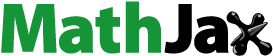
ABSTRACT
Although significant differences in soil nitrogen levels exist under different paddy-upland rotations, the main reason for this is unclear. The nitrogen retention capacity and loss of ammonia volatilisation, leaching, etc. of paddy soil with large differences in nitrogen levels from two long-term rotations, garlic-rice and wheat-rice, were measured using the soil column simulation method. The results showed that the loss rate of leaching was only 5.4%, whereas that of ammonia volatilisation was up to 22.8%, which was the main nitrogen loss way of paddy soil under the two rotations. The average ammonia volatilisation rates under wheat-rice rotation with high and low nitrogen application rates were 12.1% and 40.2% higher than that under garlic-rice rotation, leading to a decrease in the total nitrogen loss amount and rate through ammonia volatilisation by 29.8% and 8.8%, respectively. As a result, nitrogen retention in the soil under garlic-rice rotation increased by 12.7%. In conclusion, the long-term garlic-rice rotation could significantly inhibit ammonia volatilisation, thus improving the soil nitrogen retention capacity. The straw return may increase soil organic matter content, reduce ammonia volatilisation loss, and enhance soil nitrogen retention capacity and productivity.
Introduction
Approximately 15% of the total nitrogen fertilisers used in agriculture are applied to rice production alone, but the nitrogen utilisation rate of paddy fields is only 30%–35% in China (Heffer Citation2009). Most nitrogen fertilisers applied to the soil enter the environment through ammonia volatilisation, leaching, runoff, denitrification, and other losses, resulting in water and air pollution (Chen et al. Citation2014). Soil is an essential medium for rice roots to absorb nitrogen fertilisers applied to paddy fields. However, although many nitrogen fertilisers are applied to paddy fields, 50%–80% of the nitrogen taken up by rice plants comes from the soil itself (Zhu Citation1985). Therefore, it is important to reduce nitrogen loss and improve soil nitrogen retention capacity to maintain soil productivity and crop yield. Soil chemical and biological properties, such as organic matter, cation exchange capacity, pH, urease activity, and the abundance of ammonia-oxidizing bacteria and archaea, could affect the fate of nitrogen in soil (Kuypers et al. Citation2018; Li et al. Citation2020; Zhang et al. Citation2022).
Paddy-upland rotation is a good choice for major grain-producing areas to ensure global food security with continuous decreases in arable land and increasing populations, especially in Asia (Alberto et al. Citation2014; Zheng et al. Citation2016). In different paddy-upland rotations, different types and cultivation methods of upland crops and frequent cycling between flooding and drying affect the soil physicochemical and biological properties of paddy fields (Nishida Citation2016; Liu et al. Citation2022a), thereby influencing soil nitrogen retention capacity and productivity. For example, crops, such as legumes and green manures, in rotation with rice are beneficial to the sustainable production of the system, whereas long-term wheat-rice rotation (WR) results in a decrease in soil fertiliser and rice yield, which is closely related to the circulation and utilisation of soil nitrogen (Ladha et al. Citation2003; Zhou et al. Citation2021).
The results of our long-term positioning experiment showed that the soil productivity of paddy fields under WR was significantly lower than that under garlic-rice rotation (GR), and a large amount of nitrogen applied in the garlic growth season was retained in the soil and absorbed by the rice, thus significantly improving nitrogen recovery efficiency and yield in the rice season (Zhou et al. Citation2020). Therefore, we hypothesised that nitrogen losses would be significantly reduced under long-term GR, thus improving soil nitrogen retention capacity. However, the difference in the soil nitrogen retention capacity of paddy-upland rotations from the perspective of nitrogen loss pathways has not been reported. Therefore, based on an 8-year location experiment, soils with significant differences in productivity from long-term WR and GR were selected as the research object. The objective of this study was to evaluate the effects of different long-term paddy-upland rotations on soil nitrogen retention capacity and to reveal the main reasons for the improvement of soil nitrogen retention through nitrogen loss. The results of this study will be of great significance for optimising annual nitrogen management and improving the productivity of paddy-upland rotations.
Materials and methods
Experimental soil and experiment design
A long-term location experiment of GR and WR was conducted during the 2014 dry season in the modern agriculture research and development base of Sichuan Agricultural University, Chongzhou City, Sichuan Province, China (30°33′N, 103°38′E). The cultivation measures and management of these three crops are listed in . The 0–20 cm arable layer soil from the location experiment was collected after harvesting the dry season garlic and wheat crops in May 2021, which was the 8th cycle of the two rotations. Eight sub-samples were collected from each cropping system, and 5 kg of each soil sample was taken back to the laboratory to prepare the test soil column after mixing well. The device soil column, with a height of 30 cm and an inner diameter of 5 cm, was made of acrylic material. The experimental soil was beaten into the mud under simulated field production conditions. A filter cloth, quartz sand, experimental soil, and water were sequentially inserted into the device. It was ensured that the height of the soil column was 20 cm and the water layer was 5 cm after standing for 48 h. The total nitrogen content, bulk density, and weight of the GR soil column were 1.945, 1.039 g/cm3, and 408 g/column, respectively, and those of the WR soil column were 1.712, 1.123 g/cm3, and 441 g/column, respectively.
Table 1. Cultivation and management measures for different crops under long-term garlic-rice and wheat-rice rotation.
The experiment was performed using a two-factor randomised design with two different tested soils from the location experiment (GR and WR) and two nitrogen application rates, with three replications. Therefore, there were four treatments and 12 test soil columns. The two nitrogen application rates were 76.8 and 38.4 mg urea column−1, equivalent to paddy applications of 180 and 90 kg N ha−1, defined as high nitrogen (HN) and low nitrogen (LN) application rates, respectively. Nitrogen fertiliser was applied as the soil column was stable.
Indices and measurement methods
Nitrogen leaching
A laboratory simulation of the soil column was used to measure nitrogen leaching (Luo et al. Citation2021). A measuring cup was placed at the bottom of the device to collect the leachate. Samples were taken on the 1st, 2nd, 3rd, 4th, 5th, 6th, 7th, 10th, 13th, 16th, 19th, 23th, 26th, 29th, and 32th day after fertilisation. Water layers of all treatments were added to a depth of 5 cm every 2–3 days, depending on liquid leaching. The concentrations of ammonium and nitrate nitrogen were determined using indophenol blue colorimetry and ultraviolet spectrophotometry, respectively (Bao Citation2000).
Ammonia volatilization
Nitrogen loss through ammonia volatilisation was measured using the in-situ ventilation method (Wang et al. Citation2004; Chen et al. Citation2013a). One piece of round sponge (6 cm in diameter and 2 cm in thickness) was immersed in 10 mL of glycerol and phosphoric acid (prepared by combining 50 mL of phosphoric acid and 40 mL of glycerol and diluting to 1 L with pure water) was placed at the top of the nozzle of the leaching device immediately after fertilisation to absorb ammonia volatilised from the soil surface. The sample collection period was the same as mentioned above in the “Nitrogen leaching”. The collected sponges were taken to the laboratory and extracted with 100 mL of 1 M KCl for one hour, and 2–10 mL of the extraction solution was used to determine the ammonium concentration.
Nitrogen retention in soil
Soil samples were collected before insertion into the device and after the last leaching sampling. The total nitrogen content of the soil was determined using the Kieldahl method, and the soil weight of each device was measured after drying. The amount of nitrogen retained in the soil was calculated as follows:
where NSR is the amount of nitrogen retention in the soil, m is the soil weight, and N%AE and N%BE are the total nitrogen content of the soil after and before the experiment, respectively.
Nitrogen loss from the other pathway
The amount of nitrogen loss was calculated using the subtraction method as follows:
where NO is the amount of nitrogen lost in other ways, NF is the amount of nitrogen applied, NSR is the amount of nitrogen retention in soil, and NL and NA are the amounts of nitrogen lost by leaching and ammonium volatilisation, respectively.
Data analysis
All data acquired in this study were subjected to analysis of variance and comparisons of treatments, followed by least significant difference multiple range tests, using SPSS 19.0. Plots and curve fitting were conducted using Microsoft Excel 2016 and JMP Pro 16 to assess the differences and tendencies among the means of the different treatments.
Results
Soil nitrogen retention capacity
The loss and retention of nitrogen were affected by the cropping system and the nitrogen application rate (). The higher the nitrogen application rate, the higher the nitrogen loss rate. Compared with WR, the total nitrogen loss amount and loss rate decreased 11.3%–17.4% and 5.0%–8.5%, respectively, resulting in the amount of nitrogen retention in soil increased by 16.6% and 9.0% under GR with HN and LN treatments, respectively. The results showed that the soil nitrogen retention capacity increased under long-term GR.
Figure 1. Soil nitrogen retention capacity under different nitrogen application rates and paddy-upland rotations.
Notes: GR: soil from the long-term garlic-rice rotation, WR: soil from the long-term wheat-rice rotation, HN: high nitrogen application (76.8 mg urea column−1, equivalent to paddy applications of 180 kg N ha−1), LN: low nitrogen application (38.4 mg urea column-1, equivalent to paddy applications of 90 kg N ha−1). The different lowercases indicate significant difference of different treatments at 0.05 probability level according to LSD.

Nitrogen loss through different pathways
Nitrogen loss through ammonia volatilization
The ammonia volatilisation rate with HN was 2.3 and 1.8 times higher than that with LN under GR and WR, respectively (). Under different treatments, the ammonia volatilisation rate first increased and then decreased with the increase in fertilisation days, and ammonia volatilisation mainly occurred within one week after fertilisation. Under GR, the ammonia volatilisation rate significantly decreased after 10 d of fertilisation, and the average value was only 60.6 uġd−1. Under WR, the ammonia volatilisation rate stabilised below 100 uġd−1 after 20 d of fertilisation. During the experimental period, the average ammonia volatilisation rates under WR with HN and LN were 12.1% and 40.2% higher than those under GR, respectively. As a result, the total nitrogen loss amount and rate through ammonia volatilisation under GR decreased by 29.8% and 8.8%, respectively, compared to WR.
Figure 2. Ammonia volatilisation rate and loss amount under different nitrogen application rates and paddy-upland rotations.
Notes: GR: soil from the long-term garlic-rice rotation, WR: soil from the long-term wheat-rice rotation, HN: high nitrogen application (76.8 mg urea column−1, equivalent to paddy applications of 180 kg N ha−1), LN: low nitrogen application (38.4 mg urea column−1, equivalent to paddy applications of 90 kg N ha−1).

Nitrogen loss through leaching
The leachate volume was mainly affected by different rotations, and the total leachate volume under GR was 2.0 times that under WR, while the concentration of nitrate-nitrogen was mainly affected by the nitrogen application rate, and the average concentration of nitrate-nitrogen with NH was 15.9% higher than that with LN (). The daily leaching loss under the two rotations increased with increasing nitrogen application rate (). More leaching loss occurred 2–3 weeks after fertilisation, as the daily loss of leaching presented a parabolic trend with an increase in fertilisation days. The cumulative loss amount through leaching of different treatments increased rapidly at first and then slowly increased during the experimental period and increased with the increase in nitrogen application rate. The total loss amount of leaching under GR was 1.8–2.0 times compared to that under WR. These results demonstrate that the variation in leachate volume is the main reason for the difference in nitrogen leaching losses between the GR and WR.
Figure 3. Difference in daily leachate volume and nitrate nitrogen concentration under different nitrogen application rates and paddy-upland rotations.
Notes: GR: soil from the long-term garlic-rice rotation, WR: soil from the long-term wheat-rice rotation, HN: high nitrogen application (76.8 mg urea column−1, equivalent to paddy applications of 180 kg N ha−1), LN: low nitrogen application (38.4 mg urea column−1, equivalent to paddy applications of 90 kg N ha−1).

Figure 4. Difference in nitrogen leaching loss under different nitrogen application rates and paddy-upland rotations.
Notes: GR: soil from the long-term garlic-rice rotation, WR: soil from the long-term wheat-rice rotation, HN: high nitrogen application (76.8 mg urea column−1, equivalent to paddy applications of 180 kg N ha−1), LN: low nitrogen application (38.4 mg urea column−1, equivalent to paddy applications of 90 kg N ha−1).

Nitrogen loss through other pathways
The amount of nitrogen lost through other pathways increased with an increase in the nitrogen application rate (). The loss rates of nitrogen through other pathways were 16.8%–19.9% and 11.0%–12.1% with HN and LN, respectively. Compared with WR, the amount and rate of nitrogen loss through other pathways under GR decreased by 15.8% and 3.1%, respectively, with HN and decreased by 9.0% and 1.1%, respectively, with LN. Combined with the results of ammonia volatilisation and leaching, it can be concluded that the reduction in ammonia volatilisation was the main reason for the improvement in soil nitrogen retention capacity under GR.
Table 2. Soil nitrogen loss pathways and loss rates under different nitrogen application rates and paddy-upland rotations.
Discussion
It is necessary to reduce nitrogen loss from the soil to ensure soil nitrogen nutrient levels and productivity. Leaching nitrogen from denitrification is one of the main pathways of nitrogen loss in farmland ecosystems. As nitrate is negatively charged and hardly absorbed by soil colloids, nitrate-nitrogen is easily lost to the environment through leaching, resulting in water pollution (Zhao et al. Citation2011; Gao et al. Citation2016; Li et al. Citation2016). Nitrification is necessary for the conversion of both organic nitrogen and applied nitrogen fertiliser (urea) to nitrate. However, the anaerobic environment created by paddy flooding inhibited nitrifying bacteria and nitrification (Cameron et al. Citation2013), which may have led to a slight difference in nitrate-nitrogen concentration in the leachate (). The volume of leaching under GR was approximately two-fold that under WR (), which is closely related to soil porosity. Mulching rice straw after sowing is a conventional technique for garlic planting in GR, and the decomposed humus promotes soil porosity (Turmel et al. Citation2015; Abdelrhman et al. Citation2021). The leaching rate increased with increasing soil porosity, resulting in more nitrogen loss through leaching under GR ().
However, the loss rate of each route showed that ammonia volatilisation was the most critical pathway for nitrogen loss, and the amount of ammonia volatilisation in WR increased by 42.32% compared with that in GR (), which demonstrated that ammonia volatilisation was the main reason for the difference in soil nitrogen retention capacity between GR and WR. Straw mulching during the garlic growth season not only has the good insulation and moisturising effect, but also can improve soil fertility and inhibit soil ammonia volatilisation (Zhang et al. Citation2014; Zhao et al. Citation2022; Liu et al. Citation2022b). Crop straw contains abundant cellulose, which can adsorb cations (Rahman and Islam Citation2009). A large amount of humus is formed during straw decomposition, which enhances the adsorption of ammonium ion (Chen et al. Citation2013b; Li et al. Citation2018). Furthermore, long-term straw return enhances the adsorption and fixation of ammonium ions in the soil by increasing the soil’s organic carbon content (Zhang et al. Citation2022). Through a comprehensive analysis of the effects of soil properties on ammonia volatilisation, Duan and Xiao (Citation2000) proposed that increasing the soil organic matter content is a feasible means of reducing ammonia volatilisation.
In conclusion, although garlic-rice rotation with rice straw mulching increased nitrogen leaching loss due to the increase in leachate volume, the nitrogen loss reduced by inhibiting ammonia volatilisation was much higher than the leaching loss, which resulted in a decrease in the total nitrogen loss rate and an increase in nitrogen retention in soil under garlic-rice rotation. This study revealed the reasons for the difference in soil nitrogen retention capacity of different paddy-upland rotations through nitrogen loss, and the interaction between leaching and ammonia volatilisation could be studied in-depth in the future to guide for further reducing nitrogen loss.
Disclosure statement
No potential conflict of interest was reported by the author(s).
Additional information
Funding
Notes on contributors
Wei Zhou
Wei Zhou holds a doctorate in Agriculture majoring in Crop Cultivation and Farming System, with more than 10 years of Research Experience in nitrogen utilization of Paddy-upland rotation.
Yanqiu Chen
Yanqiu Chen, Zhitao Hu, and Yihong Kuang, are Undergraduate majoring in agriculture, and interested in soil nitrient cycling.
Yu Fan
Yu Fan is Graduate student majoring in Crop Cultivation and Farming System, He has research interests in efficient and safe production of rice.
Tao Wang
Tao Wang is a postdoctor majoring in Crop Cultivation and Farming System, is devoted to the study of green production and emission reduction of rice-base cropping system.
Yong Chen
Youfeng Tao and Hong Cheng engaged in rice field production and experimental management.
Fei Deng
Yong Chen, Fei Deng, and Xiaolong Lei, are Associate Professor, are devoted to the study of green, efficient, high quality, high yield and mechanized production of rice-base cropping system.
Jianfeng Hu
Jianfeng Hu holds a doctorate in Agriculture majoring in Crop Cultivation and Farming System, is a assistant dean of Sichuan Agricultural University, and committed to rural revitalization.
Wanjun Ren
Wanjun Ren is a Professor and division chief of Sichuan Agricultural University, with more than 10 years of Research Experience in the green, efficient, high quality, high yield and mechanized production of rice.
References
- Abdelrhman AA, Gao L, Li S, Lu JJ, Song X, Zhang M, Zheng F, Wu H, Wu X. 2021. Long-term application of organic wastes improves soil carbon and structural properties in dryland affected by coal mining activity. Sustainability. 13:5686. doi:10.3390/su13105686.
- Alberto MCR, Quilty JR, Buresh RJ, Wassmann R, Haidar S, Correa TQ, Sandro JM. 2014. Actual evapotranspiration and dual crop coefficients for dry-seeded rice and hybrid maize grown with overhead sprinkler irrigation. Agr Water Manage. 136:1–12. doi:10.1016/j.agwat.2014.01.005.
- Bao SD. 2000. Soil agrochemical analysis. Beijing: China Agricultural Press.
- Chen DJ, Jiang LN, Huang H, Toyota K, Dahlgren RA, Lu J. 2013a. Nitrogen dynamics of anaerobically digested slurry used to fertilize paddy fields. Biol Fert Soils. 49:647–659. doi:10.1007/s00374-012-0752-8.
- Chen HH, Li XC, Hu F, Shi W. 2013b. Soil nitrous oxide emissions following crop residue addition: a meta-analysis. Global Change Biol. 19:2956–2964. doi:10.1111/gcb.12274.
- Chen XP, Cui ZL, Fan MS, Vitousek P, Zhao M, Ma W, Wang Z, Zhang W, Yan X, Yang J, et al. 2014. Producing more grain with lower environmental costs. Nature. 514:486–489. doi:10.1038/nature13609.
- Cameron KC, Di HJ, Moir JL. 2013. Nitrogen losses from the soil/plant system: a review. Ann Appl Biol. 162:145–173. doi:10.1111/aab.12014.
- Duan Z, Xiao H. 2000. Effects of soil properties on ammonia volatilization. Soil Sci Plant Nutr. 46:845–852.
- Gao SS, Xu P, Zhou F, Yang H, Zheng CM, Cao W, Tao S, Piao SL, Zhao Y, Ji XY. 2016. Quantifying nitrogen leaching response to fertilizer additions in China’s cropland. Environ Pollut. 211:241–251. doi:10.1016/j.envpol.2016.01.010.
- Heffer P. 2009. Assesment of fertilizer use by crop at the global level 2006/07-2007/08. Paris: IFA.
- Kuypers MMM, Marchant HK, Kartal B. 2018. The microbial nitrogen-cycling network. Nat Rev Microbiol. 16:263–226. doi:10.1038/nrmicro.2018.9.
- Ladha JK, Dawe D, Pathak H, Padre A, Yadav RL, Singh B, Singh Y, Singh P, Kundu AL, Sakal R, et al. 2003. How extensive are yield declines in long-term rice–wheat experiments in Asia? Field Crops Res. 81:159–180. doi:10.1016/S0378-4290(02)00219-8.
- Li YL, Kronzucker HJ, Shia WM. 2016. Microprofiling of nitrogen patches in paddy soil: analysis of spatiotemporal nutrient heterogeneity at the microscale. Sci Rep. 6:27064. doi:10.1038/srep27064.
- Li MN, Wang YL, Adeli A, Yan HJ. 2018. Effects of application methods and urea rates on ammonia volatilization, yields and fine root biomass of alfalfa. Field Crop Res. 218:115–125. doi:10.1016/j.fcr.2018.01.011.
- Li Z, Zeng Z, Tian D, Wang J, Wang B, Chen HYH, Quan Q, Chen W, Yang J, Meng C, et al. 2020. Global variations and controlling factors of soil nitrogen turnover rate. Earth-Sci Rev. 207:103250. doi:10.1016/j.earscirev.2020.103250.
- Liu Y, Wang KK, Liao SP, Ren T, Li XK, Cong RH, Lu JW. 2022a. Differences in responses of ammonia volatilization and greenhouse gas emissions to straw return and paddy-upland rotations. Environ Sci Pollut Res. 29:25296–25307. doi:10.1007/s11356-021-17239-2.
- Liu SW, Wang M, Yin M, Chu G, Xu C, Zhang X, Abliz B, Tang C, Wang D, Chen S. 2022b. Fifteen years of crop rotation combined with straw management alters the nitrogen supply capacity of upland-paddy soil. Soil Till Res. 215:105219. doi:10.1016/j.still.2021.105219.
- Luo W, Qian L, Liu W, Zhang X, Wu Z. 2021. A potential Mg-enriched biochar fertilizer: excellent slow-release performance and release mechanism of nutrients. Sci Total Environ. 768:144454. doi:10.1016/j.scitotenv.2020.144454.
- Nishida M. 2016. Decline in fertility of paddy soils induced by paddy rice and upland soybean rotation, and measures against the decline. Jpn Agr Res Q. 50:87–94. doi:10.6090/jarq.50.87.
- Rahman MS, Islam MR. 2009. Effects of pH on isotherms modeling for Cu(II) ions adsorption using maple wood sawdust. Chem Eng J. 149:273–280. doi:10.1016/j.cej.2008.11.029.
- Turmel MS, Speratti A, Baudron F, Verhulst N, Govaerts B. 2015. Crop residue management and soil health: a systems analysis. Agric Syst. 134:6–16. doi:10.1016/j.agsy.2014.05.009.
- Wang ZH, Liu XJ, Ju XT, Zhang FS, Malhi SS. 2004. Ammonia volatilization loss from surface-broadcast urea: comparison of vented- and closed-chamber methods and loss in winter wheat–summer maize rotation in north China plain. Commun Soil Sci Plant. 35:2917–2939. doi:10.1081/CSS-200036499.
- Zhang HL, Lal R, Zhao X, Xue JF, Chen F. 2014. Chapter one-opportunities and challenges of soil carbon sequestration by conservation agriculture in China. Adv Agron. 124:1–36. doi:10.1016/B978-0-12-800138-7.00001-2.
- Zhang WZ, Chen XQ, Wang HY, Wei WX, Zhou JM. 2022. Long-term straw return influenced ammonium ion retention at the soil aggregate scale in an Anthrosol with rice-wheat rotations in China. J Integr Agr. 21:521–531. doi:10.1016/S2095-3119(20)63592-4.
- Zhao BQ, Li XY, Liu H, Wang BR, Zhu P, Huang SM, Bao DJ, Li YT, So HB. 2011. Results from long-term fertilizer experiments in China: the risk of groundwater pollution by nitrate. NJAS-Wagen J Life Sci. 58:177–183. doi:10.1016/j.njas.2011.09.004.
- Zhao ZX, Wang XY, Tian YJ, Wang R, Peng Q, Cai HJ. 2022. Effects of straw returning on soil ammonia volatilization under different production conditions based on meta-analysis. Environ Sci. 43:1678–1687. doi:10.13227/j.hjkx.202107086.
- Zheng H, Huang H, Zhang G, Li J. 2016. National-scale paddy-upland rotation in Northern China promotes sustainable development of cultivated land. Agr Water Manage. 170:20–25. doi:10.1016/j.agwat.2016.01.009.
- Zhu, Z.L., 1985. Progress in the study of soil nitrogen supply and fate of fertilizer nitrogen in China. Soil. 17:2–9. https://kns.cnki.net/KCMS/detail/detail.aspx?dbname=cjfd1985&filename=tura198506009&dbcode=cjfq.
- Zhou W, Wang T, Fu Y, Yang Z, Liu Q, Yan F, Chen Y, Tao Y, Malik N, Ren W. 2020. Residual nitrogen from preceding garlic crops is important for double-cropped rice. Nutr Cycl Agroecosyst. 118:311–324. doi:10.1007/s10705-020-10099-1.
- Zhou Y, Xu L, Xu Y, Xi M, Tu D, Chen J, Wu W. 2021. A meta-analysis of the effects of global warming on rice and wheat yields in a rice–wheat rotation system. Food Energy Secur. 10. doi:10.1002/fes3.316.