risks [Citation30].
Poisson regression models were used in the IRR toxicity analyses, offsetting for the logarithm of time at risk for toxicity, and adjusted for age, sex, and risk group. All patients were censored at the end of therapy, time of relapse, loss to follow-up or death of any cause, whichever came first. Observed differences in proportions between groups with categorical variables were tested using Fisher’s exact test.
Treatment delay was analyzed as the correlation coefficient and p-value between BMI as a continuous variable and days of delay in each treatment phase. In categorical BMI analyses, the median treatment delay for each unhealthy BMI category was compared with the delay in the healthy weight BMI category. A 2-sided p-value <0.05 was considered statistically significant in all comparisons. Statistical analyses were performed using IBM SPSS Statistics Version 28.0 (SPSS Inc., Illinois, USA) and R statistical software version 4.2.1 (R Foundation for Statistical Computing, Vienna, Austria).
The NOPHO ALL2008 protocol was approved by the relevant Ethical Review Board of each country and informed consent was obtained according to the Declaration of Helsinki. Separate ethical approval was required in Sweden for the use of registered data in this study (registration numbers 2018/1888-31, 2020-01665, 2022-07011-02, and 2023-01141-02). The STROBE cohort reporting guidelines were used in the study [Citation31].
Results
Patient characteristics
In total, 416 patients were included in the cohort, of whom 234 were non-HR and 182 were HR patients. Median age at diagnosis was 25.9 years in the non-HR and 28.1 years in the HR group. Median follow-up time for patients in complete remission was 5.9 years (range 0.1 − 13.5 years). Patient characteristics are summarized in . There were no significant differences in BMI categories between the countries. The proportion of obese/severely obese did not differ significantly between non-HR and HR groups. Five patients died during induction (3/243 with healthy weight and 2/95 with overweight).
Table 2. Baseline patient characteristics for the overall study population and stratified by body mass index category.
Survival and events
Non-HR
Almost one-fourth (23.9%; 56/234) of the non-HR patients relapsed and 16.2% (38/234) died. The proportion of relapses in the severely obese non-HR patients was 50% (6/12) compared to 21.6% (30/139) in healthy-weight patients (p-value = 0.04). The severely obese patients had an inferior EFS (hazard ratio 2.60 [95% confidence interval, CI, 1.08–6.27], p-value = 0.03) compared to the healthy weight group due to the high risk of relapse (hazard ratio relapse 2.98 [95% CI 1.22–7.27], p-value = 0.02). No association between EFS or relapse was observed in the other BMI categories compared to the healthy weight group. TRM and OS did not significantly differ in any of the BMI categories ( and ). When analyzing obese and severely obese as one group, they had a tendency for worse OS (hazard ratio 2.12 [95% CI 0.98–4.56], p-value = 0.55), but not for worse EFS, relapse, or TRM, compared to the healthy weight group.
Figure 1. Kaplan Meier curves illustrating the effect of body mass index (BMI) classification on overall survival (A), event-free survival (B), and cumulative incidence of relapse (C) in young adults stratified to standard or intermediate risk protocol. For the assessment of cumulative incidence of relapse, death was treated as a competing risk.
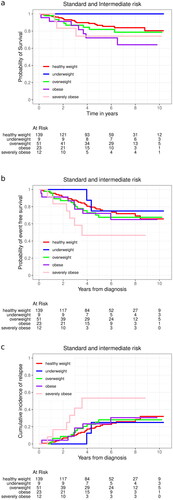
Table 3. Multivariate Cox regression hazard ratios for relapse, event-free survival, overall survival and treatment related death according to body mass index categories.
HR-chemotherapy and HSCT
In the HR-chemotherapy group, 23/81 patients (28.4%) relapsed and 32/81 (39.5%) died. There were no associations between the BMI group and OS, EFS, TRM, or cumulative incidence of relapse in the HR patients ( and Supplementary Figure 2). This was also true when separately analyzing patients stratified to the HR-chemotherapy and HR-HSCT arms, and patients who actually underwent HSCT.
Severe acute toxicity
To explore whether acute toxicity could have contributed to the adverse EFS in the severely obese, we analyzed the risk for predefined severe acute toxicities in the five BMI groups.
SAE in non-HR patients
Adverse events during treatment among the non-HR patients were common: 165/234 (70.5%) had one or more predefined SAE during treatment. The proportions of having one or more predefined SAE did not differ significantly between the BMI categories (underweight n = 8/9, 88.9%; healthy weight n = 98/139, 70.5%; overweight n = 35/51, 68.6%; obese n = 18/23, 78.3%; severely obese n = 6/12, 50%; p-value = 0.36). After adjusting for age, sex, and risk group, there were no significant differences in the IRR analyses of having one or more SAEs between healthy weight and the other BMI categories, nor a correlation between BMI as a continuous variable and number of patients with any SAE ().
When analyzing specific toxicities, there was a significantly increased risk of hyperlipidemia (IRR 3.39 [95% CI 1.20–9.47], p-value = 0.02) and hyperglycemia (3.43 [95% CI 1.06–11.12], p-value = 0.04) in the non-HR obese and a trend for increased liver toxicity (IRR 4.10 [95% CI 0.82–20.56], p = 0.09) in the severely obese patients compared to healthy weight patients (). Of note, there were no events of hyperlipidemia and hyperglycemia registered in the severely obese non-HR patients. When comparing linear associations between BMI and the IRR for different severe toxicities, besides hyperlipidemia and hyperglycemia, increasing BMI correlated with increasing IRR also for severe liver dysfunction. We did not observe an increased risk for asparaginase-associated toxicities (thrombosis, anaphylactic reaction, osteonecrosis, and pancreatitis) nor truncation of asparaginase in patients with high BMI. Underweight patients had significantly increased IRR of osteonecrosis (IRR 3.32; [95% CI 1.06–10.39], p-value = 0.04)) in the non-HR cohort, compared to healthy weight patients.
SAEs in HR patients
Adverse events during treatment were registered in 53.3% (97/182) of the HR patients. The proportions of having one or more predefined SAE did not differ significantly between the BMI categories (underweight n = 4/9, 44.4%; healthy weight n = 58/104, 55.8%; overweight n = 20/44, 45.5%; obese n = 8/16, 50.0%; severely obese n = 7/9, 77.8%; p-value = 0.44). There was no significant difference in IRR of one or more SAE during the HR ALL treatment after adjusting for age and sex, comparing the four under- and overweight BMI categories with healthy weight. Specific SAEs of special interest are presented in Supplementary Table 1.
Treatment delays
The non-HR patients had a median delay of 19 days (interquartile range, IQR, 25) from diagnosis until the start of first maintenance while the HR patients had a median delay to start of HR block 4 of 26 days (IQR 29 days). There were no significant differences in the median delay between the different treatment periods or the time from diagnosis to start of maintenance 1 (corresponding to day 134 (SR) and day 148 (IR)) or the median time to start of HR block 4 in the different BMI categories, nor was there any linear association between BMI and median delay/time.
Discussion
We studied the effect of unhealthy BMI on predefined treatment-related severe toxicities, treatment delays, and outcome of leukemia treatment to better understand the prognostic impact of body composition on ALL in young adults up to 45 years of age. We have previously shown that older obese children treated with the non-HR NOPHO ALL2008 protocol have a worse EFS and higher risk of relapse compared to children with healthy BMI [Citation10]. Since the same protocol was used up to the age of 45 years, we wanted to explore whether unhealthy BMI had a similarly negative impact on outcomes in older individuals treated according to the same protocol. We show that in line with older non-HR obese children, the severely obese non-HR young adults had a higher risk of relapse than their healthy weight counterparts. However, severe obesity was not associated with a higher risk of TRM, predefined SAEs, or treatment delays.
In a meta-analysis on obese adults with leukemia, Costello et al. showed an association between increased BMI (obesity) and increased mortality. However, no reason for the higher mortality could be identified [Citation1]. Wiedewuilt et al. compared obese adolescents and young adults (AYA) with non-obese patients, aged 16–39 years with ALL in first remission treated according to a pediatric protocol with or without HSCT. They found both a two-fold inferior disease-free survival (DFS) and OS, as well as a higher relapse rate, in obese compared to non-obese patients. The non-relapse mortality was also increased two-fold [Citation11]. The inferior prognosis in the obese group was observed both in patients receiving only chemotherapy as well as those who underwent HSCT. In a smaller study, Stock et al. also describe worse DFS in the obese AYA population as well as a tendency for worse survival [Citation12]. Another study showed inferior EFS in obese children and adolescents aged 1-20 years with HR ALL [Citation18]. In contrast, BMI had no impact on EFS or OS in HR patients in our cohort. The difference may be due to the very intensive HR therapy in the 2008 protocol potentially decreasing the effect of BMI categories. Only in non-HR patients with severe obesity could we observe a significantly decreased EFS, due to a higher risk of relapse. Yet, despite a three-fold increased risk of relapse in severely obese non-HR patients, we could not detect an association with inferior OS and obesity or severe obesity in young adults separately, although there was a tendency to inferior OS when obese and severely obese patients were analyzed together. Our cohort of severely obese patients is small, decreasing its impact on OS.
One possible explanation causing a higher risk of relapse in severely obese patients is treatment modification, resulting in undertreatment. Dose reductions in the obese and especially the severely obese young adults may be more common than in normal-weight patients due to fear of overtreatment. We explored the impact of BMI and the risk of SAEs separately in the non-HR group, where high BMI and risk of relapse were associated, and found no evidence of increased toxicity or TRM in obese or severely obese patients. The latest American Society of Clinical Oncology (ASCO) guidelines recommend full treatment doses in adult obese patients and there are very few reports on increased toxicity in obese compared to non-obese in general [Citation32]. Our study supports the ASCO guidelines on full-dose treatment in obese ALL patients to reduce the risk of relapse.
In our cohort, more than 15% of the non-HR patients were classified as obese or severely obese. In comparison, of the children treated according to the same protocol, 2.8% of all children and 4.3% of adolescents over 10 years were obese. Obesity is thus significantly more prevalent in young adult ALL patients. As weight gain is a gradual process, severely obese adults may have been obese already in adolescence. In this way, the findings may be connected. However, it is also possible that there is no causal relationship between obesity and the high relapse rate and the association with obesity may be a surrogate marker for another underlying surrogate marker for genetic susceptibility leading to more aggressive ALL.
We observed a toxicity rate regarding predefined specific reported toxicities of approximately 70% in the non-HR group, which is in line with toxicity rates observed in older children (69%), but higher compared to young children treated according to the same protocol (49%) [Citation20,Citation33]. Studies on the association between toxicity and BMI in children with ALL have shown a higher risk of toxicity in those classified as obese, especially liver toxicity, pancreatitis, and hyperglycemia [Citation7,Citation16,Citation18,Citation20,Citation34,Citation35]. The association between high BMI and treatment-related toxicity in young adults is less well explored, but a study on asparaginase-related toxicity in young adults treated with pediatric protocols demonstrated an increased risk of hepatotoxicity [Citation36]. We observed a tendency for liver dysfunction within the obese or severely obese BMI categories, and a correlation of BMI and increasing IRR for liver dysfunction. Non-alcoholic fatty liver disease is prevalent in obese individuals and may have contributed to more pronounced hepatotoxicity. As only severe liver toxicity was registered, we may have missed patients with less serious liver dysfunction. The asparaginase dose for young adults treated with the NOPHO ALL2008 protocol was lower (1000 IE/m2) compared to other protocols, which might also have affected the incidence of severe liver dysfunction. We observed an association with high BMI and a higher risk of hyperglycemia during induction and hyperlipidemia. The underweight patients had a higher incidence of osteonecrosis, which is often diagnosed later in the treatment and thus more seldomly leads to treatment modifications or delays [Citation37].
In children, truncation of asparaginase has been discussed as a possible factor for increased relapse rate [Citation13,Citation14] and indeed in our previous study, older obese children had more asparaginase-related toxicities than healthy-weight children [Citation20]. However, obese or severely obese adults with ALL treated according to the same protocol did not have a higher risk of truncation or more asparaginase-related toxicities (thrombosis, pancreatitis, anaphylactic reaction, osteonecrosis) than the healthy-weight group. There is a known association between thrombosis and increasing age in patients treated with ALL, as well as an increased risk of thrombosis in obese patients in general [Citation38]. We expected to find higher IRR for thrombosis with increasing BMI. Yet, there was no association between higher BMI and an increasing risk of thrombosis. As several other factors contribute to thrombosis risk under ALL treatment, and thromboprophylaxis is commonly used during ALL treatment, particularly in adults, our study may not be powered to detect the impact of obesity on thrombosis.
A major limitation of this study is the small cohort of patients with severe obesity and the lack of data on dose adjustments of the chemotherapeutic agents except for asparaginase. The results should therefore be interpreted with caution. Other limitations include that only predefined SAEs were registered and that data on other expected toxicities such as infection and less severe liver- or kidney dysfunction, are missing. As we only had data on BMI at diagnosis and not during treatment, we could not study whether a change in BMI during treatment contributed to the outcome.
The association between obesity and increased risk for cancer and inferior outcome is known, but the reasons behind this are not understood. We explored the role of BMI as a prognostic factor in young adults with ALL. We show that severely obese patients had increased relapse rates but not more predefined SAEs, treatment delays, or higher risk of treatment-associated death than the healthy-weight group in young adults treated with the NOPHO ALL2008 protocol. One may speculate that obesity can lead to undertreatment due to the fear of toxicity. To study the effect of treatment deviations, modifications of chemotherapeutic doses should be registered in future studies. Furthermore, a better understanding of the negative impact of obesity on ALL outcome requires more research on the biology of adipose tissue, hormonal and genetic interactions, as well as better understanding of pharmacokinetics in patients with extreme BMI.
Supplemental Material
Download MS Word (27.1 KB)Supplemental Material
Download MS Word (11.3 KB)Supplemental Material
Download PDF (17.5 KB)Supplemental Material
Download MS Word (34.6 KB)Disclosure statement
The authors report no conflicts of interest.
Data availability statement
The data that support the findings of this study are available from registry authorities, researchers are not allowed to forward confidential non-anonymous health data to any third parties. Group level data is available from the corresponding author, [CE], upon reasonable request.
Additional information
Funding
References
- Castillo JJ, Reagan JL, Ingham RR, et al. Obesity but not overweight increases the incidence and mortality of leukemia in adults: a meta-analysis of prospective cohort studies. Leuk Res. 2012;36(7):868–875. doi: 10.1016/j.leukres.2011.12.020.
- Larsson SC, Wolk A. Overweight and obesity and incidence of leukemia: a meta-analysis of cohort studies. Int J Cancer. 2008;122(6):1418–1421. doi: 10.1002/ijc.23176.
- Calle EE, Kaaks R. Overweight, obesity and cancer: epidemiological evidence and proposed mechanisms. Nat Rev Cancer. 2004;4(8):579–591. doi: 10.1038/nrc1408.
- De Pergola G, Silvestris F. Obesity as a major risk factor for cancer. J Obes. 2013;2013:291546–291511. doi: 10.1155/2013/291546.
- Orgel E, Genkinger JM, Aggarwal D, et al. Association of body mass index and survival in pediatric leukemia: a meta-analysis. Am J Clin Nutr. 2016;103(3):808–817. doi: 10.3945/ajcn.115.124586.
- Ethier MC, Alexander S, Abla O, et al. Association between obesity at diagnosis and weight change during induction and survival in pediatric acute lymphoblastic leukemia. Leuk Lymphoma. 2012;53(9):1677–1681. doi: 10.3109/10428194.2012.664843.
- Butturini AM, Dorey FJ, Lange BJ, et al. Obesity and outcome in pediatric acute lymphoblastic leukemia. J Clin Oncol. 2007;25(15):2063–2069. doi: 10.1200/JCO.2006.07.7792.
- Gelelete CB, Pereira SH, Azevedo AM, et al. Overweight as a prognostic factor in children with acute lymphoblastic leukemia. Obesity. 2011; 19(9):1908–1911. doi: 10.1038/oby.2011.195.
- Amankwah EK, Saenz AM, Hale GA, et al. Association between body mass index at diagnosis and pediatric leukemia mortality and relapse: a systematic review and meta-analysis. Leuk Lymphoma. 2016;57(5):1140–1148. doi: 10.3109/10428194.2015.1076815.
- Egnell C, Ranta S, Banerjee J, et al. Impact of body mass index on relapse in children with acute lymphoblastic leukemia treated according to nordic treatment protocols. Eur J Haematol. 2020 Dec;105(6):797–807. doi: 10.1111/ejh.13517.
- Wieduwilt MJ, Stock W, Advani A, et al. Superior survival with pediatric-style chemotherapy compared to myeloablative allogeneic hematopoietic cell transplantation in older adolescents and young adults with Ph-negative acute lymphoblastic leukemia in first complete remission: analysis from CALGB 10403 and the CIBMTR. Leukemia. 2021;35(7):2076–2085. doi: 10.1038/s41375-021-01213-5.
- Stock W, Luger SM, Advani AS, et al. A pediatric regimen for older adolescents and young adults with acute lymphoblastic leukemia: results of CALGB 10403. Blood. 2019;133(14):1548–1559. doi: 10.1182/blood-2018-10-881961.
- Gupta S, Wang C, Raetz EA, et al. Impact of asparaginase discontinuation on outcome in childhood acute lymphoblastic leukemia: a report from the children’s oncology group. J Clin Oncol. 2020;38(17):1897–1905. doi: 10.1200/JCO.19.03024.
- Gottschalk Hojfeldt S, Grell K, Abrahamsson J, et al. Relapse risk following truncation of PEG-asparaginase in childhood acute lymphoblastic leukemia. Blood. 2020;137(17):2373–2382. doi: 10.1182/blood.2020006583.
- Eissa HM, Zhou Y, Panetta JC, et al. The effect of body mass index at diagnosis on clinical outcome in children with newly diagnosed acute lymphoblastic leukemia. Blood Cancer J. 2017;7(2):e531–e531. doi: 10.1038/bcj.2017.11.
- Denton CC, Rawlins YA, Oberley MJ, et al. Predictors of hepatotoxicity and pancreatitis in children and adolescents with acute lymphoblastic leukemia treated according to contemporary regimens. Pediatr Blood Cancer. 2018;65(3):e26891. doi: 10.1002/pbc.26891.
- Hijiya N, Panetta JC, Zhou Y, et al. Body mass index does not influence pharmacokinetics or outcome of treatment in children with acute lymphoblastic leukemia. Blood. 2006;108(13):3997–4002. doi: 10.1182/blood-2006-05-024414.
- Orgel E, Sposto R, Malvar J, et al. Impact on survival and toxicity by duration of weight extremes during treatment for pediatric acute lymphoblastic leukemia: a report from the children’s oncology group. J Clin Oncol. 2014;32(13):1331–1337. doi: 10.1200/JCO.2013.52.6962.
- Hashmi SK, Navai SA, Chambers TM, et al. Incidence and predictors of treatment-related conjugated hyperbilirubinemia during early treatment phases for children with acute lymphoblastic leukemia. Pediatr Blood Cancer. 2020;67(2):e28063.
- Egnell C, Heyman M, Jonsson OG, et al. Obesity as a predictor of treatment-related toxicity in children with acute lymphoblastic leukaemia. Br J Haematol. 2022;196(5):1239–1247. doi: 10.1111/bjh.17936.
- Duarte X, Esteves S, Neto AM, et al. Incidence and risk factors for central nervous system thrombosis in paediatric acute lymphoblastic leukaemia during intensive asparaginase treatment: a single-centre cohort study. Br J Haematol. 2016; 174(2):280–291. doi: 10.1111/bjh.14048.
- Prasca S, Carmona R, Ji L, et al. Obesity and risk for venous thromboembolism from contemporary therapy for pediatric acute lymphoblastic leukemia. Thromb Res. 2018;165:44–50. doi: 10.1016/j.thromres.2018.02.150.
- Niinimaki RA, Harila-Saari AH, Jartti AE, et al. High body mass index increases the risk for osteonecrosis in children with acute lymphoblastic leukemia. J Clin Oncol. 200725(12):1498–1504. doi: 10.1200/JCO.2006.06.2539.
- Doney K, McMillen K, Buono L, et al. Impact of body mass index on outcomes of hematopoietic stem cell transplantation in adults. Biol Blood Marrow Transplant. 2019;25(3):613–620. doi: 10.1016/j.bbmt.2018.10.006.
- Fuji S, Takano K, Mori T, et al. Impact of pretransplant body mass index on the clinical outcome after allogeneic hematopoietic SCT. Bone Marrow Transplant. 2014;49(12):1505–1512. doi: 10.1038/bmt.2014.178.
- World Health Organization. Obesity: preventing and managing the global epidemic: report of a WHO consultation. World Health Organ Tech Rep Ser. 2000;894:i-xii, 1–253. Available online: https://apps.who.int/iris/handle/10665/42330
- Toft N, Birgens H, Abrahamsson J, et al. Risk group assignment differs for children and adults 1-45 yr with acute lymphoblastic leukemia treated by the NOPHO ALL-2008 protocol. Eur J Haematol. 2013;90(5):404–412. doi: 10.1111/ejh.12097.
- Frandsen TL, Heyman M, Abrahamsson J, et al. Complying with the European clinical trials directive while surviving the administrative pressure - an alternative approach to toxicity registration in a cancer trial. Eur J Cancer. 2014;50(2):251–259. doi: 10.1016/j.ejca.2013.09.027.
- Raja RA, Schmiegelow K, Albertsen BK, et al. Asparaginase-associated pancreatitis in children with acute lymphoblastic leukaemia in the NOPHO ALL2008 protocol. Br J Haematol. 2014;165(1):126–133. doi: 10.1111/bjh.12733.
- Gray B. cmprsk: subdistribution Analysis of Competing Risks. R package version 2.2-7. 2014 [cited 2019 14 Dec]. Available from: https://CRAN.R-project.org/package=cmprsk.
- von Elm E, Altman DG, Egger M, et al. The strengthening the reporting of observational studies in epidemiology (STROBE) statement: guidelines for reporting observational studies. J Clin Epidemiol. 2008;61(4):344–349. doi: 10.1016/j.jclinepi.2007.11.008.
- Griggs JJ, Bohlke K, Balaban EP, et al. Appropriate systemic therapy dosing for obese adult patients with cancer: ASCO guideline update. J Clin Oncol. 2021; 39(18):2037–2048. doi: 10.1200/JCO.21.00471.
- Toft N, Birgens H, Abrahamsson J, et al. Toxicity profile and treatment delays in NOPHO ALL2008-comparing adults and children with Philadelphia chromosome-negative acute lymphoblastic leukemia. Eur J Haematol. 2016;96(2):160–169. doi: 10.1111/ejh.12562.
- Meenan CK, Kelly JA, Wang L, et al. Obesity in pediatric patients with acute lymphoblastic leukemia increases the risk of adverse events during pre-maintenance chemotherapy. Pediatr Blood Cancer. 2019;66(2):e27515. doi: 10.1002/pbc.27515.
- Lowas SR, Marks D, Malempati S. Prevalence of transient hyperglycemia during induction chemotherapy for pediatric acute lymphoblastic leukemia. Pediatr Blood Cancer. 2009;52(7):814–818. doi: 10.1002/pbc.21980.
- Christ TN, Stock W, Knoebel RW. Incidence of asparaginase-related hepatotoxicity, pancreatitis, and thrombotic events in adults with acute lymphoblastic leukemia treated with a pediatric-inspired regimen. J Oncol Pharm Pract. 2018;24(4):299–308. doi: 10.1177/1078155217701291.
- Biddeci G, Bosco G, Varotto E, et al. Osteonecrosis in children and adolescents with acute lymphoblastic leukemia: early diagnosis and new treatment strategies. Anticancer Res. 2019;39(3):1259–1266. doi: 10.21873/anticanres.13236.
- Stein PD, Goldman J. Obesity and thromboembolic disease. Clin Chest Med. 2009;30(3):489–493, viii. doi: 10.1016/j.ccm.2009.05.006.