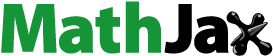
ABSTRACT
The effect of organic amendments on soil structure, microbial community and water transport in alpine ecosystems remains uncertain. Six fertilization regimes from a 2-year field experiment were selected to study this mechanism, i.e. plant litter (PL), organic fertilizer (OF), low concentration of glucose (LCG), medium concentration of glucose (MCG), high concentration of glucose (HCG) and the control (CT). Our results indicated that the soil macroporosity, branch density, length density and node density of soil macropores treated with OF, PL, HCG, MCG and LCG were larger than those of soils treated with CT. The phospholipid fatty acids (PLFAs), bacteria, fungi, actinomycetes, gram-positive bacteria (G+) and gram-negative bacteria (G−) of soils treated with OF, PL, HCG and MCG were larger than those of soils treated with LCG and CT. In contrast, the dyed area ratio and the maximum wetting depth of soils treated with OF, PL, LCG, HCG and MCG were smaller than those treated with CT. There were significant positive correlations between soil organic matter (SOM) and connectivity of soil macropores. Therefore, SOM accumulation significantly improved the soil pore structure, induced the development of the soil microbial community, increased the soil water retention ability and decreased the generation of preferential flow.
Introduction
Organic amendments typically enhance soil fertility by supplying organically complexed C, N and/or phosphorus, improving aggregate stability and stimulating soil organic carbon (SOC) mineralization and soil aggregation simultaneously (Bipfubusa et al. Citation2008; Abiven et al. Citation2009; Chivenge et al. Citation2011; Diacono and Montemurro Citation2011; Pronk et al. Citation2012; Waring et al. Citation2022). Studies have mainly focused on the effects of organic amendments on soil physical and chemical characteristics (Rumpel and Kögel-Knabner Citation2011; Krause et al. Citation2018; Xiao et al. Citation2019; Bierer et al. Citation2020, Citation2021; Majrashi et al. Citation2022). Some studies have shown that organic amendments alleviate soil degradation by improving the soil microbial community and enzymes (Ling et al. Citation2014; Ye et al. Citation2021). Wang et al. (Citation2021) indicated that organic amendments significantly modified the C dynamics, bacterial and fungal community compositions and network topological patterns in all aggregate sizes. Shu et al. (Citation2022) indicated that organic amendments significantly increased microbial diversity components and shifted microbial community structure compared to mineral-only fertilization. To date, researches on the effect of organic amendments on soil structure and water transport of alpine meadow soil on the Qinghai–Tibet Plateau are lacking.
Soil structure is the three-dimensional arrangement of solid soil constituents and voids across different scales (Rabot et al. Citation2018), and it results from interactions of biotic and abiotic factors, including climate, mineral composition, organic matter, roots, fungal hyphae, soil fauna and tillage. Studies have mainly focused on the effects of organic amendments on soil structure (Zaher et al. Citation2005; Hafida et al. Citation2007; Papadopoulos et al. Citation2009; Yilmaz and Sonmez Citation2017; Wan et al. Citation2020). The organic carbon from organic amendments acts as a binder, binding silt and clay particles together to form microaggregates (Six et al. Citation2004; Zhou et al. Citation2016). Previous studies have shown that soil organic matter (SOM) can also enhance aggregate stability by modifying aggregate pore space (Zaher et al. Citation2005; Hafida et al. Citation2007; Papadopoulos et al. Citation2009). However, the effect of organic amendment application on soil pore structure in alpine meadows is still largely neglected, especially in alpine soils. Soil pores affect the storage and transport of air, water and nutrients required for the survival of soil microbes, determining the distribution and composition of the soil microhabitat (Mummey and Stahl Citation2004; Bach et al. Citation2018), as well as the diffusion of substrates into microbial cells (Nunan et al. Citation2006). However, the effects of soil pore structure caused by SOM on soil microbial community and water transport in alpine ecosystems remain uncertain.
Organic amendments could change SOM by stimulating SOC mineralization (Pronk et al. Citation2012; Lal Citation2015). SOM is an important factor influencing soil pore structure and soil water transport (Dal Ferro et al. Citation2012, Citation2013; Zhou et al. Citation2013, Citation2022; Bell et al. Citation2023). Some studies have shown that SOM can promote the development of soil pore structure (Grosbellet et al. Citation2011; Gao et al. Citation2021). Grosbellet et al. (Citation2011) indicated that SOM leads to an increase in the soil macroporosity. Zhou et al. (Citation2013) used synchrotron X-ray microtomography and digital image analysis techniques to determine the microstructure of soils with different SOM contents and found that SOM improved the soil pore structure and increased soil pore connectivity. Dal Ferro et al. (Citation2013) found that SOM significantly increased the connectivity of soil pores and changed the distribution of soil pores from small to large pores, as evidenced by an increase in pores in the range of >560 μm and a decrease in pores in the range of 80–320 μm. Some studies have shown that SOM couldn’t change the soil pore structure (Deurer et al. Citation2009; Williams et al. Citation2017). Deurer et al. (Citation2009) found that the increase in SOM did not increase the proportion of large pores larger than 2 mm in diameter. Jarvis et al. (Citation2007) also found that soil organic carbon reduced preferential solute transport in cultivated topsoil. Gao et al. (Citation2021) indicated that the increase in SOM contributed to the improvement in soil water retention. Therefore, the effect of SOM on soil pore structure and soil water transport is still controversial and needs to be further investigated.
Alpine ecosystems are very sensitive to climate change and play an important role in Asia and even in global climate change (Qiu Citation2008). As one of the most important types of alpine grasslands, alpine meadows cover an area of 0.58 million km2. The mattic epipedon in alpine ecosystem plays a crucial role in soil water retention owing to its densely intertwined grass roots and high topsoil organic matter content (;; Zhi et al., Citation2017), in which soil organic matter content is up to about 10% (Kaiser, et al., Citation2008; Yang et al., Citation2017). The mattic epipedon layer plays an important role in maintaining the water-holding function of Tibetan plateau and alpine soils (Yang et al., Citation2017). Some studies have showed that SOM plays a dominant role in controlling soil water retention in this alpine meadow environment (Yang et al. Citation2014). Yang et al. (Citation2014) found that SOM affected soil water retention mainly through altering soil structural parameters and soil bulk density at high matric potentials, and the water adsorbing capacity of soil organic matter directly affected water retention at low matric potentials. However, whether the effect of SOM accumulation on soil pore structure and water distribution in alpine meadows is positive or negative, which needs to be studied thoroughly through experimental observations.
To fill the above knowledge gaps, we hypothesized that (1) organic amendments significantly improve the pore structure of alpine meadow soil, increasing the soil porosity, number of pores and connectivity; (2) SOM induced by organic amendments improves the soil water retention and reduced the preferential flow; and (3) soil macropore parameters induced by organic amendments are the main parameters influencing the soil microbial community.
Materials and methods
Study site and experimental design
This study was conducted in the watershed of the Shaliu River, the second largest river in the Qinghai Lake Watershed, situated in the northeastern of the Qinghai–Tibet Plateau, which lies in the semiarid, cold and high-altitude climate zone. Mean annual temperature and precipitation between 1957 and 2015 were 0.1°C and 400 mm in the watershed, respectively. Approximately 70%–80% of the annual precipitation occurs in summer and autumn. Winters are clear, dry and cold, and the summer is warm and wet. The soil type was classified as subalpine meadow soil, equivalent to Matti-Gelic Cambsols according to the FAO UNESCO system (USS Working Group WRB Citation2015).
In June 2019, the study area was divided into 18 plots, which were grouped randomly and supplemented with different organic amendments. There were three replicate groups for each treatment, and the plot size was 1.5 m × 1.5 m; the groups were as follows: 1) the control (CT); 2) organic fertilizer (OF, sheep dung, 1 kg m−2); 3) plant litter (PL, dead Kobresia pygmaea, 1 kg m−2); 4) low concentration of glucose (LCG, 0.5 kg m−2); 5) medium concentration of glucose (MCG, 1 kg m−2); and 6) high concentration of glucose (HCG, 2 kg m−2) (Fig. S1). All organic amendments were spread evenly on the soil surface layer based on the local fertilization practices. After all the plots were laid out, they were covered with a dust-proof net and enclosed with fences to prevent trampling by cattle and sheep (Fig. S2). The enclosed time was 2 years, and after application, the soil samples were collected in June 2021.
Soil sampling and analysis
The undisturbed soil samples using PVC pipes were collected from 18 plots after material application in June 2021. PVC pipe with a length of 30 cm and a diameter of 7.5 cm was selected for soil column sample collection, and the soil samples were completely filled into the PVC pipe during sampling. Core extraction followed the procedure of Sammartino et al. (Citation2012). The core was extracted by pushing, slowly and vertically, into the soil, the penetration of the cylinder progressing centimetre by centimetre, carefully removing the soil around the tube bottom at each step, to minimise mechanical stresses during extraction. After sampling, a PVC grid was glued to the base of the cylinder, and the cylinder was then glued to an annular sample holder to protect the bottom of the core from mechanical disturbance. To protect the samples during transport from field to laboratory, the cores were placed in sealed plastic bags and were wrapped with a sponge and packed with wheat straw. The soil samples for microbial determination were collected in the 0–10 cm and 10–20 cm layers, stored in a refrigerator. The samples were sieved (2 mm), and any visible roots and stones were manually removed. Then, the soil physical and chemical properties and phospholipid fatty acids (PLFAs) were analysed.
Disturbed soil samples for soil physical and chemical characteristics were collected at 0–10 cm, 10–20 cm and 20–30 cm (Wang and Hu Citation2023) with three replicates. The SOC content was measured by a carbon and nitrogen analyser (Nelson and Sommers Citation1996), and the obtained organic carbon content was multiplied by 1.724 to calculate the SOM content. Soil particle size analysis (soil texture) was performed by the pipet method (Gee and Bauder Citation1986). pH was determined following Thomas (Citation1996). The dry sieving method and the aggregate analyser (DIK–2012) were selected to measure the percentage of mechanically stable soil aggregates (Elliott Citation1986) and water – stable aggregates in the fractions of >2 mm, 1–2 mm, 0.5–1 mm, 0.25–0.5 mm, 0.1–0.25 mm and <0.1 mm, and the MWD and geometric mean diameter (GMD) of mechanically stable aggregates were calculated as follows (Tagar et al. Citation2020):
where Xi is the mean diameter of the aggregates sieved within the diameter range of i, and Wi is the percentage of the aggregate content sieved within the diameter range of i. The soil characteristics of study area are listed in .
Table 1. Physical and chemical properties of soil in the study area.
X-ray computed tomography scanning and image analysis
The scanning tool was the dual-ray source X-ray computed tomography system of NanoVoxel–5000 series produced by Tianjin Sanying Precision Instrument Limited Company. The undisturbed soil columns were scanned by X-ray computed tomography. The scanning parameters were as follows: the scanning voltage was 150 kV, the current was 250 μA, the exposure time was 0.1 s, and the scanning resolution was 68 μm.
The interpretation of soil macropores followed the procedure of Hu et al. (Citation2022) in Avizo 9.0 (FEI, 2016). A cylindrical cropping tool was applied to obtain a region of interest (ROI) to remove the cracks that formed on the margin of soil columns during sampling disturbances. The threshold value of soil macropores was selected according to the grey scale histogram (). The images were visually inspected to confirm the reasonability of the selected threshold value. The selected threshold value was then applied to the all slices of ROI to get binarization data. The soil macropore networks were visualized by volume rendering. Soil macropore parameters such as macroporosity, pore number, pore surface area, pore volume and pore equivalent diameter were calculated using the ‘Interactive Thresholding’ and ‘Label Analysis’ modules. The ‘Auto Skeleton’ module was selected to skeletonize and calculate the soil length density, node density, branch density, hydraulic radius and connectivity of the macropores.
PLFA analysis
A modified Bligh–Dyer technique was employed for soil PLFA extraction (Brant et al., Citation2006). Total lipids were extracted in a single-phase methanol–chloroform–phosphate buffer (2:1:0.8) system and separated into neutral lipids, glycolipids and polar lipids. Then, the polar lipids were trans-esterified, and the fatty acid methyl esters were separated, quantified and identified by capillary gas chromatography. Among them, methyl nondecanoate (19:0) was used as the internal standard. The calculations of PLFA, gram-positive bacteria (G+), gram-negative bacteria (G−), fungi, bacteria and actinomycetes followed the procedures of Sun et al. (Citation2011) and Bossio et al. (Citation2006).
Dyeing experiment
Dyeing experiments were carried out before and 1 year after the application of materials. In each plot, a 0.5 m × 0.5 m area was selected for the brilliant blue staining tracer experiment. An iron frame with a size of 0.5 m × 0.5 m × 0.3 m was placed approximately 15 cm into the soil. The staining tracer was a brilliant blue solution with a concentration of 3 g L−1. The 37.5 L of brilliant blue solution was mixed evenly and poured into the iron frame for infiltration. After 24 hours of infiltration, the soil profile was excavated in the plot to expose the staining area on the profile. The staining depth was measured with an iron ruler, and the area was photographed. Photoshop and MATLAB software were used to binarize the images and calculate the maximum dyeing depth and dyeing area ratio after processing.
Statistical analyses
All the statistical analyses were conducted using SPSS 20 (SPSS Inc., U.S.A.). Homogeneity of variances and normal distribution of the data were tested before applying the parametric methods. Differences between the macropore characteristics of different treatments were analysed by one-way analysis of variance (ANOVA), and differences were considered significant at the level of p < 0.05. Pearson correlations were conducted to evaluate the linkages among moss coverage, soil macropores and soil water retention.
Results
The effects of organic amendments on soil organic matter
shows the changes in the SOM content in response to OF, PL, LCG, MCG, HCG and CT in alpine meadows. At the soil depth of 0–10 cm, the contents of SOM were significantly greater in the soils treated with OF and HCG than those in the other treatments (p < 0.05), with values of 37.2 g kg−1 and 35.96 g kg−1, respectively, followed by the treatments with MCG (30.44 g kg−1), PL (30.08 g/kg) and LCG (29.14 g/kg). The SOM contents treated with CT were 27.13 g/kg, which were significantly lower than those of soils treated with OF, PL, LCG, MCG and HCG (p < 0.05).
Table 2. Soil organic matter (g/kg) under different treatments after 2 years.
At the soil depth of 10–20 cm, the SOM contents of the OF, HCG and PL treatments were significantly greater than those in the other treatments (p < 0.05), with values of 28.43 g kg−1, 27.32 g kg−1 and 27.01 g kg−1, respectively, followed by that in the LCG (23.96 g kg−1) and MCG (22.72 g kg−1) treatments. The lowest SOM content was found in the CT, with values of 21.14 g kg−1.
Overall, in the soil depth range of 0–30 cm, the SOM contents were significantly greater in the OF (29.99 g kg−1), HCG (29.67 g kg−1), PL (26.89 g kg−1), MCG (25.24 g kg−1) and LCG treatment (24.17 g kg−1) treatments than those in the treatment of CT (22.61 g kg−1) (p < 0.05).
The effects of organic amendments on soil structure
shows the 3D visualization of soil macropores treated with OF, PL, LCG, MCG, LCG and CT in alpine meadows. Soils of OF with the highest SOM contents had the high macropore number, the dense distribution and the best connectivity. Soils of CT had the low macropore number, the sparse distribution of pores and poor connectivity. The number and connectivity of pores in the treatment of HCG were significantly higher than those in the treatment of LCG (p < 0.05).
Figure 2. Three-dimensional visualization of soil macropores under different treatments (blue denotes soil macropores).

shows the characteristics of soil pore parameters after the application of OF, PL, LCG, MCG, HCG and CT. There were significant differences in the pore surface area density, pore number density, macroporosity, pore branching density, pore length density and pore node density among the different treatments (p < 0.05). The macropore surface area density of soils applied with the treatments of OF, PL, MCG and HCG (0.1241 mm2 mm−3, 0.0794 mm2 mm−3, 0.0653 mm2 mm−3 and 0.1039 mm2 mm−3) with the highest SOM contents was significantly greater than soils treated with CT and LCG (0.0503 mm2 mm−3 and 0.0573 mm2 mm−3) with lower SOM contents. The macroporosity, pore number density, branch density, length density, and node density applied with the treatment of OF, PL, LCG, MCG and HCG were significantly greater than that treated with CT (p < 0.05). The macroporosity (0.0092 mm3 mm−3) applied with the OF with the highest SOM contents was significantly greater than those treated with CT and LCG (0.0037 mm3 mm−3 and 0.0046 mm3 mm−3). The pore node density applied with the OF (1186.0533 no. mm−3) was significantly greater than that treated with CT and LCG (593.4897 no. mm−3 and 660.8383 no. mm−3) (p < 0.05).
Table 3. Soil macropore characteristics of all soil columns as determined using the X-ray computed tomography under different treatments.
The effects of organic amendments on the soil microbial community
The total PLFAs of soils applied with OF, PL, MCG and HCG were significantly greater than those of CT and LCG (, p < 0.05). There were significant differences in the total PLFAs between the CT and OF, MCG and HCG. There were no significant differences in the total PLFAs among the OF, PL, MCG and HCG. The total PLFAs were mostly distributed in the top 10 cm of soils under the OF and PL treatments ().
Figure 3. Total PLFAs, total bacteria, G+ bacteria, G− bacteria, fungi and actinomycetes under different treatments.

Table 4. Total phospholipid fatty acids (PLFAs), total bacterial, fungal, actinomycete, gram-positive bacterial and gram-negative bacterial contents under different treatments.
The bacteria, fungi, actinomycetes, G+ and G− of soils treated with OF, PL, MCG and HCG were larger than those of soils treated with CT and LCG (p < 0.05). The contents of total bacteria, fungi, actinomycetes, protozoa, G+ bacteria and G− bacteria were distributed in the deep soil layer among all treatments (). Significant differences in soil total PLFAs, bacteria, G+ and G− were observed between soils under CT and OF, PL, MCG and LCG (). Significant differences in soil total PLFAs, bacteria, fungi, actinomycetes, G+ and G− were observed between soils in the OF, PL and LCG.
The effects of organic amendments on soil water distribution
shows the dyed area and maximum dyed depth of alpine meadow soils treated with different organic amendments. In the range of 0–10 cm of soils, the dyed area of soils treated with OF, PL, LCG, MCG and LCG was significantly lower than soils treated with CT. At the depth of 10–20 cm, the dyed areas of soils treated with CT were significantly larger than soils treated with OF, PL, LCG, MCG and HCG. At the soil depth of 20–30 cm, soils treated with CT had the largest dyed area of 35% (p < 0.05). Overall, soils treated with CT had the largest dyed area of 65% in the 0–30 cm soil depth, which was significantly larger than soils treated with OF, PL and HCG.
Table 5. The dyed area ratio and the maximum wetting depth under different treatments.
Relationships between organic matter and soil structure, microbial community, and water transport
Strong correlations between SOM and surface area density, number density, macroporosity, branch density, length density, node density and connectivity of soil macropores were observed, as shown in . There were significant positive correlations between the SOM and surface area density, number density, macroporosity, branch density, length density, node density and connectivity of soil macropores. SOM was significantly and positively correlated with the MWD of dry aggregates, >0.25 mm dry aggregates, MWD of water-stable aggregates and >0.25 mm water-stable aggregates. The saturated water content was significantly and positively correlated with the content of SOM in soil depth of 0–30 cm ().
Table 6. Correlations between soil organic matter and soil structure (0–30 cm).
shows the relationships between the soil structure and the soil microbial community. The soil microbial community was significantly and positively correlated with the surface area density, number density, macroporosity, branch density, length density and node density of soil macropores. The ratio of G+/G− was significantly and negatively correlated with the surface area density, number density, macroporosity, branch density, length density and node density of soil macropores. Soil bulk density was significantly and negatively correlated with the contents of total PLFAs, bacteria and fungi.
Table 7. Correlations between the soil microbial community and soil pore structure.
Discussion
The results showed that the increase in the organic matter content of soils treated with organic amendments significantly improved the pore structure of alpine meadow soil, reduced the soil bulk density and increased the porosity, pore number and connectivity of the soil. These results are consistent with previous studies that showed a positive correlation between SOM content and soil pore space (Garcia-Orenes et al. Citation2005, Citation2013; Tejada and Gonzalez Citation2008). Garcia-Orenes et al. (Citation2005) indicated the application of biosolids increased significantly the organic carbon, carbohydrates and aggregate stability percentage, resulting in a decrease of the bulk density of soils. An increase in SOM content will improve soil structural stability, thus increasing soil porosity and aeration (Tejada and Gonzalez Citation2008). SOM will mix with denser mineral components in the soil, and soil bulk will decrease and porosity will increase due to the dilution effect of adding lower density organic matter to the denser mineral components (Chen et al., Citation2013). Moreover, microorganisms in the soil will improve soil structure by decomposing fresh organic matter, causing soil particles to aggregate and ultimately increasing soil pore connectivity (Zhou et al. Citation2016). This is consistent with our results, which showed that the total PLFAs, bacteria, fungi, actinomycetes, G+ and G− of soils treated with OF, PL, LCG, MCG and HCG were larger than soils treated with CT. Rabbi et al. (Citation2020) indicated that adding glucose to the soil increased the SOM content and found a significant increase in porosity and pore connectivity in glucose-amended soils. Our results also showed that SOM content was highly significantly and positively correlated with macroporosity, pore surface area density and pore branch density and significantly and positively correlated with pore number density, pore length density, pore node density and connectivity. Therefore, organic amendments significantly improved the pore structure of alpine meadow soil, increasing soil macroporosity, branch density, length density and node density.
The results showed that the macroporosity, branch density, length density and node density of soils treated with OF, PL, LCG, MCG and HCG were larger than those of soils treated with CT. Jarvis (Citation2007) observed that vertically convoluted and connected soil macropores facilitated the predominance of water transport through macropore infiltration. The connectivity of macropores enables them to conduct water, thus resulting in soil nutrient runoff (Pachepsky et al. Citation2000;). It is thus expected that water would travel more readily through the macropores in the soils treated with OF, PL, LCG, MCG and HCG than through those treated with CT. Therefore, it is expected that the soil water retention in the soils treated with OF, PL, LCG, MCG and HCG was smaller than that in soils treated with CT. This is consistent with our studies showing that the dyed area ratio and the maximum wetting depth of soils treated with OF, PL, LCG, MCG and HCG were smaller than those of soils treated with a CT. The increase in SOM content reduced the dyed area ratio and the maximum wetting depth, so that SOM significantly contributed to the water-holding capacity of the soil and weakened the generation of dominant soil flow. SOM possesses certain hydrophilic properties that can improve the ability to adsorb water (Yang et al. Citation2014). The results also showed that the saturated water content was significantly and positively correlated with the content of SOM (). Yang et al. (Citation2014) found that the extensive accumulation of SOM plays a dominant role in controlling soil water retention in this alpine environment by controlling water retention in alpine soils. Ebel (Citation2012) indicated that SOM was the main factor influencing the differences in soil water retention. Gao et al. (Citation2021) indicated that the increase in SOM due to shrub encroachment contributed to the improvement in soil water retention. Therefore, organic amendments significantly increased the soil water retention ability and reduced the generation of preferential soil flow in alpine meadows.
The results showed that the soil microbial community was significantly and positively correlated with the surface area density, number density, macroporosity, branch density, length density and node density of soil macropores. Moreover, the surface area density, number density, macroporosity, branch density, length density and node density of soil macropores were the main connectivity parameters (Hu et al. Citation2019). This is consistent with previous studies showing that the spatial distribution of soil microbes was affected by soil porosity and pore size (Kravchenko et al. Citation2014; Jien et al. Citation2021). Soil pores affect the storage and transport of air, water and nutrients required for the survival of soil microbes, determining the distribution and composition of the soil microhabitat (Mummey and Stahl Citation2004; Bach et al. Citation2018), as well as the diffusion of substrates into microbial cells (Nunan et al. Citation2006). Yoo et al. (Citation2006) pointed out that the pore size distribution could provide useful information for the mechanistic relationship between the soil structure and microbes. These results indicated that soil pore connectivity is a combination of many habitat attributes and can affect the structure and survival of the microbial community by changing the oxygen content (Kuikman et al. Citation1990; Young et al. Citation1998). Therefore, the soil macropore parameters (surface area density, number density, macroporosity, branch density, length density and node density) were the main parameters influencing the soil microbial community.
Conclusions
The findings of this study verified our hypothesis that organic amendments significantly improved the pore structure of alpine meadow soil, increasing soil porosity, number of pores and connectivity, and organic amendments improved the soil water retention and reduced the preferential flow. The soil macroporosity, branch density, length density and node density of soils treated with OF, PL, LCG, MCG and HCG were larger than those of soils treated with CT. The total PLFAs, bacteria, fungi, actinomycetes, G+ and G− of soils treated with OF, PL, LCG, MCG and HCG were larger than those of soils treated with CT. In contrast, the dyed area ratio and the maximum wetting depth of soils treated with OF, PL, LCG, MCG and HCG were smaller than those treated with CT. There were significant positive correlations between the content of SOM and surface area density, number density, macroporosity, branch density, length density, node density and connectivity of soil macropores. The content of SOM was significantly and positively correlated with the MWD of dry aggregates, >0.25 mm dry aggregates, MWD of water-stable aggregates and >0.25 mm water-stable aggregates. Soil macropore parameters (surface area density, number density, macroporosity, branch density, length density and node density) were the main parameters influencing the soil microbial community. Therefore, SOM accumulation significantly improved the soil pore structure, increased the soil water retention ability and reduced the generation of preferential soil flow.
Supplemental Material
Download PDF (373.5 KB)Disclosure statement
No potential conflict of interest was reported by the author(s).
Supplemental data
Supplemental data for this article can be accessed online at https://doi.org/10.1080/03650340.2023.2289513
Additional information
Funding
References
- Abiven S, Menasseri S, Chenu C. 2009. The effects of organic inputs over time on soil aggregate stability–A literature analysis. Soil Biol Biochem. 41(1):1–12. doi: 10.1016/j.soilbio.2008.09.015.
- Bach EM, Williams RJ, Hargreaves S, Yang F, Hofmockel KS. 2018. Greatest soil microbial diversity found in micro-habitats. Soil Biol Biochem. 118:217–226. doi: 10.1016/j.soilbio.2017.12.018.
- Bell SL, Zimmerman AE, Hofmockel KS. 2023. Cropping system drives microbial community response to simulated climate change and plant inputs. J Soil Water Conserv. 78(2):178–192. doi: 10.2489/jswc.2023.00069.
- Bierer AM, Maguire RO, Strickland MS, Stewart RD, Thomason WE. 2020. Evaluating effects of dairy manure application method on soil health and nitrate. Evaluating effects of dairy manure application method on soil health and nitrate. J Soil Water Conserv. 75(4):527–536. doi: 10.2489/jswc.2020.00074.
- Bierer AM, Maguire RO, Strickland MS, Stewart RD, Thomason WE. 2021. Manure injection alters the spatial distribution of soil nitrate, mineralizable carbon, and microbial biomass. J Soil Water Conserv. 76(2):175–189. doi: 10.2489/jswc.2021.00002.
- Bipfubusa M, Angers D, N’Dayegamiye A, Antoun H. 2008. Soil aggregation and biochemical properties following the application of fresh and composted organic amendments. Soil Sci Soc Am J. 72(1):160–166. doi: 10.2136/sssaj2007.0055.
- Bossio DA, Fleck JA, Scow KM, Fujii R. 2006. Alteration of soil microbial communities and water quality in restored wetlands. Soil Biol Biochem. 38(6):1223–1233. doi: 10.1016/j.soilbio.2005.09.027.
- Brant J B, Sulzman E W and Myrold D D. (2006). Microbial community utilization of added carbon substrates in response to long-term carbon input manipulation. Soil Biology and Biochemistry, 38(8), 2219–2232. 10.1016/j.soilbio.2006.01.022
- Chen Y, Ren C, Yang B, Peng Y and Dai C. (2013). Priming Effects of the Endophytic Fungus Phomopsis liquidambari on Soil Mineral N Transformations. Microb Ecol, 65(1), 161–170. 10.1007/s00248-012-0093-z
- Chivenge P, Vanlauwe B, Gentile R, Six J. 2011. Organic resource quality influences short-term aggregate dynamics and soil organic carbon and nitrogen accumulation. Soil Biol Biochem. 43(3):657–666. doi: 10.1016/j.soilbio.2010.12.002.
- Dal Ferro N, Berti A, Francioso O, Ferrari E, Matthews GP, Morari F. 2012. Investigating the effects of wettability and pore size distribution on aggregate stability: the role of soil organic matter and the humic fraction. Eur J Soil Sci. 63(2):152–164. doi: 10.1111/j.1365-2389.2012.01427.x.
- Dal Ferro N, Charrier P, Morari F. 2013. Dual-scale micro-CT assessment of soil structure in a long-term fertilization experiment. Geoderma. 204-205:84–93. doi: 10.1016/j.geoderma.2013.04.012.
- Deurer M, Grinev D, Young I, Clothier BE, Muller K. 2009. The impact of soil carbon management on soil macropore structure: a comparison of two apple orchard systems in New Zealand. Eur J Soil Sci. 60(6):945–955. doi: 10.1111/j.1365-2389.2009.01164.x.
- Diacono M, Montemurro F. 2011. Long-term effects of organic amendments on soil fertility. Agron Sustain Dev. 2:761–786.
- Ebel BA. 2012. Wildfire impacts on soil-water retention in the Colorado front range, United States. Water Resour Res. 48(12):1–12. doi: 10.1029/2012WR012362.
- Elliott ET. 1986. Aggregate structure and carbon, nitrogen, and phosphorus in native and cultivated soils. Soil Sci Soc Am J. 50(3):627–633. doi: 10.2136/sssaj1986.03615995005000030017x.
- Gao Z, Hu X, Li XY. 2021. Changes in soil water retention and content during shrub encroachment process in Inner Mongolia, northern China. Catena. 206:105528. doi: 10.1016/j.catena.2021.105528.
- Garcia-Orenes F, Guerrero C, Mataix-Solera J, Navarro-Pedreno J, Gomez I, Mataix-Beneyto J. 2005. Factors controlling the aggregate stability and bulk density in two different degraded soils amended with biosolids. Soil Tillage Res. 82(1):65–76. doi: 10.1016/j.still.2004.06.004.
- Gee GW, Bauder JW. 1986. Particle-size analysis. In: Klute A, editor Methods of soil analysis. Part 1. Agron. Monogr. 9. ASA and SSSA, Madison, WI. Part. Anal. p. 383–411. A. Klute methods soil anal. Part 1. 2nd ed. Agron. Monogr. 9. Madison, WI: ASA SSSA; pp. 383–411.
- Grosbellet C, Vidal-Beaudet L, Caubel V, Charpentier S. 2011. Improvement of soil structure formation by degradation of coarse organic matter. Geoderma. 162(1–2):27–38. doi: 10.1016/j.geoderma.2011.01.003.
- Hafida Z, Caron J, Angers DA. 2007. Pore occlusion by sugars and lipids as a possible mechanism of aggregate stability in amended soils. Soil Sci Soc Am J. 71(6):1831–1839. doi: 10.2136/sssaj2006.0257.
- Hu X, Li XY, Li ZC, Gao Z, Wu XC, Wang P, Lyu YL, Liu LY. 2019. Linking 3-D soil macropores and root architecture to near saturated hydraulic conductivity of typical meadow soil types in the Qinghai Lake watershed, northeastern Qinghai–Tibet Plateau. Catena. 185:104287. doi: 10.1016/j.catena.2019.104287.
- Hu X, Li X, Zhao Y, Cheng Y, Gao Z and Yang Z. (2022). Identification of water flow through non‐root soil macropores and along roots in shrub‐encroached grassland. European J Soil Science, 73(4), 10.1111/ejss.13260
- Jarvis NJ. 2007. A review of non-equilibrium water flow and solute transport in soil macropores: principles, controlling factors and consequences for water quality. Eur J Soil Sci. 58(3):523–546. doi: 10.1111/j.1365-2389.2007.00915.x.
- Jarvis NJ, Larsbo M, Roulier S, Lindahl A, Persson L. 2007. The role of soil properties in regulating non-equilibrium macropore flow and solute transport in agricultural topsoils. Eur J Soil Sci. 58(1):282–292. doi: 10.1111/j.1365-2389.2006.00837.x.
- Jien SH, Kuo YL, Liao CS, Wu YT, Igalavithana AD, Tsang DCW, Ok YS. 2021. Effects of field scale in situ biochar incorporation on soil environment in a tropical highly weathered soil. Environ Pollut. 272:116009–7491. doi: 10.1016/j.envpol.2020.116009.
- Kaiser K, Miehe G, Barthelmes A, Ehrmann O, Scharf A, Schult M, Schlütz F, Adamczyk S and Frenzel B. (2008). Turf-bearing topsoils on the central Tibetan Plateau, China: Pedology, botany, geochronology. CATENA, 73(3), 300–311. 10.1016/j.catena.2007.12.001
- Krause HM, Hüppi R, Leifeld J, El-Hadidi M, Harter J, Kappler A, Hartmann M, Behrens S, M¨ader P, Gattinger A. 2018. Biochar affects community composition of nitrous oxide reducers in a field experiment. Soil Biol Biochem. 119:143–151. doi: 10.1016/j.soilbio.2018.01.018.
- Kravchenko AN, Negassa WC, Guber AK, Hildebrandt B, Marsh TL, Rivers ML. 2014. Intra-aggregate pore structure influences phylogenetic composition of bacterial community in macroaggregates. Soil Sci Soc Am J. 78(6):1924–1939. doi: 10.2136/sssaj2014.07.0308.
- Kuikman P, Van Elsas J, Jansen A, Burgers S, Van Veen J. 1990. Population dynamics and activity of bacteria and protozoa in relation to their spatial distribution in soil. Soil Biol Biochem. 22(8):1063–1073. doi: 10.1016/0038-0717(90)90031-T.
- Lal R. 2015. Restoring soil quality to mitigate soil degradation. Sustainability. 7(5):5875–5895. doi: 10.3390/su7055875.
- Ling N, Sun Y, Ma J, Guo J, Zhu P, Peng C, Yu G, Ran W, Guo S, Shen Q. 2014. Response of the bacterial diversity and soil enzyme activity in particle-size fractions of mollisol after different fertilization in a long-term experiment. Biol Fertil Soils. 50(6):901–911. doi: 10.1007/s00374-014-0911-1.
- Majrashi MA, Obour AK, Moorberg CJ, Lollato RP, Holman JD, Du J, Mikha MM, Assefa Y. 2022. No-tillage and fertilizer-nitrogen improved sorghum yield in dryland wheat–sorghum–fallow rotation. J Soil Water Conserv. 77(6):609–618.
- Mummey DL, Stahl PD. 2004. Analysis of soil whole- and inner-microaggregate bacterial communities. Microb Ecol. 48(1):41–50. doi: 10.1007/s00248-003-1000-4.
- Nelson DW, Sommers LE. 1996. Total carbon, organic carbon, and organic matter. In: Bartels JM Bigham JM, editors Methods of soil analysis part 3—chemical methods. Madison WI., USA: Soil Science Society of America; pp. 961–1010.
- Nunan N, Ritz K, Rivers M, Feeney DS, Young IM. 2006. Investigating microbial micro-habitat structure using X-ray computed tomography. Geoderma. 133(3–4):398–407. doi: 10.1016/j.geoderma.2005.08.004.
- Pachepsky Y, Rawls W, Timlin D. 2000. A one-parameter relationship between unsaturated hydraulic conductivity and water retention. Soil Sci. 165(12):911–919. doi: 10.1097/00010694-200012000-00001.
- Papadopoulos A, Bird NR, Whitmore AP, Mooney SJ. 2009. Investigating the effects of organic and conventional management on soil aggregate stability using X-ray computed tomography. Eur J Soil Sci. 60(3):360–368. doi: 10.1111/j.1365-2389.2009.01126.x.
- Pronk GJ, Heister K, Ding GC, Smalla K, Kogel-Knabner I. 2012. Development of biogeochemical interfaces in an artificial soil incubation experiment; aggregation and formation of organo-mineral associations. Geoderma. 189-190:585–594. doi: 10.1016/j.geoderma.2012.05.020.
- Qiu J. 2008. China: the third pole. Nature. 454(7203):393–396. doi: 10.1038/454393a.
- Rabbi SMF, Minasny B, McBratney AB, Young LM. 2020. Microbial processing of organic matter drives stability and pore geometry of soil aggregates. Geoderma. 360:114033. doi: 10.1016/j.geoderma.2019.114033.
- Rabot E, Wiesmeierb M, Schlütera S, Vogela HJ. 2018. Soil structure as an indicator of soil functions: a review. Geoderma. 314:122–137. doi: 10.1016/j.geoderma.2017.11.009.
- Rumpel C, Kögel-Knabner I. 2011. Deep soil organic matter—a key but poorly understood component of terrestrial C cycle. Plant Soil. 338(1–2):143–158. doi: 10.1007/s11104-010-0391-5.
- Sammartino S, Michel E and Capowiez Y. (2012). A Novel Method to Visualize and Characterize Preferential Flow in Undisturbed Soil Cores by Using Multislice Helical CT. Vadose Zone Journal, 11(1), 10.2136/vzj2011.0100
- Shu XY, He J, Zhou ZH, Xia LL, Hu YF, Zhang YY, Luo YQ, Chu HY, Liu WJ, Yuan S, et al. 2022. Organic amendments enhance soil microbial diversity, microbial functionality and crop yields: a meta-analysis. Sci Total Environ. 829:154627. doi: 10.1016/j.scitotenv.2022.154627.
- Six J, Bossuyt H, Degryze S, Denef K. 2004. A history of research on the link between (micro) aggregates, soil biota, and soil organic matter dynamics. Soil Tillage Res. 79(1):7–31. doi: 10.1016/j.still.2004.03.008.
- Sun Y, Wu J, Shao Y, Zhou L, Mai B, Lin Y, Fu S. 2011. Responses of soil microbial communities to prescribed burning in two paired vegetation sites in southern China. Ecol Res. 26(3):669–677. doi: 10.1007/s11284-011-0827-2.
- Tagar AA, Adamowski J, Memon MS, Do MC, Mashori AS, Soomro AS, Bhayo WA. 2020. Soil fragmentation and aggregate stability as affected by conventional tillage implements and relations with fractal dimensions. Soil Tillage Res. 197:104494. doi: 10.1016/j.still.2019.104494.
- Tejada M, Gonzalez JL. 2008. Influence of two organic amendments on the soil physical properties, soil losses, sediments and runoff water quality. Geoderma. 145(3–4):325–334. doi: 10.1016/j.geoderma.2008.03.020.
- Thomas GW. 1996. Soil pH and soil acidity. In: Sparks DL, editor Methods of soil analysis. Part 3, chemical methods. Madison, WI: SSSA Book Series 5; pp. 475–490.
- USS Working Group WRB. 2015). World reference base for soil resources 2014, update 2015: international soil classification system for naming soils and creating legends for soil maps. (World Soil Resources Rep. 106). FAO.
- Wang XY, Bian Q, Jiang YJ, Zhu LY, Chen Y, Liang YT, Sun B. 2021. Organic amendments drive shifts in microbial community structure and keystone taxa which increase C mineralization across aggregate size classes. Soil Biol Biochem. 153:108062. doi: 10.1016/j.soilbio.2020.108062.
- Wang R, Hu X. 2023. Pore structure characteristics and organic carbon distribution of soil aggregates in alpine ecosystems in the Qinghai Lake basin on the Qinghai-Tibet Plateau. Catena. 231:107359. doi: 10.1016/j.catena.2023.107359.
- Wan W, Li X, Han S, Wang L, Luo X, Chen W, Huang Q. 2020. Soil aggregate fractionation and phosphorus fraction driven by long-term fertilization regimes affect the abundance and composition of P-cycling-related bacteria. Soil Tillage Res. 196:104475. doi: 10.1016/j.still.2019.104475.
- Waring E, Licht M, Ripley E, Staudt A, Carlson S, Helmers M. 2022. Cover crop mixtures versus single species: water quality and cash crop yield. J Soil Water Conserv. 78(1):1–15. doi: 10.2489/jswc.2023.00174.
- Williams A, Davis AS, Jilling A, Grandy AS, Koide RT, Mortensen DA, Smith RG, Snapp SS, Spokas KA, Yannarell AC. 2017. Reconciling opposing soil processes in row-crop agroecosystems via soil functional zone management. Agr Ecosyst Environ. 236:99–107. doi: 10.1016/j.agee.2016.11.012.
- Xiao ZG, Rasmann S, Yue L, Lian F, Zou H, Wang ZY. 2019. The effect of biochar amendment on N-cycling genes in soils: a meta-analysis. Sci Total Environ. 696:133984. doi: 10.1016/j.scitotenv.2019.133984.
- Yang F, Huang L M, Rossiter D G, Yang F, Yang R M and Zhang G L. (2017). Evolution of loess‐derived soil along a climatic toposequence in the Qilian Mountains, NE Tibetan Plateau. European J Soil Science, 68(3), 270–280. 10.1111/ejss.12425
- Yang F, Zhang GL, Yang JL, Li DC, Zhao YG, Liu F, Yang RM, Yang F. 2014. Organic matter controls of soil water retention in an alpine grassland and its significance for hydrological processes. J Hydrol. 519:3086–3093. doi: 10.1016/j.jhydrol.2014.10.054.
- Ye GP, Banerjee S, He JZ, Fan JB, Wang ZH, Wei XY, Hu HW, Zheng Y, Duan CJ, Wan S, et al. 2021. Manure application increases microbiome complexity in soil aggregate fractions: results of an 18-year field experiment. Agr Ecosyst Environ. 307:107249. doi: 10.1016/j.agee.2020.107249.
- Yilmaz E, Sonmez M. 2017. The role of organic/bio–fertilizer amendment on aggregate stability and organic carbon content in different aggregate scales. Soil Tillage Res. 168:118–124. doi: 10.1016/j.still.2017.01.003.
- Yoo GY, Nissen TM, Wander MM. 2006. Use of physical properties to predict the effects of Tillage Practices on organic matter dynamics in three Illinois soils. J Environ Qual. 35(4):1576–1583. doi: 10.2134/jeq2005.0225.
- Young IM, Blanchart E, Chenu C, Dangerfield M, Fragoso C, Grimaldi M, Ingram J, Monrozier LJ. 1998. The interaction of soil biota and soil structure under global change. Glob Chang Biol. 4(7):703–712. doi: 10.1046/j.1365-2486.1998.00194.x.
- Zaher H, Caron J, Ouaki B. 2005. Modeling aggregate internal pressure evolution following immersion to quantify mechanisms of structural stability. Soil Sci Soc Am J. 69(1):1–1. doi: 10.2136/sssaj2005.0001.
- Zhi J, Zhang G, Yang F, Yang R, Liu F, Song X, Zhao Y and Li D. (2017). Predicting mattic epipedons in the northeastern Qinghai-Tibetan Plateau using Random Forest. Geoderma Regional, 10 1–10. 10.1016/j.geodrs.2017.02.001
- Zhou H, Fang H, Mooney SJ, Peng X. 2016. Effects of long-term inorganic and organic fertilizations on the soil micro and macro structures of rice paddies. Geoderma. 266:66–74. doi: 10.1016/j.geoderma.2015.12.007.
- Zhou X, Li C, Wu L, Lv J, Xie X, Wei J, Zhang Y. 2022. Responses of soil water retention to climate and underlying condition changes in river source areas: a case study of the Hei river basin and Bai river basin, China. J Soil Water Conserv. 77(6):555–567.
- Zhou H, Peng XH, Perfect E, Xiao TQ, Peng GY. 2013. Effects of organic and inorganic fertilization on soil aggregation in an Ultisol as characterized by synchrotron based X-ray micro-computed tomography. Geoderma. 195-196:23–30. doi: 10.1016/j.geoderma.2012.11.003.