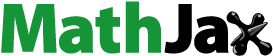
ABSTRACT
Global wheat yields must increase to meet current and rising global demand despite the increasing threats to production resulting from climate change. One climate change adaptation strategy in wheat production in the Western Cape of South Africa is conservation agriculture (CA). Using a data set of 1,043 plot-level wheat observations collected at Langgewens and Tygerhoek research farms from 2002 to 2020, this study conducts a stepwise Life Cycle Assessment (LCA) to estimate the environmental and economic impacts of switching from conventional wheat production to CA’s zero tillage (zero-till) and no-tillage (no-till) systems. The results indicate that CA is more profitable and has a higher environmental efficiency, than conventional tillage wheat production. In Langgewens, zero-till and no-till are respectively 113% and 55% more efficient than conventional tillage when comparing the environmental impact of producing one kg of wheat. Findings also suggest that, compared to 100% conventional tillage wheat production, the adoption of CA systems has led to reductions in environmental damage valued between R269.2 and R402.5 million in the Western Cape.
1. Introduction
Conservation agriculture (CA) is a climate-smart agricultural practice that aims to improve farmers’ livelihoods in the midst of climate change. As an alternative to conventional tillage practices, which in some cases leads to land degradation, CA is considered a more sustainable farming approach that internalises both environmental and economic metrics when evaluating success (Mitchell et al. Citation2019). Like most agricultural practices, the benefits of CA are heterogeneous across regions and even within countries (Giller et al. Citation2009), with some benefits (such as increased yield) not realised for several growing seasons. A better understanding of the holistic (environmental and economic) benefits of CA and their respective spatial and temporal components could help increase CA adoption in low- and lower-middle-income countries as producers often have a higher discount rate for money. While there is extensive literature on CA and its effect on crop yields (Rusinamhodzi et al. Citation2011; Lawrance, Prinsloo, and Berry Citation1999), little research has been conducted in monetising CA’s environmental impacts in Sub-Saharan Africa (SSA). Economists and producers often recognise the presence of environmental benefits from CA adoption but often fail to quantify these benefits with little to no attempt to monetise them. A more holistic (both economic and environmental) valuation of CA could help producers and policymakers adopt/incentivize the adoption of CA in SSA. Further, by monetising the environmental externalities of production using an LCA approach, economists extend possibilities of accounting for the social cost of environmental burdens resulting from technological shifts in agricultural production, leading to more informed decision making in SSA food systems.
Since the 1990s, global CA adoption has increased through producer education and non-governmental and governmental action to highlight the environmental and economic benefits of CA, specifically in the face of increased climate variability (Kassam, Friedrich, and Derpsch Citation2019). By estimating the holistic benefits CA, producers can potentially internalise the economic, agronomic, environmental, and social benefits of adoption. Ideally, these benefits are not mutually exclusive. Although CA has seen low adoption across Africa, South Africa has experienced a large increase in CA adoption, specifically in dryland production areas (Kassam, Friedrich, and Derpsch Citation2022), given its large-scale commercial agricultural setting and increased frequency and severity of droughts and heat events (Archer et al. Citation2019). One important question in the South African context is, has the adoption of CA benefited producers, the environment or possibly both? Or was adoption of CA simply a product of a changing environment which could possibly reduce producer profitability and increase the metric of “environmental damage per unit of output?”
The Western Cape province, which accounts for 60% of South Africa’s wheat production (USDA Citation2020), has experienced the largest relative and absolute adoption of CA. According to ARC (Citation2021), CA’s increased economic and ecological sustainability are the contributing factors pushing its expansion in Western Cape. Previous studies have shown that adoption of CA in wheat production in Western Cape has resulted in increased yields and profit, reduced soil erosion, and improved water quality and soil health (Knott, Hoffmann, and Strauss Citation2017). CA is increasing in importance for South Africa’s rainfed agriculture sector as it responds to the country’s low and increasingly erratic rainfall and poor-quality soils (van Antwerpen et al. Citation2021).
While CA adoption has seemingly been driven by an increase in both climatic variability and input costs, this study sets out to address if CA is more efficient from both an environmental and economic perspective. The adoption of agricultural practices that increase marginal profitability in the short run but could exacerbate environmental degradation and climate change in the long run is not sustainable. Importantly, the findings presented in this study have direct relevance to policymakers and stakeholders interested in quantifying environmental damage in the current era of adapting food systems to global climate change. Thus, a monetisation, in Rand, of the environmental damage per unit of output (kg of wheat) produced is a metric policymakers and producers can internalise.
Using primary data from long-term wheat trials in Western Cape, this study uses the LCA framework to examine two CA wheat production practices and their effects on changes in traditional profitably efficiency as well as changes in environmental efficiency. The study uses a Stepwise Life Cycle Impact Assessment (LCIA) to monetise conventional tillage and CA’s environmental impact in commercial wheat production in the Western Cape province of South Africa. The Stepwise LCIA method allows the holistic quantification of environmental damages of a process or product as a single score expressed in South African Rand (R) per unit of output (kg of wheat), Quality Adjusted Life Years (QALYs), or Biodiversity Adjusted Hectare Years (BAHYs) (Weidema Citation2009).
This paper is unique amongst the CA literature as it values and then compares the efficiency of switching from conventional tillage to CA (zero or no-till) wheat production from both a producer profitability and environmental standpoint. Using yearly data collected on 1,043 trial plots from 2002 to 2020 in Langgewens and Tygerhoek research farms, we compare Stepwise LCIA single scores for one hectare and one kilogram of wheat produced in Western Cape under zero-till, no-till, and conventional wheat production. Policymakers can use the results of this study to potentially create producer incentives in Western Cape and other wheat-growing areas of South Africa to adopt CA. A large contribution of this study is that when decision-makers evaluate input-reducing research, such as CA, they should look beyond cost savings to include increased input use efficiency and internalise the wider environmental implications when trying to maximise agricultural production efficiency.
2. Literature review
2.1 CA adoption in South Africa
In 2020, some form of CA was estimated to be adopted on 25% of South Africa’s cropland (Smith Citation2021; Strauss et al. Citation2021). South Africa accounts for 51% of CA farmland in Africa (Kassam, Friedrich, and Derpsch Citation2022). CA is typically implemented on large-scale commercial production with limited adoption amongst smallholder and subsistence farming operations across South Africa (Mudavanhu Citation2015). While there is spatial heterogeneity in adoption, there is also a distinction between commercial and subsistence farming adoption rates with CA. It was estimated that CA was adopted on just 0.84% of subsistence farms across South Africa in 2020 (Smith Citation2021). The area under CA production in South Africa has increased by 366% from 2016 to 2019 (Kassam, Friedrich, and Derpsch Citation2022). Unlike in Europe and the United States, where there are government programmes which incentivize CA adoption, in South Africa adoption has been producer driven, likely a result of increased input costs and precipitation variability.
The adoption of CA in commercial production in SSA has been traditionally associated with reduced costs and decreased soil degradation. Additional motives for CA adoption in South Africa include climate shock mitigation, a growing awareness amongst producers, and efforts to improve resource management (Blignaut et al. Citation2015). Previous research has indicated that the decision to adopt CA by commercial farmers in South Africa is individually-motivated, and these adopters were not incentivized by government programmes (Findlater, Kandlikar, and Satterfield Citation2019). In the Western Cape province, CA adoption was also facilitated by the availability of no-till machinery made possible in the late 1990s (Strauss et al. Citation2021). The higher cost for suitable planters and the lack technical expertise were both limiting factors in early adoption of CA in South African commercial farming (Modiselle, Verschoor, and Strauss Citation2015).
In 2021, Western Cape had a CA adoption rate estimated at 51% of its annual cropland, the highest area and percentage in South Africa (Smith Citation2021). In the rest of South Africa, CA’s major field crops range from maize, soybean, and sunflower to pastures, while in Western Cape, wheat in rotation with legumes is the primary winter cropping system found under CA (Smith et al. Citation2017). A survey conducted by Modiselle, Verschoor, and Strauss (Citation2015) suggests that 49% of commercial wheat farmers in Western Cape implemented all three principles of CA (no or low soil disturbance, mulching, and rotation), and another 49% implement at least one of the three CA principles.
2.2 CA and profitability in South Africa
The existing economic research in South Africa on CA has primarily focused on CA’s crop diversification principle, which contributed to the dissemination of CA in South Africa, assessing financial profitability in wheat production (Knott Citation2015; Visser Citation2014). In the Western Cape province, Knott (Citation2015) simulated a wheat farming budget over a 20 year period, demonstrating that investing in wheat monoculture and conventional tillage led to negative present value (NPV) and internal rates of return (IRR) below the real interest rate (2.73%). Knott (Citation2015) also found that CA led to varying positive IRRs and NPVs depending on the rotation option. Visser (Citation2014) and Knott (Citation2015) highlight the financial implication of implementing CA principles and show that crop rotations under CA improve wheat production profitability.
2.3 Environmental externalities of CA
Previous literature has shown that CA has the potential to provide environmental benefits in Southern Africa when compared to conventional tillage (Thierfelder et al. Citation2015). Some of the main environmental benefits can include increased water infiltration, reduced soil erosion and run-off, improved soil structure, increased biodiversity, improved soil, air, and water quality, and carbon sequestration (Thierfelder et al. Citation2015).
Previous research by Knot (Citation2014) quantified the environmental impact in the form of GHG emissions between CA and conventional wheat production using inputs and an assumed an arbitrary carbon tax of R120 per ton of CO2-eq. Knot (Citation2014) found that over seven years, CA implemented with no agrochemical inputs had a lower environmental cost compared to conventional tillage production. The diesel requirements were the main driver of environmental damage from conventional tillage, requiring 79% more diesel per hectare than CA (Knot Citation2014). While Knot (Citation2014) provided a valuable first insight into the effects of CA adoption on GHG emissions, environmental degradation manifests itself in many alternative forms besides GHG emissions.
Although CA can reduce the amount of diesel requirements in production, empirical evidence has shown that CA can increase weed infestation and CA crops can benefit from an increase in herbicides use (Corbeels et al. Citation2020; Singh et al. Citation2015). Under CA, the efforts to control weeds in eastern Free State, were significantly higher per hectare more in herbicides (Knot Citation2014). A 2015 survey of commercial wheat farmers in Western Cape, indicated 60% reported increased weed control costs while 40% spent more on pest and insects control as a result of implementing CA (Modiselle, Verschoor, and Strauss Citation2015). Unlike Knot (Citation2014), whose assessment of the environmental impact of CA in South Africa was limited to analysing GHG emissions, LCA approaches CA’s environmental impact more holistically providing various quantifiable impact categories and accounting for inputs and outputs of processes and products involved in commercial wheat production.
2.4 Estimating the environmental impact of wheat production
LCA is a compilation and evaluation of a product system’s inputs, outputs, and potential environmental impacts throughout its life cycle (International Standard Organization [ISO] Citation2006). Unlike previous studies that assumed an arbitrary value of carbon (Knot Citation2014) to value environmental damage and those who only focused on carbon dioxide emissions (de Kock et al. Citation2018), this study uses the Stepwise LCIA method to monetise multiple aspects of environmental damages from the production of a specific metric (per kg or per ha) of wheat produced in the Western Cape province. Monetising environmental damage allows policymakers, researchers, and other stakeholders to either try to reduce environmental damage holding yield constant, increase yield while holding environmental damage constant, or, ideally, increase yield while reducing environmental damage by increasing the “efficiency” at turning environmental damage into a kilogram of wheat. As the Stepwise LCIA method monetises the value of environmental damage, it is possible to capture and figure out ways to minimise the negative environmental impacts of agricultural production. Commercial wheat farming in South Africa provides an interesting medium for studying this environmental “efficiency” measurement as CA has been identified as both an input reducing practice but one which has mostly been adopted out of necessity due to climate change (Archer et al. Citation2019).
3. Materials and methodology
3.1 Research data and location
The Western Cape wheat data used for this study was extracted from the long-term trials database led by the Directorate Plant Sciences of the Western Cape Department of Agriculture (DPS). The data set is comprised of 1,043 plot-level rainfed winter wheat production observations collected at the Tygerhoek and Langgewens research farms from 2002 to 2020 (Western Cape Department of Agriculture [WCDA] Citation2021). The individual plot size ranged from 0.5 to 2.0 ha. Each year contained data related to each plot used in that particular year. Each plot contained information on the cropping system, gross income and margin, prices of wheat produced during the winter season, types and costs of wheat seed, fertilisers, amendments, fungicides, herbicides, insecticides, machinery, and operational field activities. Two CA systems were implemented: no tillage (no-till) and zero tillage (zero-till). According to the ARC (2021), both no-till and zero-till in the South African context refer to the seeder used to implement the CA system. In the case of no-till, a knifepoint opener is used to place seed and fertiliser, while for zero-till the placement is executed with discs. One could also describe the use of the knifepoint opener as “high disturbance” no-till and the use of discs as “low disturbance” no-till. The production information related to conventional tillage used in this study was sourced from Knott (Citation2015) based on data collected at Langgewens experimental farm between 2007 and 2013. Ideally, there would be head-to-head comparisons of CA and conventional tillage, but this was not available for the WCDA dataset. Knott (Citation2015) described and used three yield scenarios for conventional wheat in Western Cape based on seasonal variations, a poor, an average, and a good yielding year.
3.2 Environmental impact estimation
LCA was used to quantify the cradle-to-farm gate environmental impacts of producing one kilogram (kg) and one hectare (ha) of wheat under CA’s no-till and zero-till on two experimental farms in the Western Cape. Following similar environmental impact studies (Durand-Morat, Nalley, and Thoma Citation2018; Nalley et al. Citation2016; Shew et al. Citation2020), the LCA aimed to elicit the environmental impacts of applying the two CA systems and conventional commercial wheat production. Comparisons were made between one kg and one ha of wheat produced under no-till and zero-till using the LCA software SimaPro 9.1.0.8.Footnote1 (PRé Sustainability B.V.) and the Ecoinvent and Agri-footprint databases (Durlinger et al. Citation2017; Wernet et al. Citation2016). Estimating two functional units in LCA research (e.g., one kg and one ha of wheat) is not uncommon and depends on study’s objective (Hayashi Citation2013; Cerutti et al. Citation2013). The LCA provides an environmental cost in Rand from the environmental externalities of producing one kg and one ha of wheat using the preceding farming practices in the Western Cape province. presents the impact categories included in the current LCA.
Table 1. Impact categories used in the LCA for CA and conventional wheat production in South Africa (Stepwise method).
The average yields for each farming practice at Tygerhoek and Langgewens () were entered into SimaPro along with their respective inputs (e.g., diesel fuel, fertilisers, amendments, and pesticides) and amounts (per hectare) to serve in the computation of environmental damage per kg and hectare in the LCA Stepwise analysis. The amounts of these inputs were all averaged across year and plot by production practice. The production cost and subsequent variance in Langgewens is larger () which is explained by the larger amounts of fertilisers and soil amendments required. Detailed inputs for Tygerhoek and Langgewens used in this analysis are given in .
Table 2. Average yield and production costs (R2020) per hectare for no-till and zero-till in Tygerhoek and Langgewens: 2002–2020.
The Stepwise LCIA method applied in SimaPro provided a combined score for both human and environmental effects in monetary terms (Weidema Citation2009). Ala-Kokko et al. (Citation2021) provides a consistent framework that applied the Stepwise LCIA method to estimate the cost of environmental externalities attributed to genetically modified (GM) adoption compared to conventional maize adoption in South Africa. The Stepwise method has midpoint characterisation factors and endpoint characterisation factors (Weidema et al. Citation2008; Weidema Citation2015). In addition, normalisation and weighting factors based on the European Union cumulative per-capita emissions in 1995 are included. The method extends other impact assessment approaches based on damage characterisation to human health and the ecosystem as defined by QALY and BAHY. The two measures are related to estimated costs associated with various contributing factors to different midpoint impact categories, as presented in . Further, the method assigns a cost of 1/54 QALY per BAHY (Weidema et al. Citation2008; Weidema Citation2015). The costs presented, as a function of the outputs on , are the estimated expenses to offset environmental and human health externalities when choosing to implement CA’s no-till or zero-till or conventional tillage in wheat production. That is, to restore full QALYs and BAHYs based on the “ability to pay,” derived from resource constraints and the equivalence factor between QALY and BAHY adopted by the method. The Stepwise cost outputs were generated in Euro (EUR) 2003, adjusted to EUR 2020, accounting for inflation, then converted to R2020.
3.3 Environmental efficiency
Environmental efficiency has received several names in agricultural LCA research, but its interpretation is based on the environmental impact found on ecosystems. Common names seen in LCA literature include agricultural eco-efficiency (Wang et al. Citation2022), productive efficiency (Tricase et al. Citation2018), EcoX indicator (Brentrup et al. Citation2004), eco-efficiency (Masuda Citation2016), and environmental efficiency (Cerutti et al. Citation2013). In this study, the term “environmental efficiency” used by Cerutti et al. (Citation2013) is preferred as it considers mass-based interpretation to better define the environmental performance of a production system.
The comparison of the LCA single score (the combined impact of all LCA categories from into one monetised score) in R2020Footnote2 per kg of wheat allows for a comparison in efficiency between the two CA production methods and conventional tillage. The increase in environmental efficiency is described as the change in the ratio of monetised environmental damage, was calculated as
(1)
(1) where Env.SSconv and Env.SSCA are respectively LCA single score costs for conventional and CA wheat, respectively.
3.4 Profitability of commercial wheat production
This study also implements a profitability analysis comparing no-till versus zero-till in Langgewens and Tygerhoek and a comparison of all three production methods in Langgewens. We use plot-level wheat production reports from 2002 to 2020 (WCDA 2021) to estimate average wheat yields, prices, and production costs across years. All price and cost values were given in Rands and inflation-adjusted to January 1, 2020. summarises the information used for profit simulation.
Profitability for wheat production under CA’s no-till and zero-till in Langgewens and Tygerhoek, respectively, was simulated using @Risk© (Palisade, Ithaca, NY). Following similar studies (Nalley et al. Citation2016; Ala-Kokko et al. Citation2021), 1,000 iterations were run for each of the two sites, and a two-tailed t-test was used to test for statistical differences between the profitability of producing wheat under no-till and zero-till. The simulated profit and environmental impact (i.e., the environmental cost obtained from Stepwise LCA) are used to derive the net impact of switching from conventional to no-till and zero-till. The economic impact of switching is the profit difference between no-till and zero-till, respectively, and conventional tillage.
The simulated net profit (NP) without environmental benefits for a hectare of wheat production was calculated as
(2)
(2) where NPij is the simulated net profit as a function of total revenue (TRij) and total cost (TCij) per hectare of producing wheat under ith CA system (zero-till or no-till) in jth site (Langgewens or Tygerhoek) all of which were simulated using a normal distribution from the statistics given on . The TRij was obtained from
(3)
(3) where Yij the simulated wheat yield in ton per hectare under ith practice (zero-till or not-till) from at jth site (Langgewens or Tygerhoek) was multiplied by the simulated price of wheat per ton (P), from 2002–2020 in R2020. The simulated price mean (in ZAR inflation-adjusted to January 1, 2020) was R3,804.06 with a standard deviation of R741.64 per ton (WCDA 2021). Wheat prices for each production regime have been adjusted for quality (grades) by year ().
The environmental benefits (EBi), in R2020 per hectare, from switching from conventional to CA production system i per hectare can be calculated as:
(4)
(4) where ECcon is the LCA single score cost of producing one hectare of conventionally tilled wheat and ECCA is the LCA single score cost of producing one hectare of wheat using CA system i.
The total benefits (TB) in R2020 per hectare of switching from conventional wheat production to either zero-till or no-till production in Langgewens was calculated as
(5)
(5) where the difference in net profit from Equation (2) (ΔNP) between conventional and CA system i (zero-till or no-till) is summed with the change environmental benefits (ΔEB) from Equation (4) of switching from conventional to CA system i (zero-till or no-till) wheat production.
4. Results and discussion
4.1 Wheat production environmental efficiency
The single score results from the LCA are presented in . The single score is given in R2020 and is representative of the monetised environmental cost for producing one kilogram of wheat under zero-till, no-till, and conventional farming at Langgewens and Tygerhoek research sites in Western Cape. also provides the LCA scores for each impact category.
Table 3. Environmental impact costs (in R2020) for various wheat production methods using the Stepwise LCIA method for one kg of wheat production in Western Cape, South Africa.
The LCA single score results in indicate that for every kg of wheat produced in Langgewens there was R0.89 and R0.65 in environmental damage under no-till and zero-till wheat production, respectively. In Tygerhoek, there was R0.71 and R0.60 in environmental damage under no-till and zero-till wheat production, respectively. Given the additional nitrogen fertiliser usage requirements in Langgewens and lower yields, it is not surprising that its single scores were higher than Tygerhoek. The single scores for conventional tillage wheat production were R2.92, R1.80, and R1.37 per kg in environmental damage in a poor, an average, and a good yield scenario, respectively in Langgewens. These findings suggest that CA wheat production has a lower environmental impact than conventional wheat production and, among CA systems, zero-till has a lower environmental impact than no-till.
These results provide important metrics about the efficiency at turning environmental damage (input) into a kg of wheat (output). suggests that zero-till is 352%, 178%, and 113% more efficient at converting environmental damage into a kg of wheat compared to conventional tillage under poor, average and good yielding scenarios, respectively. Another way of viewing this efficiency gain is that for the same environmental damage as one kg of conventional tillage under poor, average, and good yielding scenarios you could yield 3.52, 1.78, and 1.13 more kgs of wheat, respectively, with zero till wheat production in Langgewens. Using the same methodology, no-till is 229%, 102%, and 55% more efficient at converting environmental damage into a kg of wheat than conventional tillage under poor, average, and good yielding scenarios, respectively.
Estimating per hectare environmental damage is a function of the single scores reported on multiplied by the average yields on . It was estimated in Langgewens to be R3,039, R2,134, and R4,671 worth of environmental damage per hectare for no-till, zero-till, and conventional wheat production, respectively. In Tygerhoek, this environmental cost per hectare was estimated to be R2,567 and R2,018 for no-till and zero-till, respectively.
The total environmental cost expresses the cost of the overall damage wheat production inflict on ecosystems, estimated per kg of wheat produced in Langgewens and Tygerhoek and disaggregated per impact category (). Respiratory inorganics and effects associated with global warming from fossil fuels accounted for 91.7% of the environmental costs associated with conventional, no-till, and zero-till wheat production at Langgewens and Tygerhoek. The other environmental burdens were clustered around photochemical ozone, terrestrial eutrophication, terrestrial ecotoxicity, human toxicities, and land occupation ().
While increasing environmental efficiency is becoming more important for policymakers and producers, the driving factor of agricultural technology is producer profitability. If found to be less profitable than conventional tillage, and without government incentives, commercial wheat producers will likely continue to practice conventional tillage.
4.2 Profitability differences between CA and conventional wheat production
4.2.1 CA systems economic profitability
The results of the profit simulations (using Equation (2)) indicate () that the average gain in ZAR per ha obtained from CA wheat production varies by location and CA system. In Tygerhoek and Langgewens, the no-till profit is 6% and 4% higher than zero-till, respectively (). CA economic profit is a function of several factors, including crop yields which are heterogenous across the Western Cape (Swanepoel, Swanepoel, and Smith Citation2018).
Table 4. Estimated net profit per hectare (R2020) for CA wheat production in Western Cape, South Africa.
Table 5. Average total benefits (R2020 /ha) associated with switching to CA from conventional tillage systems with varying yields per hectare in Langgewens.
4.2.2 Holistic benefit of switching to CA systems in Western Cape
The results of the LCA single scores suggest that there are more benefits beyond the economic profit from CA adoption. provides the traditional metric of profit (not accounting for environmental damage), the environmental metric (LCA single score per hectare) and a combined metric (traditional plus environmental) for profitability between CA systems and conventional tillage per hectare in Langgewens. Importantly, may shed some light on why CA has not reached full adoption potential as the difference between conventional profitability under a good year of conventional tillage is only marginally different than CA systems. When accounting for the environmental benefits (which most producers do not internalise) the benefit from switching from conventional to zero-till goes from R55 per hectare under the traditional method of measuring profitability to R2,593 per hectare. Under poor and average yields for conventional tillage, CA adoption seems to make economic sense, even in the traditional profitability accounting system. However, there seems to be some hesitation to CA adoption when looking at conventional tillage with good yields, specifically given the fact that a 2015 survey of commercial wheat farmers in the Western Cape, indicated 60% reported increased weed control costs and more health risks, while 40% spent more on pest and insects control as a result of implementing CA (Modiselle, Verschoor, and Strauss Citation2015).
While is important because it shows averages, estimates the percentage chance of being more profitable by adopting CA using the traditional (straight profit), environmental (per hectare LCA single score), and combined (traditional plus LCA single score) metrics. shows that on average (with average conventional yields) zero and no-till have a 75 and 74% chance of being more profitable than conventional tillage, respectively. When accounting for the environmental services from switching from conventional to CA, shows that zero and no-till have a 90 and 86% chance of being more profitable than conventional tillage, respectively. Interestingly, assuming a “good yield” for conventional tillage zero and no-till only have a 48 and 46% chance of being more profitable than conventional tillage, respectively indicating they are less profitable. These numbers increase to 70 and 62% for zero and no-till, respectively, indicating that without accounting for the environmental services that CA can provide, what on the surface looks like a lucrative production practice, “conventional tillage,” can provide misleading results from a holistic standpoint. That is, without accounting for the environmental services provided by CA adoption, producers and policymakers may think that conventional tillage is the correct practice to adopt and endorse for long run sustainability.
Table 6. Estimated probability of being more profitable per hectare by switching from conventional tillage with varying yields to CA systems in Langgewens.
Table 7. Environmental benefits (R2020) of complete adoption of zero and no-till wheat production in the Western Cape from conventional tillage practice.
4.2.3 Province level implications of CA adoption
While a per hectare analysis provides a small snapshot of the benefits of any technology, it is important to extrapolate the benefits of a technology to actual adopted area. As such, we ask the counterfactual question based on the findings from the LCA single scores, “how much additional environmental damage would have occurred if wheat producers in the Western Cape did not adopt CA?” We take the LCA single scores by production type () and their respective yields () to calculate the environmental damage that would be incurred to produce the entire 2020 wheat crop in the Western Cape. The difference between any two production practices provides the changes in environmental damage by switching production methods. Not surprisingly from the LCA single scores, 100% conventional tillage had the highest damage. However, given that an estimated 51% of the Western Cape is under CA (Smith Citation2021), the actual difference in environmental damage via CA adoption is 51% of the total difference between full adoption of conventional and full adoption of CA. In other words, without 51% of the wheat area under CA the estimated environmental damage would have been R402.5 and R269.2 million more than if 100% of land was under conventional tillage annually for zero and no-till, respectively (). Worth noting, these differences assume a “good yield” for conventional tillage and the benefits of CA would increase under an “average” or “poor” yielding conventional year.
Another way of looking at is what are the additional environmental benefits still left to obtain if the remaining 49% of the wheat area in the Western Cape adopted CA. indicates that if the remaining 49% of conventional tillage wheat producers switched to CA that there would be an additional R386.7 and R258.6 million annually in environmental gains to be captured if the remaining conventional wheat area adopted zero and no-till, respectively. The environmental benefits of CA adoption highlight two important concepts. First, by CA adoption in wheat production in the Western Cape, TFP is increased both from an input/output (increased profitability with less inputs) standpoint as well as environmental damage/output (reduced environmental damage per kg of wheat produced) standpoint. Importantly, in using the well-established categories defined by the UNEP/SETAC framework for LCIA (Jolliet et al. Citation2004), our results show that CA wheat leads to multiple environmental improvements over conventional production, a large addition to the literature which previously only looked at GHG emissions. Overall, the results from the producer, consumer, and environmental portions of this study are significant as agricultural scientists attempt to sustainably increase caloric production to feed a growing population.
While producers will likely not “capture” the environmental gains or increased environmental efficiency gains estimated in this study, as environmental concerns increase for consumers and policymakers alike the comparative statics from these estimates could be used for purchasing decisions. For instance, the global wheat industry may begin to source wheat from “more sustainable” production practices and policy makers may provide incentives/disincentives for more/less sustainable production practices. While CA adoption in South Africa has been more of a means of coping with climate change and mitigating increases in input costs and to date has not been marketed as holistically “sustainable” production, understanding the broader environmental implications of its adoption is important.
An important caveat with the scaling up of results to the province level is that we assume that all wheat area in the Western Cape can implement CA simultaneously, which is not a viable option. Because CA requires rotation out of wheat only a portion of the current land used for wheat production in the Western Cape could be available to produce wheat at any given time. Other crops integrated with wheat includes crops like barley and pasture which would require less inputs compared to wheat. That being said, 100% CA could dampen the supply of wheat annually given the requirements of such a rotation. Further, we assume that there is seamless transition from conventional tillage to no/zero-tillage when in reality new equipment maybe required and subsequent depreciation rates would need to be taken into account.
5. Conclusions
Given increased consumer and political awareness of environmental sustainability globally, it is time for economists to rethink the how we value the environmental impact of agricultural production. There is not a perfect correlation with simply reducing input amounts and a reduction in environmental impact, given differences in active ingredients and their environmental impacts across substitutable inputs (i.e., different types of herbicides). This study used a Stepwise LCA to quantify and compare CA to conventional farming in wheat production using the single score for environmental damage per kg of wheat as a measure efficiency across production methods. Our findings suggest that CA is more profitable and has a higher environmental efficiency, than conventional tillage wheat production in the Western Cape. Importantly, this study does not capture the temporal benefits of CA such as increased soil health and yields, which are likely enhanced, to some point, over time.
In the current era of trying to promote sustainable agricultural systems to feed a growing global population, novel agricultural practices like CA require further research contributions, in our case efficiency metrics, to guide policy design and agricultural development in order to make climate-smart decisions which will both enhance food security and reduce environmental degradation. In promoting sustainable development, the South African authorities could use LCA to quantify the environmental impact associated with different practices and encourage producers to use cleaner production methods. Based on the identified environmental cost of switching to CA, the government of Western Cape could invest in promoting CA and supporting commercial farmers to disseminate CA as it is more sustainable than conventional tillage. While producers will likely not receive payments for any of the environmental benefits they provide by switching from conventional to CA, the South African government could attempt to provide incentives for CA adoption in an effort to promote a sustainable wheat industry moving forward. While the estimated ecosystem benefits provided by CA in this study are relatively large (compared to the estimated increased in profitability through adoption), the benefits are not simply accrued by CA wheat producers, but rather by all of society. While producers do benefit from a variety of ecosystem benefits, their activities may strongly influence the delivery of services to other individuals who do not control the production of these services. The challenge is to use estimates from studies like this to develop policies and incentives that are easily implemented and adaptable to changing ecological and market conditions.
Data availability statement
Data is available from the authors upon request.
Notes
1 There were no data related to conventional tillage at Tygerhoek and as such only the two CA production methods could be compared. In Langgewens, data existed for all three production methods for a more complete comparison.
2 The conversion rate of US Dollars to South African Rand is USD1 = R16.17 as of May 10, 2022 (https://www.oanda.com/currency-converter).
References
- Agricultural Research Council [ARC] - Small Grain. 2021. Guideline 2021 - Production of Small Grains in the Winter Rainfall Area. Bethlehem, South Africa: ARC.
- Ala-Kokko, K., L. Nalley, A. Shew, J. Tack, P. Chaminuka, M. Matlock, and M. D’Haese. 2021. Economic and ecosystem impacts of GM maize in South Africa. Global Food Security 29: 100544.
- Archer, E., W. Landman, J. Malherbe, M. Tadross, and S. Pretorius. 2019. South Africa’s winter rainfall region drought: A region in transition? Climate Risk Management 25: 100188.
- Blignaut, J., J. Knot, H. Smith, N. Nkambule, D. Crookes, A. Saki, S. Drimie, S. Midgley, M. de Wit, W. von Loeper, et al. 2015. Promoting and advancing the uptake of sustainable, regenerative, conservation agricultural practices in South Africa with a specific focus on dryland maize and extensive beef production. Asset Research 2: 19.
- Brentrup, F., J. Küsters, J. Lammel, P. Barraclough, and H. Kuhlmann. 2004. Environmental impact assessment of agricultural production systems using the life cycle assessment (LCA) methodology II. The application to N fertilizer use in winter wheat production systems. European Journal of Agronomy 20, no. 3: 265–279.
- Cerutti, A.K., S. Bruun, D. Donno, G.L. Beccaro, and G. Bounous. 2013. Environmental sustainability of traditional foods: The case of ancient apple cultivars in northern Italy assessed by multifunctional LCA. Journal of Cleaner Production 52: 245–252.
- Corbeels, M., K. Naudin, A.M. Whitbread, R. Kühne, and P. Letourmy. 2020. Limits of conservation agriculture to overcome low crop yields in Sub-Saharan Africa. Nature Food 1, no. 7: 447–454.
- de Kock, L., E. Vienings, A. Blignaut, W. Pretorius, P. Fourie, and H. Smith. 2018. Determining the carbon footprint intensity of different winter grain farming regimes in the western cape. Pretoria: Grain SA.
- Durand-Morat, A., L.L. Nalley, and G. Thoma. 2018. The implications of red rice on food security. Global Food Security 18: 62–75.
- Durlinger, B., E. Koukouna, R. Broekema, M. van Paassen, and J. Scholten. 2017. Agri-footprint 4.0-Part 1: Methodology and basic principles. Agri-Footprint: Gouda, The Netherlands.
- Findlater, K.M., M. Kandlikar, and T. Satterfield. 2019. Misunderstanding conservation agriculture: Challenges in promoting, monitoring and evaluating sustainable farming. Environmental Science & Policy 100: 47–54.
- Giller, K.E., E. Witter, M. Corbeels, and P. Tittonell. 2009. Conservation agriculture and smallholder farming in Africa: The heretics’ view. Field Crops Research 114, no. 1: 23–34.
- Hayashi, K. 2013. Practical recommendations for supporting agricultural decisions through life cycle assessment based on two alternative views of crop production: The example of organic conversion. The International Journal of Life Cycle Assessment 18, no. 2: 331–339.
- International Standard Organization [ISO]. 2006. ISO 14040: environmental management-life cycle assessment-principles and framework. Geneva: ISO.
- Jolliet, O., R. Müller-Wenk, J. Bare, A. Brent, M. Goedkoop, R. Heijungs, N. Itsubo, et al. 2004. The LCIA midpoint-damage framework of the UNEP/SETAC life cycle initiative. The International Journal of Life Cycle Assessment 9, no. 6: 394.
- Kassam, A.H., T. Friedrich, and R. Derpsch. 2019. Global spread of conservation agriculture. International Journal of Environmental Studies 76, no. 1: 29–51.
- Kassam, A.H., T. Friedrich, and R. Derpsch. 2022. State of the global adoption and spread of conservation agriculture. In Advances in conservation agriculture volume 3: Adoption and spread, ed. Amir Kassam, 1–13. Cambridge, UK: Burleigh Dodds Science Publishing Limited.
- Knot, J. 2014. Promoting conservation agriculture and commercial farmers in the eastern free state. PhD diss., University of the Free State. https://scholar.ufs.ac.za/handle/11660/1490.
- Knott, S.C. 2015. An analysis of the financial implications of different tillage systems within different crop rotations in the Swartland area of the Western Cape, South Africa. MS thesis. Stellenbosch University.
- Knott, S., W. Hoffmann, and J. Strauss. 2017. The whole farm financial implications of different tillage systems on different crop rotations in the Swartland area of the western cape, South Africa. International Farm Management Association and Institute of Agricultural Management 6, no. 1: 20–31.
- Lawrance, K.F., M.A. Prinsloo, and W.A.J. Berry. 1999. Long and short term effects of tillage systems on grain yield, the incidence of diseases and production costs for maize. South African Journal of Plant and Soil 16, no. 2: 85–91.
- Masuda, K. 2016. Measuring eco-efficiency of wheat production in Japan: A combined application of life cycle assessment and data envelopment analysis. Journal of Cleaner Production 126: 373–381.
- Mitchell, J.P., D.C. Reicosky, E.A. Kueneman, J. Fisher, and D. Beck. 2019. Conservation agriculture systems. CABI Reviews 14, no. 001: 1–25.
- Modiselle, S., A.J. Verschoor, and Johann Strauss. 2015. Western cape wheat producers and conservation agriculture technology uptake. SA Grain 17, no. 5: 50–51.
- Mudavanhu, S. 2015. The impact of economic policies and instruments on conservation agriculture in South Africa. MS thesis. Stellenbosch University. https://www.researchgate.net/publication/289532130_The_impact_of_economic_policies_and_instruments_on_conservation_agriculture_in_South_Africa.
- Nalley, L., F. Tsiboe, A. Durand-Morat, A. Shew, and G. Thoma. 2016. Economic and environmental impact of rice blast pathogen (Magnaporthe Oryzae) alleviation in the United States. PloS One 11, no. 12: e0167295.
- Rusinamhodzi, L., M. Corbeels, M.T. Van Wijk, M.C. Rufino, J. Nyamangara, and K.E. Giller. 2011. A meta-analysis of long-term effects of conservation agriculture on maize grain yield under rain-fed conditions. Agronomy for Sustainable Development 31, no. 4: 657–673.
- Shew, A.M., J.B. Tack, L.L. Nalley, and P. Chaminuka. 2020. Yield reduction under climate warming varies among wheat cultivars in South Africa. Nature Communications 11, no. 1: 4408.
- Singh, V.P., K.K. Barman, R. Singh, and A.R. Sharma. 2015. Weed management in conservation agriculture systems. In Conservation agriculture, eds. Muhammad Farooq, and Kadambot H. M. Siddique, 39–77. New York: Springer.
- Smith, H.J. 2021. An assessment of the adoption of conservation agriculture in annual crop-livestock systems in South Africa.
- Smith, H.J., E. Kruger, J. Knot, and J.N. Blignaut. 2017. Conservation agriculture in South Africa: Lessons from case studies. In Conservation agriculture for Africa: Building resilient farming systems in a changing climate, eds. Amir H. Kassam, Saidi Mkomwa, and Theodor Friedrich, 214–245. Wallingford: CABI Publishing.
- Southern African Grain Laboratory [SAGL]. 2021. South African wheat crop – quality report 2020/2021 season. Pretoria.
- Strauss, J.A., P.A. Swanepoel, H. Smith, and E.H. Smit. 2021. A history of conservation agriculture in South Africa. South African Journal of Plant and Soil 38, no. 3: 196–201.
- Swanepoel, C.M., L.H. Swanepoel, and H.J. Smith. 2018. A review of conservation agriculture research in South Africa. South African Journal of Plant and Soil 35, no. 4: 297–306.
- Thierfelder, C., L. Rusinamhodzi, A.R. Ngwira, W. Mupangwa, I. Nyagumbo, G.T. Kassie, and J.E. Cairns. 2015. Conservation agriculture in Southern Africa: Advances in knowledge. Renewable Agriculture and Food Systems 30, no. 4: 328–348.
- Tricase, C., E. Lamonaca, C. Ingrao, J. Bacenetti, and A.L. Giudice. 2018. A comparative life cycle assessment between organic and conventional barley cultivation for sustainable agriculture pathways. Journal of Cleaner Production 172: 3747–3759.
- United States Department of Agriculture [USDA]. 2020. Grain and feed annual: Republic of South Africa. Washington, DC: USDA – Global Agricultural Information Network [GAIN]. https://apps.fas.usda.gov/newgainapi/api/Report/DownloadReportByFileName?fileName=GrainandFeedAnnual_Pretoria_South Africa-Republic of_03-19-2020.
- van Antwerpen, R., M.C. Laker, D.J. Beukes, J.J. Botha, A. Collett, and M. du Plessis. 2021. Conservation agriculture farming systems in rainfed annual crop production in South Africa. South African Journal of Plant and Soil 38, no. 3: 202–216.
- Visser, M.H. 2014. Influence of cropping sequence on wheat production under conservation agriculture in the eastern free state. MS thesis. University of the Free State.
- Wang, G., R. Shi, L. Mi, and J. Hu. 2022. Agricultural eco-efficiency: Challenges and progress. Sustainability 14, no. 3.
- Weidema, B.P. 2009. Using the budget constraint to monetarise impact assessment results. Ecological Economics 68, no. 6: 1591–1598.
- Weidema, B.P. 2015. Comparing three life cycle impact assessment methods from an endpoint perspective. Journal of Industrial Ecology 19, no. 1: 20–26.
- Weidema, B.P., M. Wesnæs, J. Hermansen, T. Kristensen, N. Halberg, P. Eder, and L. Delgado. 2008. Environmental improvement potentials of meat and dairy products. JRC Scientific and Technical Reports.
- Wernet, G., C. Bauer, B. Steubing, J. Reinhard, E. Moreno-Ruiz, and B.P. Weidema. 2016. The ecoinvent database version 3 (part I): Overview and methodology. The International Journal of Life Cycle Assessment 21, no. 9: 1218–1230.
- Western Cape Department of Agriculture. 2021. Agricultural research data – cropping systems trials in Langgewens and Tygerhoek 2002–2020. Elsenburg: Western Cape Department of Agriculture.
Appendix
Table A1. List of inputs used per hectare in the Stepwise LCIA by location (Langgewens or Tygerhoek) and wheat production system (no-till, zero-till, or conventional till).
Table A2. Average annual wheat prices per ton adjust to 2020 ZAR by location and CA system extracted from Western Cape Department of Agriculture dataset (2021).
Table A3. Environmental damage by location and farming practice estimated using Stepwise LCIA for 1 kg of wheat produced in Western Cape, South Africa.