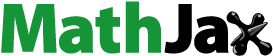
ABSTRACT
Justify that the use of selenium has been shown to promote seed germination and seedling growth of some crops under salt stress but the physiological mechanisms in sorghum remain poorly understood. Herein, grain sorghum [Sorghum bicolor (L.) Moench] variety Jinza 2002 was used to explore the effects and mechanisms of seed priming with 25 μmol/L sodium selenite [Se(IV)] on seed germination, seedling growth, antioxidant enzyme activity and osmotic regulatory substances under 120 mmol/L NaCl. The results showed that soaking sorghum seeds in Se(IV) effectively alleviated the harm of salt stress. Compared with the Na group, Se(IV) application increased germination percentage (by 23.8%), while facilitating the elongation of radicles (by 4.2%) and plumules (by 18.4%) under salt stress. Salt-stressed seedlings showed increased levels of leaf chlorophyll, soluble sugar, soluble protein and proline contents, and antioxidant enzyme activities, as well as root activity, and total biomass following Se(IV) application, along with decreased relative electrical conductivity and malondialdehyde content in the leaves. Findings of this study indicate that seed priming with Se(IV) facilitates sorghum seed germination and seedling growth under salt stress by improving root activity, enhancing leaf photosynthesis and antioxidant responses, and reducing cell membrane damage.
Introduction
Soil salinisation is a process in which salts from the soil or groundwater rise to the surface with capillary water, which evaporate, causing salts to accumulate in the surface soil. Soil salinisation is a worldwide environmental issue restricting agricultural production, its obvious effect on plants is to inhibit the growth and differentiation of plant tissues and organs, resulting in plant growth retardation. Exploitation of salinised land resources and crop production in saline soils are therefore essential for China to achieve food security.
During plant growth, crops are most sensitive to salt stress at the germination and seedling stages. When salt stress is encountered at these two sensitive stages, the number of seedlings per unit area and crop yield are dramatically reduced. In addition, Lei et al. (Citation2022) showed that the germination percentage of buckwheat seeds decreased under higher salt stress conditions. Consequently, enhancing seed germination and seedling emergence under salt stress is a prerequisite for the rational utilisation of saline soils and the improvement of crop productivity in salinised farmland.
Salt stress affects the physiological functions of crop plants because it inhibits plant water transport, leading to osmotic stress and water deficiency. Plants have developed multiple endogenous mechanisms, such as enzymatic and non-enzymatic antioxidant systems to deal with environmental stress but these mechanisms are often insufficient under high-stress levels. The application of exogenous substances, such as osmotic protectants, antioxidants, polyamines and microelements, can effectively increase plant stress resistance (Perveen et al. Citation2018). As an antioxidant protector, selenium (Se) has a dual effect on plant growth and development. Low concentrations of Se benefit plants by enhancing antioxidant enzyme activity whereas high concentrations of Se pose a toxic effect on plants (Siddiqui et al. Citation2021). Therefore, appropriate Se concentrations should be applied to alleviate salt stress in plants.
Different methods are available for exogenous Se application at various plant growth stages. For example, Nemat Alla et al. (Citation2020) showed that soaking wheat seeds with 15 µM sodium selenite (Se(IV)) enhanced plant resistance to salt stress by up-regulating the expression of antioxidants and Na+-manipulating genes. Liang (Citation2020) showed that low concentrations (0.1–4 μM) of Se(VI or VI) soaking wheat seeds under salt stress could improve the germination percentage and germination index of durum wheat seeds, alleviate the degree of membrane lipid peroxidation, reduce reactive oxygen species (ROS) accumulation, maintain a higher photosynthetic rate of leaves and improve the adaptability of durum wheat under salt stress. In addition, Rasool et al. (Citation2020) added 200 mM NaCl into Hoagland’s nutrient solution to simulate salt stress, and found that supplementation with 1 µM Se substantially increased the photosynthetic pigment and the relative water content in millet leaves, reduced cell membrane damage, while facilitating the growth of millet seedlings and biomass accumulation. Furthermore, Desoky et al. (Citation2021) found that three foliar sprays of 8 µM Se (25, 40 and 55 days after sowing, respectively) alleviated the adverse effects of salt stress on wheat crops by enhancing photosynthetic efficiency, antioxidant defense system and osmotic metabolism.
Sorghum [Sorghum bicolor (L.) Moench) is a potential crop in saline soils because of its high salt and alkali resistance, outstanding photosynthetic performance and adaptation to drought and poor soil fertility. The salt stress threshold refers to the critical concentration at which plant growth is significantly inhibited. Liu et al. (Citation2016) showed that 150 mmol/L NaCl was the salt stress threshold for sweet sorghum and 50 mmol/L NaCl was the salt stress threshold for spring wheat during the seed germination stage. The salt stress threshold of oats (Li et al. Citation2018) was 0.6–0.8% NaCl. Zhang et al. (Citation2021) concluded that 0.6% salt concentration was optimal for the identification of salt tolerance in the whole growth period of rice. The responses of sorghum plants to salt stress have been investigated with respect to the screening of salt-tolerant materials, the identification of salt-resistance genes and the analysis of physio-biochemical changes. Bao et al. (Citation2020) indicated that dry seedling weight and fresh root weight can be used to evaluate salt tolerance in a large number of sorghum varieties. Zhu et al. (Citation2018) showed that seed priming with exogenous growth-regulating substances (gibberellin and γ-aminobutyric acid) improved the ability of sweet sorghum to resist salt stress. Moreover, Zhang et al. (Citation2020) found that the salt stress resistance of a salt-tolerant sorghum genotype, Bayeqi, was improved owing to the enhanced activity of antioxidant enzymes and increased contents of osmoregulatory substances. Despite the accumulating knowledge of salt stress responses in sorghum, the effects and mechanisms of seed priming with Se on crop germination and initial growth in this species under salt stress are still poorly understood.
Exogenous selenium can effectively reduce the adverse effects of salt stress, such as water potential reduction and ion toxicity, by regulating ion balance, improving the activity of antioxidant enzymes and increasing the content of osmotic regulatory substances, and it significantly enhances the salt tolerance of plants. The present study investigated the changes in seed germination and seedling growth of sorghum under salt stress after seed priming with selenite [Se(IV)]. The aim of the study was to decipher the physiological role of Se(IV) in alleviating the adverse effects of salt stress on sorghum germination and growth. The results could contribute to efficient utilisation of salinised land and sustainable sorghum production in saline soils.
Materials and methods
Experimental materials
The grain sorghum (S. bicolor) variety Jinza 2002 used in this study was bred by Sorghum Research Institute of Shanxi Agricultural University (Jinzhong, Shanxi Province, China). Sodium selenite (Na2SeO3, analytically pure) and sodium chloride (NaCl, analytically pure) were purchased from Shandong Xiya Chemical Industry Co., Ltd. (Linyi, Shandong Province, China).
Experimental design and setup
A salt solution was prepared from NaCl and distilled water with a concentration of 120 mmol/L. The Se(IV) solution used to soak seeds was prepared from Na2SeO3 and distilled water with a concentration of 25 μmol/L. The concentrations of salt and Se(IV) used in the experiments were determined by pre-experiment tests. Seed germination and seedling growth experiments were respectively conducted in Petri dishes and pots with four treatments and three replications: (i) no NaCl or Na2SeO3 (control group), (ii) 25 μmol/L Na2SeO3 (Se group), (iii) 120 mmol/L NaCl (Na group) and (iv) 120 mmol/L NaCl + 25 μmol/L Se (Se + Na group). On April 15, 2022, the Petri dish experiment was performed in a light incubator at 25 ± 1°C with a 12:12-h day/night cycle to determine the effects of Se(IV) treatment on seed germination under salt stress. Briefly, sorghum seeds of uniform size (approx. 500 seeds) were disinfected with a 75% alcohol solution for 5 min and then rinsed with distilled water three times. Seeds were surface sterilised with 0.1% HgCl2 solution (w/v) for 3 min and then rinsed with sterilised distilled water. After the surface water was carefully absorbed with filter papers, the seeds were soaked in the Se(IV) solution or distilled water at 25°C temperature under dark conditions. Twelve hours later, the seeds were washed with distilled water three times, and the excess water was removed with a paper towel. Twenty-one seeds were placed on a 9-cm-diameter Petri dish, and 10 mL of the salt solution or distilled water was then added. The salt solution was exchanged every 24 h to maintain the NaCl concentration.
The pot experiment was carried out In a greenhouse at 25/15 ± 2°C day/night to explore the effects of Se(IV) treatment on seedling growth under salt stress. 12-cm-high and 13-cm-diameter pots were filled with 1 kg of mixed substrate (soil: sand = 2:1, w/w). Ten Se(IV) or distilled water-treated seeds per pot were sown evenly at a depth of 4 cm on April 19, 2022. Four and eight days later, each pot received 60 mL of the salt solution and distilled water, respectively.
Seed germination and plant growth measurements
Seed germination (i.e. emergence of radicles to a 0.5 cm length) and seedling emergence (growth stage 11 in Biologische Bundesanstalt, Bundessortenamt and Chemical Industry scale) were observed and recorded daily. The radicle and plumule lengths of germinated seeds were measured in Petri dishes on day 8 using a Vernier caliper. The fresh weight of radicles and plumules was also determined, followed by decolorisation at 105°C and oven-drying at 65°C until constant weight; then the dry weight was recorded. In addition, the height and leaf area of seedlings were measured using a tape measure, and their fresh weight and dry weight were determined 14 days after sowing.
In the pot experiment, fresh leaf samples of whole plant were collected 10, 12 and 14 days after sowing to measure the contents of osmoregulatory substances (soluble sugar, soluble protein and proline), malondialdehyde content and antioxidant enzyme activities (superoxide dismutase, peroxidase, catalase). Soluble sugar content was measured spectrophotometrically at 620 nm according to Li (Citation2000) using glucose as a standard. Soluble protein content was determined using Coomassie Brilliant Blue assay. Bovine serum albumin was used as the standard and the absorbance was recorded at 595 nm (Li Citation2000). Proline content was determined by ninhydrin assay. Toluene was used as the standard and the absorbance was recorded at 520 nm (Li Citation2000).
Fresh leaves (500 mg) were homogenised with 5 mL of 100 mM phosphate buffer (pH 7.0) using a mortar and pestle on ice. The homogenate was centrifuged at 15,000×g for 10 min at 4°C. The supernatant was used to determine the activity of superoxide dismutase (SOD), peroxidase (POD) and catalase (CAT). SOD activity was measured by nitro blue tetrazolium (NBT) reduction; one unit of SOD was defined as the amount of enzyme required to cause 50% reduction of NBT as recorded at 560 nm (Zou Citation2000). POD activity was measured using a guaiacol assay (Zou Citation2000); in the presence of H2O2, the rate of guaiacol oxidation recorded at 470 nm reveals the enzyme activity. CAT activity was measured according to the method of Aebi (Citation1984); the determination was based on the decrease of H2O2 absorbance at 240 nm.
Malondialdehyde (MDA) content was analysed by thiobarbituric acid assay (Zou Citation2000). Fresh leaves (100 mg) were homogenised with 1% trichloroacetic acid on ice. The homogenate was centrifuged at 12,000×g for 15 min at 4°C, 0.5% thiobarbituric acid was added to the supernatant and the mixture was reacted at 95°C for 30 min, cooled in an ice bath, and recentrifuged for 10 min. The absorbance of the supernatant was read at 532 and 600 nm.
In the pot experiment, fresh leaf and root samples were collected 14 days after sowing to analyse the following parameters. The measurement of relative electrical conductivity was conducted using a DDS-11A conductivity meter (Enfan Instrument Equipment Co., Ltd., HeFei, ShanDong, China). Fresh leaves (0.1 g) were soaked in 10 mL of deionised water for 12 h. The conductivity of the extract (R1) was measured with a conductivity meter, and then the extract (R2) was measured again after heating in a boiling water bath for 30 min.
Chlorophyll (a, b) contents were determined by spectrophotometric assay after extraction with 95% ethanol (Li Citation2000). Fresh leaves (0.1 g) were finely ground in 3 mL 95% ethanol and sieved using filter paper (1825-090, Whatman®, England). The absorbance was determined at 663 and 645 nm. Total chlorophyll was the sum of chlorophyll a and chlorophyll b content.
Root activity was determined by triphenyl tetrazolium chloride (TTC) reduction assay (Zou Citation2000). A 10-mL mixture of 0.4% TTC solution and pH 7.0 phosphoric acid buffer (1:1 volume ratio) was added to 0.5 g of fresh roots. After holding in the dark at 37°C for 1 h, 2 mL of 1 mol/L sulphuric acid was added and the mixture was allowed to stand for 10 min. The root was taken out and ground with 4 mL ethyl acetate, then adjusted to 10 mL with ethyl acetate. The absorbance was recorded at 485 nm.
Statistical analysis
Data are presented as the means ± standard deviation (n = 3). Microsoft Excel 2010 (Microsoft Corp., Redmond, WA, USA) was used to collate the experimental data. SPSS 23.0 software (IBM Corp., Armonk, NY, USA) was used for one-way analysis of variance, significantly different means between treatments were compared at p < .05 by Duncan’s multiple range test.
Results
Effect of seed priming with Se(IV) on sorghum seed germination under salt stress
Seed germination percentage
The germination percentage of sorghum seeds was lower under salt stress than under non-saline conditions on the third day of sowing, whereas seeds priming with Se(IV) showed considerably improved germination percentage and enhanced elongation of radicles and plumules (A). Specifically, compared with the control group (93.7%), the seed germination percentage increased by 1.8% in the Se group, while the seed germination percentage decreased by 28.1% (p < .05) in the Na group. Seed priming with Se(IV) effectively increased the germination percentage by 23.8% (p < .05) in the Se + Na group compared with the Na group, although the parameter values were still lower than those under normal conditions (B).
Figure 1. Picture depicting the third day of seed germination (A) and histogram depicting the seventh-day germination percentage (B) of sorghum seeds treated with and without Se(IV) under salt stress. CK, control with no NaCl or Na2SeO3; Se, treatment with 25 μmol/L Na2SeO3; Na, treatment with 120 mmol/L NaCl; and Se + Na, treatment with 120 mmol/L NaCl + 25 μmol/L Se. Error bars represent standard deviation of the mean (n = 3). Different letters above error bars indicate significant differences among the treatments at the 0.05 level.

Radicle and plumule growth
Salt stress exhibited a distinct inhibitory effect on the radicle and plumule elongation of sorghum seeds (A). The radicle length was 14.6 cm in the control group, which had no significant difference compared with 15.8 cm in the Se group (B). The radicle length significantly decreased to 9.4 and 9.8 cm in the Na and Se + Na groups (p < .05), respectively. Similar trends were observed for plumule length under various treatments (control: 7.9 cm, Se: 8.4 cm, Na: 3.9 cm and Se + Na: 4.6 cm).
Figure 2. Radicle and plumule growth (A) and length (B) of sorghum seeds under various treatments. Abbreviations are defined in caption. Error bars represent standard deviation of the mean (n = 3). Different letters above error bars indicate significant differences among the treatments at the 0.05 level.

The fresh and dry weights of radicles and plumules in the control group were 359.3, 734.8, 53.8 and 80.8 mg, respectively. The fresh weight of radicles and plumules remarkably increased by 11.4% and 18.8% in the Se group, respectively, compared with those of the control group (p < .05); the respective dry weight increased slightly, but not significantly (4.0% and 8.7%, respectively). Both the fresh and dry weights of radicles and plumules were significantly lower in the Na group than in the control and Se groups (p < .05). Following Se(IV) application, the fresh weight of radicles and plumules significantly increased by 35.2% and 21.7%, respectively, in the Se + Na group compared with those of the Na group (p < .05) but the parameter values were always lower than those under normal conditions. The dry weight of radicles and plumules in the Se + Na group also increased compared with those of the Na group, albeit not significantly (14.3% and 17.2%, respectively; ).
Table 1. Radicle and plumule biomass of sorghum seeds under different treatments.
Effect of seed priming with Se(IV) on sorghum seedling growth under salt stress
Seedling growth performance
Compared with the control group (8.2 cm), there was a 36.3% increase in the plant height of seedlings in the Se group (p < .05), whereas a 40.0% decrease occurred in plant height in the Na group. The plant height significantly increased by 52.4% (p < .05) in the Se + Na group compared with that of the Na group, but the parameter value was still significantly lower than those under normal conditions.
Leaf area (p < .05), fresh weight and dry weight (p < .05) of seedlings increased under the Se treatment compared with those of the control group (Leaf area: 2.5 cm2, fresh weight: 104.5 mg and dry weight: 9.1 mg), whereas the three seedling parameters significantly decreased (p < .05) under the Na treatment (). Compared with the Na treatment, the fresh weight and dry weight of seedlings under the Se + Na treatment increased by 61.4% and 56.0% (p < .05), respectively, along with a modest, insignificant increase in leaf area (28.7%).
Table 2. Growth performance of sorghum seedlings under different treatments at 14 days after sowing.
Osmoregulation and cell membrane damage
Irrespective of salt stress and/or Se(IV) application, the soluble sugar content in sorghum leaves increased from day 10 to day 12, followed by a decrease on day 14 after sowing. The trend of soluble sugar content among different treatment groups was consistent between 10 and 14 days after sowing (A). For example, on day 14, the soluble sugar content of leaf samples in the Se group was 39.3 mg/g, only 7.5% higher than that of control samples (36.6 mg/g). The soluble sugar content of leaf samples in the Na group was 41.4 mg/g, which was significantly higher than that of control samples (p < .05). The soluble sugar content of leaf samples further increased by 10.0% in the Se + Na group compared with that of the Na group (p < .05). The soluble protein content in sorghum leaves exhibited similar trends as soluble sugar content with time and among the four treatment groups (B). For example, on day 14 after sowing, the soluble protein content in leaf samples of the Se and Na groups increased by 5.5% and 20.0% (p < .05), respectively, compared with those of control samples (17.2 mg/g). The soluble protein content in leaf samples of the Se + Na group further increased by 4.3% (p < .05) compared with that of the Na group.
Figure 3. Soluble sugar, soluble protein, proline and malondialdehyde (MDA) contents in the leaves of sorghum seedlings. Abbreviations are defined in caption. Error bars represent standard deviation of the mean (n = 3). Different letters above error bars indicate significant differences among the treatments at the 0.05 level.

In all cases, the proline content of seedling leaves increased from day 10 to day 14 after sowing and a similar trend was observed among various treatments (C). Compared with the control group (10d: 97.8 μg/g, 12d:123.4 μg/g and 14d: 153.2 μg/g), the leaf proline content of the Se group increased by 16.4, 1.8 and 1.2% at the three time points, respectively. More pronounced increase was observed in the leaf proline content of the Na group by 20.8% (p < .05), 10.75% and 11.2%, respectively. The leaf proline content of the Se + Na group increased by 11.0, 2.3 and 2.5% at 10, 12 and 14 days, respectively compared with that of the Na group. However, in the two latter dates (12 and 14 days after sowing), differences among the four treatments were not statistically significant.
The MDA content in seedling leaves also increased from day 10 to day 14 after sowing, and the trend of changes among different treatment groups was almost the same at the three time points (D). For example, on day 14, the leaf MDA content was lowest in the Se group (4.3 µmol/g) compared with other groups (control: 5.5 µmol/g, Na: 6.8 µmol/g; and Se + Na: 6.1 µmol/g). The leaf MDA content decreased by 20.7% in the Se group compared with that of the control group (p < .05). The MDA content of leaf samples in the Na group was significantly higher than those of CK and Se groups (p < .05). There was a 9.6% decrease in the leaf MDA content of the Se + Na group compared with that of the Na group.
Antioxidant enzyme activities
The antioxidant enzyme activities of seedling leave increased first and then decreased from day 10 to day 14, with consistent trend of changes among various treatment groups (). For example, on day 14, the leaf SOD, POD and CAT activities were all higher in the Se group than in the control group (SOD:163.7 U/(g min) protein, POD: 51.2 U/(g min) protein and CAT: 8.2 U/(g min) protein) under normal conditions, with 10.8, 6.0 and 2.7% increase, respectively. The SOD, POD and CAT activities were enhanced by 25.2, 54.5 and 52.7%, respectively, in the Na group compared with the control group (p < .05). Furthermore, under the Se + Na group, the SOD, POD and CAT activities significantly increased by 10.8, 16.6 and 17.8% (p < .05), respectively, compared with those of the Na group.
Figure 4. Antioxidant enzyme activities (superoxide dismutase, peroxidase, catalase) in leaves of sorghum seedlings. SOD, superoxide dismutase; POD, peroxidase; CAT, catalase. Abbreviations are defined in caption. Error bars represent standard deviation of the mean (n = 3). Different letters above error bars indicate significant differences among the treatments at the 0.05 level.

Relative electrical conductivity, photosynthetic pigments and root activity
The relative electrical conductivity of leaf samples was lowest in the Se group among the different treatments (A). Compared with the control group (18.5%), the leaf relative electrical conductivity in the Se group decreased by 15.6% (p < .05), whereas that of the Na group increased by106.5% (p < .05). In the Se + Na group, the relative electrical conductivity decreased by 21.9% (p < .05) compared with that of the Na group.
Figure 5. Relative electrical conductivity (A), photosynthetic pigment content (B) and root activity (C) of sorghum seedlings. Abbreviations are defined in caption. Error bars represent standard deviation of the mean (n = 3). Different letters above error bars indicate significant differences among the treatments at the 0.05 level.

The total chlorophyll content in leaf samples of the Se group increased by 3.8% (p < .05) compared with that of the control group (chlorophyll a: 0.8 mg/g, chlorophyll b: 1.1 mg/g, total chlorophyll: 1.9 mg/g; B). Specifically, there was a 5.8% (p < .05) increase in the chlorophyll a content and a 2.2% increase in the chlorophyll b content. The chlorophyll contents in leaf samples of the Na group decreased significantly (chlorophyll a: −13.8%, chlorophyll b: −19.7% and total chlorophyll: −17.2%; p < .05) compared with that of the control group. Furthermore, the total chlorophyll content of leaf samples significantly increased (3.4%; p < .05) in the Se + Na group compared with the Na group, although the difference in the content of chlorophyll a (3.1%) or b (3.6%) was not significant. The total chlorophyll content of leaf samples in the Se + Na group was still lower than those of the Se and control groups (p < .05).
Root activity indicates the ability of plants to absorb water and nutrients in the soil. Among the various treatments, sorghum seedlings showed the highest root activity in the Se group (control: 66.5 μg/(g h), Se: 82.8 μg/(g h), Na: 38.7 μg/(g h) and Se + Na: 46.1 μg/(g h); C). Compared with the control group, the root activity of seedlings in the Se group increased by 24.6% (p < .05), while that of the Na group decreased by 41.8% (p < .05). The root activity of seedlings in the Se + Na group increased by 19.1% (p < .05) compared with that of the Na group.
Discussion
Seed germination percentage directly affects crop yield because seed germination is the first step for crop growth. In saline soils, high salinity is a major limiting factor for crop seed germination. In the present study, we used 120 mmol L–1 NaCl to simulate salt stress. It was found ( and ) that under the stress condition, the grain sorghum variety Jinza 22 showed a lower seed germination percentage with shorter radicles and plumules than under normal conditions. Seed priming with Se(IV) increased the germination percentage of sorghum seeds under salt stress. Zhu et al. (Citation2018) also showed that the seed germination potential and germination percentage of sweet sorghum varieties Guotian 2011 and Guotian 106 decreased by 32–50% under 100 mmol L–1 salt stress.
It was found ( and ) that the radicle and plumule elongation of sorghum seeds primed with 25 μmol/L Se(IV) was higher than that of sorghum seeds primed without Se(IV) under salt stress, and the fresh and dry weights of radicles and plumules also showed similar trends, which is consistent with the findings of foxtail millets (Rasool et al. Citation2020). Previous studies have shown that plant cells cannot absorb sufficient water from the external environment due to salinity stress-induced water potential reduction of the growing medium; this water deficit stress or osmotic effect of salinity disturbs normal metabolic activities, blocks cell division and differentiation, and thus inhibits seed germination. Seed priming with Se(IV) maintained the cell membrane stability, the water environment required for starch hydrolysis and enzyme activation during seed germination, thereby ensuring normal seed germination.
Morphological parameters are sensitive indicators of plant stress levels. It was found () that seed priming with Se(IV) not only promoted the growth of sorghum seedlings under non-stress conditions but also induced an increase in the plant height, leaf area and biomass of sorghum seedlings under salt stress. A previous study indicated that adding 25 μmol/L Na2SeO3 to the nutrient solution of tomato colonisation alleviated the adverse effect of salt stress on the growth of germinating tomato, leading to an increase in the stem diameter and plant height (Fan Citation2022). Under NaCl-induced salt stress, the seed germination percentage, root length and germ length of mustard plants supplied with nano-Se markedly increased compared with those of no-Se group (Dev and Chandra Citation2022). Taken together, the results demonstrate that seed priming with Se(IV) can enhance the growth and development of crop seedlings by alleviating salt stress and improving salt resistance.
Salt stress adversely affects plant metabolism, mainly through osmotic stress and ion toxicity (Zeng et al. Citation2015). Many studies have shown that the accumulation of some sugars can mitigate the adverse effects of salt stress on plants by increasing the osmotic potential (Hare et al. Citation1998). It has been shown that in Arabidopsis and banana seedlings, salt stress can upregulate the gene expression of SWEET and subsequently promote the transport of soluble sugars and improve the resistance of plants to salt stress (Breia et al. Citation2021). It has also been shown that salt stress-upregulated salt tolerance genes can increase the endogenous levels of glucose, sucrose and fructose by regulating the biosynthesis of soluble sugars as a form of carbon storage and to exercise osmotic protective functions, thereby protecting the protein biosynthesis system from salt stress damage and improving the resistance of the plant to salt stress (Rosa et al. Citation2009; Wang et al. Citation2021). Wang et al. (Citation2022a) showed that salt-tolerant inbred line maize enhances osmoregulation ability in seedlings by increasing the content of soluble protein compared with salt-sensitive inbred line maize, which is beneficial for maintaining the normal morphological structure and metabolic processes of maize seedlings under salt stress. It was found (A and B) that an increase in the leaf contents of soluble sugar and soluble protein of sorghum seedlings occurred under salt stress, and seed priming with Se(IV) increased the leaf contents of soluble sugar and soluble protein in salt-stressed sorghum seedlings. Which is consistent with the findings by Wang et al. (Citation2012) in rice reported that proteins involved in photosynthesis were upregulated under low Se concentration. In foxtail millet, the total soluble proteins and total soluble sugars increased in response to the supplementation of Se (Shah et al. Citation2020). Turakainen et al. (Citation2004) suggested that the increase in soluble sugar content of potato leaves after Se application may be related to the role of Se as a protective element in plant chloroplast enzymes and carbohydrate metabolism.
Proline is a low molecular weight antioxidant that plays an important role in an osmotic adjustment under salinity stress (Desoky et al. Citation2021). It can reduce the inhibitory effects of ions on anti-ROS activity, reduce ROS damage and promote plant tolerance (Hayat et al. Citation2012). Relative electrical conductivity is considered an indicator of the integrity of the cell membrane. It was found (C and A) that the proline content and relative electrical conductivity of sorghum seedling leaves increased under salt stress. Seed priming with Se(IV) increased the proline content and reduced the relative electrical conductivity in salt-stressed sorghum seedlings. This is consistent with previous findings by Elkelish et al. (Citation2019) in wheat, who reported that proline content increased under salt stress, and Se regulated proline accumulation by enhancing γ-GK enzyme activity and decreased its degradation by reducing PROX enzyme activity. Seed priming with Se(IV) can alleviate osmotic stress by increasing the content of osmoregulatory substances, which is an effective approach to alleviate salt stress-triggered membrane lipid peroxidation and hence retain the cell membrane integrity and stability. This results in the maintenance of plasma membrane properties and functionality.
Salt stress can induce a ROS burst and trigger cell membrane lipid peroxidation, which in turn destroys the structural and functional integrity of cell membranes, affecting normal metabolic activities in plants. Because MDA is a product of peroxidation, its content indicates the level of membrane lipid peroxidation in plant cells. It was found (D) that an increase occurred in the leaf MDA content of sorghum seedlings under salt stress, indicating stress-induced damage to the cell membrane system. The increase in leaf MDA content under salinity was countered upon seed priming with Se(IV), which is consistent with a previous report on wheat (Elkelish et al. Citation2019). These results indicate that Se(IV) application can benefit the growth of sorghum seedlings by reducing ROS accumulation and alleviating cell membrane lipid peroxidation. This may be due to the fact that Se counteracted the change in fatty acids saturation induced by NaCl stress and prevented the DNA demethylation process of plant seedlings (Maria et al. Citation2008).
Excess ROS accumulation resulting from salt damage harm plant cells if not cleared in time. Li et al. (Citation2022) also showed that under low salt and alkali stress, ROS accumulation in Acer truncatum was controlled by increasing SOD, POD and CAT activities. However, when the salt concentration exceeded the tolerance range of A truncatum, SOD, POD and CAT activities all decreased and cell membrane lipid peroxidation intensified. It was found () that the SOD, POD and CAT activities in the leaves of sorghum seedlings considerably increased under 120 mmol/L NaCl treatment. Seed priming with Se(IV) significantly enhanced SOD, POD and CAT activities in the leaves of sorghum seedlings under salt stress, which is consistent with findings by Shah et al. (Citation2020). In summary, our results show that priming seeds with Se(IV) increase antioxidant enzyme activities in salt stress seedlings of sorghum, which can facilitate ROS clearance in cells and reduce cell membrane lipid peroxidation. Indeed, Se regulates the expression of resistance-related genes at the transcriptional level, thus improving the activity of antioxidant enzymes (Zhang Citation2017).
Because chlorophyll is the most important pigment for plant photosynthesis, the changes in chlorophyll content directly affect photosynthesis intensity, which in turn influences crop yield. It was found (B) that a decrease occurred in the leaf total chlorophyll content of sorghum seedlings under salt stress, seed priming with Se(IV) not only promoted the leaf total chlorophyll content of sorghum seedlings under non-stress conditions, but also induced an increase in the leaf total chlorophyll content of sorghum seedlings under salt stress, which is consistent to findings by Desoky et al. (Citation2021) in wheat reported that salt stress caused a decrease in total chlorophyll content, stomatal conductance and photosynthetic rate and foliar spraying of Se solution mitigated the harmful effects of salt stress. Exogenous Se (IV) can enhance plant survival capabilities under salinity conditions, most likely by regulating the expression of key genes in plant leaves, increasing photosynthetic function and promoting the synthesis of photosynthetic products, resulting in increased dry matter accumulation (Desoky et al. Citation2021; Shah et al. Citation2022).
Roots are the most sensitive organ for crops to sense stress and initiate the response. Root activity indicates the ability of plants to absorb water and nutrients in the soil. Plants that show stronger root activity have a greater ability to absorb water and nutrients, with a more vigorous metabolism and stronger stress resistance. The results (C) show that the root activity of sorghum seedlings was markedly inhibited under salt stress, which is consistent with the results of other studies that found salt stress reduces plant root length and activity (Wang et al. Citation2022b). Seed priming with Se(IV) imparts benefits to photosynthesis and root activity in sorghum seedlings, but the effects on sorghum yield and quality need to be further studied.
Conclusion
Petri dish and pot experiments demonstrated that NaCl-induced salt stress affected seed germination and seedling growth of grain sorghum by inducing cell membrane lipid peroxidation. Seed priming with selenium (25 μmol/L Na2SeO3) may have enhanced plant’s capacity for tolerance by enhancing antioxidant enzyme activities, increasing soluble sugar, soluble protein, proline and chlorophyll contents and reducing membrane lipid peroxidation, as well as increasing root activity. Therefore, seed priming with Se(IV) is an effective strategy for facilitating sorghum germination and growth under salt stress. Se(IV) can be used for the induction of salt tolerance and promotion of biomass production in grain sorghum grown on saline soils. This study provides a reference for improving plant survival capacities under salt stress. Mengen Nie, [email protected], Center for Agricultural Gene Resources Research, Shanxi Agricultural University, Taiyuan, Shanxi, People's Republic of China.
Disclosure statement
No potential conflict of interest was reported by the author(s).
Additional information
Funding
Notes on contributors
Mengen Nie
Mengen Nie is an assistant research at Center for Agricultural Gene Resources Research, Shanxi Agricultural University. Her area of work focuses on sorghum germplasm innovation and new variety selection.
Na Ning
Na Ning is an associate professor at College of Resources Environment and Chemistry, Chuxiong Normal University. Her area of work focuses on high yield and efficient crop cultivation technology and theory.
Du Liang
Du Liang is an associate research at Sorghum Research Institute, Shanxi Agricultural University. His area of work focuses on the selection and breeding of new sorghum varieties.
Haiping Zhang
Haiping Zhang is a research at Center for Agricultural Gene Resources Research, Shanxi Agricultural University. Her area of work focuses on crop drought tolerance gene mining.
Shuangshuang Li
Shuangshuang Li is an undergraduate student at College of Resources Environment and Chemistry, Chuxiong Normal University. Her work focuses on crop adversity physiology.
Shuai Li
Shuai Li is an undergraduate student at College of Resources Environment and Chemistry, Chuxiong Normal University. His work focuses on crop adversity physiology.
Xinqi Fan
Xinqi Fan is an assistant research at Sorghum Research Institute, Shanxi Agricultural University. Her work focuses on sorghum genetics and germplasm innovation.
Yizhong Zhang
Yizhong Zhang is an associate research at Sorghum Research Institute, Shanxi Agricultural University. His area of work focuses on the selection and breeding of new sorghum varieties.
References
- Aebi H. 1984. Catalase in vitro. In: In Methods in enzymology. New York: Academic Press.
- Bao LG, Lu P, Shi MS, Xu Y, Liu MX. 2020. Screening and identification of Chinese sorghum landraces for salt tolerance at germination and seedling stages. Acta Agron Sin. 46:734–744.
- Breia R, Conde A, Badim H, Fortes AM, Gerós H, Granell A. 2021. Plant SWEETs: from sugar transport to plant-pathogen interaction and more unexpected physiological roles. Plant Physiol. 186:836–852.
- Desoky ESM, Merwad AMA, El-Maati A, Mansour MFA, Arnaout E, Awad SMAI, Ramadan MF, Ibrahim MF, A S. 2021. Physiological and biochemical mechanisms of exogenously applied selenium for alleviating destructive impacts induced by salinity stress in bread wheat. Agronomy. 11:926–926.
- Dev SR, Chandra KM. 2022. Green synthesized Se nanoparticle-mediated alleviation of salt stress in field mustard TS-36 variety. J Biotechnol. 359:95–107.
- Elkelish AA, Soliman MH, Alhaithloul HA, El-Esawi MA. 2019. Selenium protects wheat seedlings against salt stress-mediated oxidative damage by up-regulating antioxidants and osmolytes metabolism. Plant Physiol Biochem. 137:144–153.
- Fan SY. 2022. Study on physiological mechanisms for exogenous selenium-mediated drought and salt tolerance in tomato [master’s thesis]. Shanxi(CHN): Northwest A&F University.
- Hare PD, Cress WA, Staden JV. 1998. Dissecting the roles of osmolyte accumulation during stress. Plant Cell Environ. 21:535–553.
- Hayat S, Hayat Q, Alyemeni MN, Wani AS, Pichtel J, Ahmad A. 2012. Role of proline under changing environments. Plant Signal Behav. 7:1456–1466.
- Lei XH, Wan CX, Tao JC, Leng JJ, Wu YX, Wang JL, Wang PK, Yang QH, Feng BL, Gao JF. 2022. Effects of soaking seeds with MT and EBR on germination and seedling growth in buckwheat under salt stress. Acta Agron Sin. 48:1210–1221.
- Li HS. 2000. Principles and techniques of plant physiological and biochemical experiments. Beijing: Higher Education Press.
- Li HC, Li YF, Wang B, Sha N, Liu JQ, Du C, Jing Y, Ren DS. 2018. Response of different oat varieties to NaCl stress and its salt tolerance threshold. J North Agric. 46(03):13–17.
- Li Y, Cai YA, Ren AQ, Wei LX, Zhang YH, Zhang RX, Li QW. 2022. Effects of saline-alkali stress on growth and physiological characteristics of Acer truncatum seedlings. J Northeast For Univ. 50:5–14. + 21.
- Liang Y. 2020. Alleviating effects of exogenous selenium on nutrient accumulation and salt stress in durum wheat [master’s thesis]. Sichuan (CHN). Chengdu: University.
- Liu HB, Wei YQ, Zhou WS, Zou C. 2016. Effects of NaCl stress on physiological and biochemical characteristics of sweet sorghum and spring wheat at germination stage. Jiangsu Agric Sci. 44:106–111.
- Maria F, Rucka K, Helina H, Iwona S, Agnieszka J, Zbigniew M, Agnieszka G. 2008. The protective role of selenium in rape seedlings subjected to cadmium stress. J Plant Physiol. 165:833–844.
- Nemat Alla MM, Badran EG, Mohammed FA, Hassan NM, Abdelhamid MA. 2020. Overexpression of Na+-manipulating genes in wheat by selenium is associated with antioxidant enforcement for enhancement of salinity tolerance. Rendiconti Lincei. Scienze Fisiche e Naturali. 31:177–187.
- Perveen S, Iqbal N, Saeed M, Zafar S, Arshad Z. 2018. Role of foliar application of sulfur-containing compounds on maize (Zea mays L. var. Malka and hybrid DTC) under salt stress (Zea mays L. var. Malka and hybrid DTC) under salt stress. Braz J Bot. 41:805–815.
- Rasool A, Shah WH, Tahir I, Alharby HF, Hakeem KR, Rehman R. 2020. Exogenous application of selenium (Se) mitigates NaCl stress in proso and foxtail millets by improving their growth, physiology and biochemical parameters. Acta Physiol Plant. 42:191–205.
- Rosa M, Prado C, Podazza G, Interdonato R, González JA, Hilal M, Prado FE. 2009. Soluble sugars. Plant Signal Behav. 4:388–393.
- Shah AA, Yasin NA, Mudassir M, Ramzan M, Hussain I, Siddiqui MH, Ali HM, Shabbir Z, Ali A, Ahmed S, Kumar R. 2022. Iron oxide nanoparticles and selenium supplementation improve growth and photosynthesis by modulating antioxidant system and gene expression of chlorophyll synthase (CHLG) and protochlorophyllide oxidoreductase (POR) in arsenic-stressed Cucumis melo. Environ Pollut. 307:119413.
- Shah WH, Rasool A, Tahir I, Rehman RU. 2020. Exogenously applied selenium (Se) mitigates the impact of salt stress in Setaria italica L. and Panicum Miliaceum L. Nucleus. 63:299–301.
- Siddiqui SA, Blinov AV, Serov AV, Gvozdenko AA, Kravtsov AA, Nagdalian AA, Raffa VV, Maglakelidze DG, Blinova AA, Kobina AV, et al. 2021. Effect of selenium nanoparticles on germination of hordéum vulgáre barley seeds. Coatings. 11:862.
- Turakainen M, Hartikainen H, Seppänen MM. 2004. Effects of selenium treatments on potato (Solanum tuberosum L.) growth and concentrations of soluble sugars and starch. J Agric Food Chem. 52:5378–5382.
- Wang MQ, Gong SC, Fu LX, Hu GH, Li GL, Hu SX, Yang JF. 2022a. The involvement of antioxidant enzyme system, nitrogen metabolism and osmoregulatory substances in alleviating salt stress in inbred maize lines and hormone regulation mechanisms. Plants. 11:1547–1547.
- Wang JX, Wang YZ, Xing LX, Liu JX, Wang RM. 2022b. Effects of application of GA3 on root growth and osmotic adjustment of broomcorn millet seedlings in NaCl stressed. Crops. 98–104.
- Wang YD, Wang X, Wong YS. 2012. Proteomics analysis reveals multiple regulatory mechanisms in response to selenium in rice. J Proteom. 75:1849–1866.
- Wang ZB, He ZH, Xu X, Shi XX, Ji XY, Wang YC. 2021. Revealing the salt tolerance mechanism of Tamarix hispida by large-scale identification of genes conferring salt tolerance. Tree Physiol. 41:2153–2170.
- Zeng YL, Li L, Yang RR, Yi XY, Zhang BH. 2015. Contribution and distribution of inorganic ions and organic compounds to the osmotic adjustment in Halostachys caspica response to salt stress. Sci Rep. 5:13639.
- Zhang F, Sapkota S, Neupane A, Yu JL, Wang YQ, Zhu K, Lu F, Huang RD, Zou JQ. 2020. Effect of salt stress on growth and physiological parameters of sorghum genotypes at an early growth stage. Iindina J Exp Biol. 58:404–411.
- Zhang JW. 2017. Effects of exogenous selenium on the growth of tomato seedlings under Ca(NO3)2 stress [master’s thesis]. Xinjiang (CHN). Shihezi University.
- Zhang R, Wang Y, Shahid H, Liu YH, Shao XY, Yang S, Chen YL, Wei HH, Dai QG. 2021. Identification of salt-tolerant rice cultivars in the growth period under hydroponic conditions. J Plant Genet Resour. 22:1567–1581.
- Zhu GL, Song CY, Yu LL, Chen XB, Zhi WF, Liu JW, Jiao XR, Zhou GS. 2018. Alleviation effects of exogenous growth regulators on seed germination of sweet sorghum under salt stress and its physiological basis. Acta Agron Sin. 44:1713–1724.
- Zou Q. 2000. Experimental guidance in plant physiology. Beijing: China Agricultural Press.