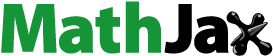
ABSTRACT
In arid Africa, it is unclear how the transition from grassland to cropland affects CO2 fluxes and whether these effects are climate-dependent. From November 2019 to March 2020 (dry season), we studied autotrophic (Ra) and heterotrophic (Rh) soil respiration. At highlands, grazing land (1.18 ± 0.13 µmol m−2 s−1) and teff croplands (1.36 ± 0.14 µmol m−2 s−1) had the lowest Rh, while acacia decurrens plantations (1.94 ± 0.19 µmol m−2 s−1) were the largest. When compared to teff (2.08 ± 0.20 µmol m−2 s−1) and khat (1.73 ± 0.24 µmol m−2 s−1), grazing land in the midland had a lower Rh (1.18 ± 0.13 µmol m−2 s−1). Ra on teff (1.51 ± 0.27 µmol m−2 s−1) was substantially (P < 0.05) greater than on grazing land (1.08 ± 0.32 µmol m−2 s−1) in the highlands. Grazing lands (1.54 ± 0.21 µmol m−2 s−1) have a considerably (P < 0.05) greater Ra than farmlands (teff, 0.20 ± 0.07 µmol m−2 s−1; groundnut, 0.09 ± 0.03 µmol m−2 s−1) in lowlands. Growing teff in the highlands and midlands will result in higher CO2 fluxes into the atmosphere. However, in-depth research is needed to implement effective land management that reduces CO2 emissions.
Introduction
Soil respiration (Rs) or CO2 flux is the mechanism by which soil releases carbon dioxide (CO2). Annual global CO2 fluxes from soil ranged from 68 to 87 Pg C yr−1 (Warner et al. Citation2019). Rs is the sum of the autotrophic (Ra) and heterotrophic (Rh) respiration of soil organisms and plant roots, respectively. The worldwide terrestrial carbon cycle includes Rh, which is influenced by environmental factors (Bukombe et al. Citation2021). Rh is also influenced by microbial biomass such as fungi and bacteria (Li et al. Citation2006). To determine CO2 emissions, it is necessary to quantify the contribution of Ra and Rh to Rs. The main uncertainty in projecting future climate-carbon feedback is the emission of CO2 (due to Ra and Rh) in soils. An increase in SOC loss and subsequent climatic feedback could cause the Rh/Rs ratio to increase (Bond-Lamberty et al. Citation2018). The global response of Rh and Ra to climate change is important in predicting the future behaviour of the terrestrial carbon cycle. In subtropical China, coniferous forests have much lower Rh levels than three other types of forests with a predominance of broadleaf species (Wei et al. Citation2015). Ra’s vulnerability to temperature increased with land use, unlike that of Rh.
The potential for African soils to act as substantial carbon reserves is quite high (Epule Citation2015). Farmland modifications and forest destruction are getting worse in the Congo basin and other tropical areas, and this worsening is having an impact on carbon emissions (Verchot et al. Citation2020). About 60% of the African continent is composed of savannas, which are important contributors to global carbon emissions via land use and fire (Fan et al. Citation2015). Due to their vast expanse, savannas cause significant changes in global carbon levels that are difficult to understand and predict, explaining global warming. Heterotrophic microorganisms break down SOC, resulting in the release of CO2 into the air. The overuse of South Africa’s Karoo rangeland is anticipated to lead to changes in ecosystem function, and measuring Rs may help to identify these changes (Mills et al. Citation2011). Africa’s growing participation in the carbon cycle could, have a major implications for future climate change. To understand how climate change affects the carbon cycling and accumulation, it is necessary to decompose Rs into its components. The Rs composition of soils has been measured using a variety of methods, with varying results from one study to another range of methodologies, and there is variation in the results among studies. However, root excavation experiments are commonly used to study Ra and Rh (Hu et al. Citation2018).
Soil respiration studies conducted in Ethiopia include those by Fekadu et al. (Citation2023), who reported on the dynamics of soil respiration in land management practices. Michelsen et al. (Citation2004) investigated soil CO2 emissions during the wet season, and Teklay et al. (Citation2006) studied the kinetics of soil respiration as a function of microbial nutrient status. There has never been a study of Ra and Rh anomalies in different agroecological regimes of different land uses in Ethiopia. Our objectives here were to (1) estimate the magnitude of Ra and Rh in dry months; (2) examine the effects of different land uses and agroecological systems on Ra and Rh; and (3) evaluate the factors contributing to variations in the components of Rs. We hypothesised that Ra and Rh differ significantly across land uses in different agroecologies.
Materials and methods
Study sites
Three agroecological systems were used for the research (). Districts in which the study’s watersheds were located varied. The Dibatie site (10o45′30″−10o47′0″N, 36o16′0″−36o18′0″E), the Aba Gerima (11o38′0″−11o40′30″N, 37o29′30″−37o31′0″E), and the Guder watershed (10o59′30″−11o1′0″N, 36o54′0″−36o56′0″E) were used to represent the lowlands, midlands, and highlands, respectively (Berihun et al. Citation2019). These locations exhibit various land uses, annual rainfall patterns, and elevations in the major agroecological systems in the basin (Sultan et al. Citation2018). The predominant crops, major land uses, soil types, and climatic data for each watershed are listed in . We collected climate data from the Bahir Dar for the Aba Gerima, the Dangila and Enjibara for the Guder watershed, and the Chagni and Dibatie meteorological stations for the Dibatie watershed.
Table 1. Research site features.
The growing season in the study area is somewhat different. Sowing everywhere begins in July. Harvest is expected in the midlands and lowlands in November. In the highlands, the harvest season continues into January. The dry season lasts from November to April. When looking at the onset of the dry season (November) in the midland and lowlands, crops are in their maturation stage or ready for harvest. However, this period was the tillering season, the beginning of the data collection, and January is the end of the harvest season in the highlands.
The major land uses in the study sites were teff cropland, plantations, and grazing land in the midlands and highlands. But in the lowlands, teff cropland, groundnut, and grazing lands were the dominant land uses. In the highlands, croplands accounted for roughly 41% of all land usage, followed by acacia plantations at 30% and grazing land at 9.5% (Sultan et al. Citation2018). The main land use in the midland was arable land, accounting for 51.64%, followed by plantations and grazing land at 8.9 and 3.43%, respectively (Mulualem et al. Citation2021). Lowland arable land accounted for 54.0% of the total land in 2017, with grazing land the second largest in 1982 (36.4%). However, grazing area decreased by 38.3% between 1982 and 2017, mainly due to arable land (Berihun et al. Citation2019).
Setup of experimental plots
In order to explore Rh and Ra in the three contrasting watersheds, we established experimental plots with various land uses in 2019. Fifty-four fixed test plots were set up (3 replicates, 3 land uses, 2 variants [with or without roots], and 3 watersheds). We inserted a polyvinyl chloride (PVC) collar in each experimental plot at the commencement of the Rs and Rh measurements, 0.05 meters below the soil’s surface. The PVC collar used to define the area for measurement of Rh and Rs was 0.19 meters in diameter and 0.11 meters long (Fekadu et al. Citation2023). Experiments were conducted where the slope was nearly uniform in each watershed. The mean elevation in the highlands was 2585, 2586, and 2587 m a.s.l. for grazing land, A. decurrens, and teff cropland, respectively. In the midlands, the average elevations for teff cropland, grazing land, and khat plantations were 1992, 1991, and 1989m a.s.l., respectively. There were no elevation data available for the lowlands study site because data collection had been interrupted due to security problems. The land uses were located at adjacent sites in each watershed. The experimental plots were designed to measure Rs and Rh. One meter of soil was removed from the ground. The soil was then filled in after any roots had been manually removed. To ensure the soil’s stability and the accuracy of the Rh measurements, before placing the PVC collar, we waited 6 months after we had replaced the soil in each hole (). To avoid root respiration, we cut plants or any weed without disturbing soils before measuring data at Rh plots. On the same date, we measured soil respiration at the Rs and Rh plots. We subtract Rh value from Rs to get Ra. All plots were 1 meter square. To avoid further variations, the distance between paired plots with and without roots was about 1 meter for each land use.
Data collection
The Li 8100A, a device for measuring Rs rates, was used to collect data between November 2019 and March 2020. Each month, Rh and Rs are measured between 9:00 and 12:00 am (Fekadu et al. Citation2023). For measurements using dynamic closed-chamber systems, the chamber had to be placed on the PVC collar on the soil surface for 60–120 s (Rochette et al. Citation1997). We measured Rh and Rs for 90 s. Since the machine records every second, an average of Rs or Rh was used for each collar. Measurements were subjected to a 90% coefficient of determination of the lower limit prior to analysis (Savage et al. Citation2008). Along with data on soil respiration (Rs and Rh), the soil’s moisture and temperature were simultaneously monitored. Moisture content was monitored monthly by using a hand auger to collect approximately 200 g of soil from each plot at a depth of 0.05 m, as used for the Rs measurement. Soil samples were kept in an oven at 105°C for 24 h until a stable weight was reached. To calculate the soil moisture (Sm), the difference in mass between the wet and dry soil samples was divided by the mass of the dry sample and multiplied by 100. A soil temperature (ST) omega probe, module number 6000-09TC (Li-Cor Inc.), was used to estimate ST at a depth of 0.05 m.
Sampling and statistical analysis
Soil samples were taken from the experimental site at a depth of 20 cm (Rs and Rh plots within 1 m2) for further analysis. After the soil samples were dried in the air at room temperature, they were crushed in a mortar and pestle and sieved through a sieve having a mesh size of 2 mm. Soil pH and texture were studied using soil samples sieved with a 2 mm mesh sieve. For total nitrogen (TN) and soil organic carbon (SOC) analyses, the soil was ground and soil samples were sieved through a 0.5 mm sieve. Soil pH was measured in a supernatant suspension of a mixture of soil and distilled water in a 1:2.5 ratio using a pH meter (Peech Citation1965). Soil organic carbon (SOC) was measured by the dichromate oxidation method of Walkley and Black (Citation1934). Total nitrogen (TN) was titirimetrically determined using the Kjeldahal method described by Nelson and Sommers (Citation1980). Soil texture was analyzed using a hydrometer (Bouyoucos Citation1962). The bulk density of the soil was determined by the core method.
A one-way analysis of variance (ANOVA) was used to examine statistical differences in soil properties between major land uses. Least Significant Difference (LSD) tests were used to detect significant differences (P < 0.05) between soil properties for major land uses. The data were checked for normality for Rh and Ra. The normality checks showed that all of the Rh and Ra data were non-normal. In order to analyze the data, Friedman’s non-parametric test was applied. Friedman’s analysis was followed by a Wilcoxon post-hoc analysis (P < 0.05) to separate means. Pearson correlations were also used to study the relationship between Rs components, environmental factors, and soil properties.
The following function was used to estimate Rh or Ra in ST (Huang et al. Citation2018):
(1)
(1) where Ra or Rh is the CO2 flux in µmol m−2 s−1; ST – soil temperature (oC); a and b are the model coefficients.
The value of Q10 (temperature sensitivity) was calculated by substituting parameter b into Equation 2 (Meyer et al. Citation2018a)
(2)
(2) Where b is the coefficient obtained from Equation 1
From Sm data, either Rh or Ra might be predicted using the second-order equation below (Peng et al. Citation2015):
(3)
(3) Where, Sm is soil moisture in %; a, b and c are the model coefficients.
A regression model was applied to predict Ra/Rh in ST and Sm (Cui et al. Citation2020):
(4)
(4) In the equations above, all variables are as specified
Results
Characteristics of soil
Soil physic-chemical analysis was performed for bare soil (without roots to evaluate Rh) and for soil covered in the relevant grassland, crop, or plantation to evaluate Rs. No significant differences (P > 0.05) were found between soil parameters by land-use type in the highlands at Rh plots. In the midlands TN, clay content varied significantly (P < 0.001) between land uses, and soil pH varied significantly (P < 0.001). In lowlands, sand and silt varied significantly (P < 0.05) across land use. At the same time, pH, BD, and clay content were statistically different at P < 0.01 (see ).
Table 2. Topsoil (0–20 cm) sample characteristics (mean ± SE) in the bare soil (Rh plots) (n = 3).
One-way ANOVA results for Rs plots showed that in highland soil, pH was statistically (P < 0.05) lower in teff cropland or Acacia decurrens plantations than in grazing land (Table S1). In the midland and lowlands Rs plots, pH varied significantly (P < 0.05) across land use, where SOC, TN, sand, and clay were statistically different at P < 0.01 (Table S1).
Monthly variations in Rh and Ra in different agroecological systems and with different land uses
On grazing land, the lowest Ra value was recorded in the midlands in January, and the highest was recorded in the highlands in November. In December, the lowlands on teff had the smallest mean of Ra and the highest in the highlands in November ((a)–). The lowest Rh value on grazing land was recorded in March in the lowlands, followed by the midlands in March and the highlands in November. The highest Rh value on grazing land was recorded in the lowlands in January, followed by the midlands in January and the highlands in December. The lowest and highest Rh values on teff cropland were found in the lowlands in March and the midlands in January, respectively ((b)–).
Figure 3. Monthly soil respiration rates at Guder for various land uses: Ra, autotrophic soil respiration (a); Rh, heterotrophic soil respiration (b); Rs, soil respiration (c); and Rh/Rs (d). Note: TC, teff cropland; GL, grazing land; AP, acacia decurrens plantation.

Figure 4. Monthly soil respiration rates at Aba Gerima for various land uses: Ra, autotrophic soil respiration (a); Rh, heterotrophic soil respiration (b); Rs, soil respiration (c); and Rh/Rs (d). Note: TC, teff cropland; GL, grazing land; KP, khat plantation.

Figure 5. Monthly soil respiration rates at Dibatie for various land uses: Ra, autotrophic soil respiration (a); Rh, heterotrophic soil respiration (b); Rs, soil respiration (c); and Rh/Rs (d). Note: TC, teff cropland; GC, groundnut cropland; GL, grazing land.

In the highland catchment, the lowest average Ra CO2 fluxes at grazing land (0.32 ± 0.11µmol m−2 s−1) and teff (0.78 ± 0.16 µmol m−2 s−1) were measured in March. Conversely, the lowest average flux of Ra (0.36 ± 0.11µmol m−2 s−1) in the A. decurrens was observed in February. In November, teff (2.81 ± 0.41µmol m−2 s−1) recorded the highest average Ra at highland, preceded by grazing land (2.42 ± 1.32 µ mol m−2 s−1) and A. decurrens (1.82 ± 0.75 µmol m−2 s−1) (). In midland, the mean Ra value varied from 0.12 ± 0.07 (January) to 2.29 ± 0.54 (December) for grazing land; from 0.85 ± 0.22 (March) to 2.17 ± 0.15 (November) for teff; and from 0.1 ± 0.05 (March) to1.62 ± 0.22 µmol m−2 s−1 (December) for khat (). The lowest average Ra CO2 fluxes in lowland were measured in March for land use grazing and (0.92 ± 0.29) and groundnut (0.03 ± 0.01 µmol m−2 s−1). On teff, the lowest Ra was recorded in December. The greatest Ra values were discovered on grazing land in February, teff in March, and groundnut in December ().
The lowest mean Rh CO2 fluxes at the highland site were found at grazing land in November, teff in March, and A. decurrens in March. The highest mean grazing land and A. decurrens values were observed in December, whereas CO2 flux on teff cropland was highest in November (). At the midland site, the lowest Rh CO2 flux values were in decreasing order: khat in March, teff in December, and grazingland in March. Similarly, the peak Rh CO2 flux values with the same decreasing trend were recorded at khat in November, teff in January, and grazing land in January (). Rh CO2 flux values in lowlands were generally lower than in the other agroecologies. In grazing land, Rh (µmol m−2 s−1) ranged from 0.33 ± 0.11 in March to 3.18 ± 0.64 in January. The lowest and highest values of Rh CO2 flux at teff cropland were in March and December, respectively. For groundnut, the months of January and March had the most and the least Rh CO2 flux levels, respectively ().
Examination of the mean Rh as a percentage of Rs at highland showed that A. decurrens (71%) had the highest percentage, followed by grazing land (58%) and teff cropland (49%) (). At midland, the lowest mean Rh/Rs percentage (57%) was observed on grazing land and the highest (71% on khat) (). At lowland, groundnut (83%) and teff (77%) had higher mean Rh/Rs percentages than grazing land (46%) ().
Agroecological systems and land use influences in Ra and Rh
The mean Rh value of grazing land was higher in the lowlands than in the other two areas, whereas it was the same in the highlands and midlands (). For teff cropland, the highest mean rank Rh value was recorded in the midlands and then the highlands, followed by the lowlands. From the highlands through the midlands to the lowlands, the overall mean Ra value exhibited a rising tendency for grazing land and a steady decline for teff. The mean Ra and Rh values for plantations were greater in the highlands than in the midlands (). The maximum Rh value in the highlands was found in A. decurrens, which was accompanied by teff and grazing land. In the midlands, Rh was higher on teff and khat than on grazing land. In lowland areas, no significant difference in Rh value was observed; however, grazing land had the highest Rh values, preceded by teff and groundnut. Furthermore, in the highlands, the Ra value on teff was markedly (P < 0.05) greater than on grazing land. In the midlands, the Ra measurements at different land uses did not differ significantly from each other. However, the highest Ra values were found for teff (1.40 ± 0.26), GL (1.16 ± 0.28), and khat (0.78 ± 0.17). In the lowlands, grazing land had a statistically (P < 0.05) higher Ra than teff and groundnut ().
Table 3. ANOVA results for Ra and Rh (µmol m−2 s−1) at various land uses in different agroecological systems (n = 3 for each land use for each month).
Factors influencing Ra and Rh
In the highland study area, there were no statistical relationships found between soil variables and Rh, although there were trends toward positive correlations of Rh with SOC, clay content, and soil pH (Table S2). At midland, Rh was significantly correlated (r = 0.75*) with clay content. SOC and TN also showed non-significant positive relations with Rh (r = 0.04 and 0.68, respectively). A significant correlation was observed between Rh and the pH of the soil (r = 0.77*), silt (r = 0.78*), and clay (r = −0.83*) in the lowland study area. SOC and TN also demonstrated a non-significant trend toward positive association with Rh (r = 0.47 and 0.60, respectively) (Table S2).
Rh and Sm strongly associated in highland study site (r = 0.42**); however, ST did not significantly connect with Rh (r = 0.05) (Table S2). In the midland study area, neither Sm nor ST was significantly correlated with Rh. In the lowland study area, Sm was not substantially connected with Rh, while ST was (r = −0.33*) (Table S4). Rh strongly (P < 0.05) associated exponentially with ST across all research sites. Sm was significantly associated with Rh in quadratic function at highland but not at midland or lowland (). Additionally, only at the highland location did Sm and ST show a strong connection with Rh.
Table 4. Interaction between environmental variables (ST and Sm) and Rh.
At the midland and highland sites, no conclusive relationships between soil variables and Ra were observed. Meanwhile, Ra was significantly (P < 0.05) related to pH, sand, silt, and clay content at the lowland research site (Table S3). In the highland study site, Ra was substantially (P < 0.01) correlated with Sm and ST (Table S4). While Sm (r = 0.35**) was substantially connected with Ra in midland, ST (r = 0.18) had a non-significant tendency toward a positive connection with Ra. In lowland, no significant (P > 0.05) correlation was found between Ra and Sm or ST (Table S4).
There was a significant (P < 0.05) exponential relationship between ST and Ra at all study sites. In the quadratic function, Ra was strongly (P < 0.05) correlated to Sm in highland but not in midland or lowland. The interaction between Sm and ST was also strongly (P < 0.05) associated with Ra in midland and highland ().
Table 5. Multiple regression models between Ra and environmental variables (ST and Sm).
Discussion
Effects of land-use type on soil properties in various agroecologies
The SOC in the midlands increased when other land uses replaced grazing land, but the increase was not significant. In the highlands, land-use change increased the SOC on teff but decreased it on A. decurrens. In the lowlands, a land-use shift from grazing land to other land uses decreased the SOC. It is possible that these variations in SOC are related to variations in land-management practices. In the midlands on grazing land, we noticed overgrazing and more soil degradation than with other land uses. In the case of the highland watershed, we noticed good land management practices on grazing land that were being used in a controlled manner. However, on the lowlands, the grasses are somewhat thorny, and it is possible that this made the pastures resistant to overgrazing. Rh values increased with a change from GL to other land uses in the highlands and midlands. In other land uses, there are different inputs that contribute to organic matter in the soil. Research done by Hao et al. (Citation2010), which revealed that a decline in SOC was the primary cause for low soil respiration, confirms this finding. Conversely, on the lowlands, the Rh values decreased with a change from grazing land to other land uses (see ).
Monthly variations in Ra and Rh in different agroecological systems and under different land uses
Monthly variations in Rh values were observed according to land use in each study area. From November through March, all sites showed a steady decline for Rh (see ). At the three study sites, the lowest Rh values were measured in March. The main reason for the variation in Rh values could be the different Sm contents in different months under different land uses. In highland, a strong association between Rh values and Sm levels was observed (Table S2). One study reported that CO2 emissions were highest in the months with the highest Sm (Valente et al. Citation2021). Additionally, moist soil conditions improve soil microbial physiological processes and root respiration, which increases Rs (Takahashi et al. Citation2011). One of the most significant ecosystem factors influencing production and carbon cycling in terrestrial ecosystems is Sm (Moyano et al. Citation2012).
Ra varied across months and under various land uses. There is a statistically significant association between Sm and Ra in midland and highland. Additionally; ST at highland had a significant relationship with Ra (Table S2). The study by Herbst et al. (Citation2016) found that ST did not account for a large portion of the fluctuation in Ra.
Impact of land use across different agroecological systems on Ra and Rh
In the highlands, we found a significantly (χ2 = 8.13, df = 2, P = 0.017) higher Rh value with A. decurrens than with the teff and grazing land uses. This implies that land-use conversion from grazing land or teff to A. decurrens contributes to an increase in Rh in the highland study areas. A possible reason for this could be Sm level (Hu et al. Citation2018). The average Sm for the A. decurrens land use was 34.44%, which was higher than the values for the teff (28.16%) and grazing land (29.46%), according to our measurements. At the highland research site, Sm was also substantially connected with Rh (r = 0.42*) (Table S4). However, this site’s soil characteristics and Rh did not significantly correlate (Table S2). In the midlands, the teff and khat land uses had significantly (χ2 = 6.53, df = 2, P = 0.038) higher Rh values than that of the grazing land (see ). At the midland watershed, land-use change from grazing to khat or teff cropland contributed to a higher Rh. The most probable reason could be changes in the soil properties. On teff and khat, the clay content was found to be considerably (P < 0.01) higher than on grazing land. In addition, the soil texture varied. The soil texture class for teff and khat was clay loam, whereas that for grazing was sandy loam (see ).
At midland, there was a substantial association between clay content and Rh (r = 0.75*) (Table S2). The amount of clay in the soil affects the amount of moisture. The addition of clay to sandy soils improves the water retention capacity by two to six times (Tahir and Marschner Citation2016). The primary element influencing soil CO2 emissions is the fluctuation in Sm (Valente et al. Citation2021). Rh is also affected by the presence of substrate and the soil environment. In environments with high substrate availability, the potential for carbon sequestration is lower and carbon release is greater (Aguilos et al. Citation2013). Soil Rh determines whether an ecosystem serves as a carbon sink or source. Sm influences Rs (Meyer et al. Citation2018b), and soil properties affect the response of Rh to moisture (Shahbaz et al. Citation2022).
Ra was substantially (P < 0.05) greater on teff than on grazing land at the highland site (). According to the ANOVA findings at highland, the soil pH was substantially (P < 0.05) high on teff than on grazing land (Table S1). The pH of the soil affects its biochemical processes and fundamentally affects soil behaviour (Neina Citation2019). In our study, Sm (r = 0.56**) and ST (r = 0.39**) were significantly associated with Ra at the highland site (see Table S4), and at lowland, we observed significantly (P < 0.05) higher Ra values on grazing land than on groundnut cropland. The reasons for this could be related to soil characteristics. The ANOVA results depicted that, at lowland, TN and SOC were considerably (P < 0.05) greater on grazing than on groundnut cropland. Moreover, pH of soil was substantially (P < 0.01) greater on grazingland compared to other land uses (Table S1). Autotrophic soil processes are closely coupled to carbon production by photosynthesis (Schindlbacher et al. Citation2009). A statistical association was found between Ra and soil pH, sand content, and silt content at Dibatie (see Table S3).
Factors contributing to Ra and Rh
Both Rh and Ra increased exponentially (P < 0.05) with ST across all study sites ( and ). Moreover, we found a significant linear relationship between Rh and ST (R2 = 0.11) at lowlands (), and a significant linear relationship (R2 = 0.15) between Ra and ST (). Rh levels increase with soil temperature. Soil microbial respiration is also temperature dependent. Higher temperatures can accelerate Rh, enhancing the activation of enzymes that degrade organic matter in the soil (Blagodatskaya et al. Citation2016). The main causes of this destruction are fungi and bacteria (Balogh et al. Citation2015). The components of soil respiration (Rh and Ra) are less dependent on the temperature in the midlands and lowlands than in the highlands. The reason is that the Q10 value is relatively high in the highlands, followed by the midland and lowlands in that order ().
Figure 6. Relationship between Rh, heterotrophic soil respiration (µmol m−2 s−1) with soil temperature (Oc) and soil moisture (%) at the three study sites.

Figure 7. Relationship between Ra, autotrophic soil respiration (µmol m−2 s−1) with soil temperature (Oc) and soil moisture (%) at the three study sites.

Sm made significantly contributions to Rh and Ra in both linear and quadratic functions at the highland study site (see (d) and , Table S4). One explanation for this is that the highland research site has a long rainy season, and the soils there may thus contain enough moisture for soil organisms. Rs increases with Sm, indicating that microbial activity is most by Sm (Thomas et al. Citation2011). It is generally accepted that Sm and ST affect Rh and Ra (Wei et al. Citation2015). Ra is more sensitive to ST than Rh, but Rh is more sensitive to Sm (Chen et al. Citation2017).
Multiple regression in the analysis showed that the combination of Sm and ST significantly affected Ra and Rh in the highlands ( and ). Our finding is supported by the study (Cai et al. Citation2021). No significant correlation was observed in the lowlands. Our study is supported by Hinko-Najera et al., (Citation2015), who reported that the effects of Sm and ST on the Rs component were not noticeable in lowlands.
In conclusion, Rs components varied on monthly basis across agroecologies and land use. The Ra values of teff cropland at highland and midland were higher than those of other land uses, whereas at lowland they were lower than those on grazing land. Similarly, the Rh value of teff cropland at midland was higher than other land uses; in highland Rh value was higher in acacia plantation than other land uses. These results suggest that growing teff in the relatively humid regions (highland and midland) will result in higher CO2 fluxes into the atmosphere. Sm was significantly associated (P < 0.01) with Ra and Rh at highland, but only Sm was statistically (P < 0.05) related to Ra at midland. However, in the lowlands, Sm was not statistically associated with components of Rs. ST and Ra only strongly (P < 0.01) associated in the highlands. Thus, the components of Rs at highlands continue to depend more on ST and Sm than at lowlands. An exponential function for ST (R2 = 0.24*), a second-order polynomial function for Sm (R2 = 0.34*), and multiple regression for both ST and Sm (R2 = 0.39*) explain the greater variation in Rh at highlands than any other agroecology. In general, different factors contributed to the variations in the components of Rs at the study sites. Consequently, more studies are needed to determine the effects of different land uses on Rh and Ra in order to predict future Rs components and suggest appropriate land management options.
Acknowledgements
We acknowledged the Japan Science and Technology/Japan International Cooperation Agency for funding this research through the Japan Science and Technology Research Partnership for Sustainable Development (SATREPS, grant number JPMJSA1601). We would also like to express our gratitude to the SATREPS project team at Bahir Dar University, Ethiopia, for their excellent collaboration in the laboratory and fieldwork.
Disclosure statement
No potential conflict of interest was reported by the author(s).
Data availability statement
The data analyzed during the current study are available from the corresponding author on rational request.
Additional information
Funding
Notes on contributors
Genetu Fekadu
Genetu Fekadu (M.Sc.) is a PhD candidate at Bahir Dar University in the soil science program and a lecturer at the University of Gondar, Ethiopia.
Enyew Adgo
Enyew Adgo (PhD) is a Professor and researcher at Bahir Dar University, Ethiopia.
Derege Tsegaye Meshesha
Derege Tsegaye Meshesha (PhD) is an Associate Professor and researcher at Bahir Dar University, Ethiopia.
Atsushi Tsunekawa
Atsushi Tsunekawa (PhD) is a Professor and researcher at Tottori University, Japan.
Nigussie Haregeweyn
Nigussie Haregeweyn (PhD) is a Professor and a researcher at Tottori University, Japan.
Fei Peng
Fei Peng (PhD) is an Associate Professor and a researcher at the Chinese Academy of Sciences, China.
Mitsuru Tsubo
Mitsuru Tsubo (Professor) is a Professor and researcher at Tottori University, Japan.
Asaminew Tassew
Asaminew Tassew (PhD) is an Associate Professor and researcher at Bahir Dar University, Ethiopia.
Gizachew Ayalew Tiruneh
Gizachew Ayalew Tiruneh (PhD) is an Assistant Professor and lecturer at Debre Tabor University, Ethiopia.
Nigus Tadesse
Nigus Tadesse (M.Sc.) is a PhD student at Bahir Dar University, College of Agriculture and Environmental Sciences, Ethiopia.
Tsugiyuki Masunaga
Tsugiyuki Masunaga (Professor) is a Professor and researcher at Shimanie University, Japan.
References
- Aguilos M, Takagi K, Liang N, Watanabe Y, Teramoto M, Goto S, Takahashi Y, Mukai H, Sasa K. 2022. Sustained large stimulation of soil heterotrophic respiration rate andits temperature sensitivity by soil warming in a cool-temperate forestedpeatland. Tellus Ser B Chem Phys Meteorol. 65:1–14.
- Arevalo CB, Bhatti JS, Chang SX, Jassal RS, Sidders D. 2010. Soil respiration in four different land use systems in north central Alberta, Canada. J Geophys Res. 115:1–12.
- Balogh J, Papp M, Pintér K, Fóti S, Posta K, Eugster W, Nagy Z. 2015. Autotrophic component of soil respiration is repressed by drought more than the heterotrophic one in a dry grassland. Biogeosciences. 12:16885–16911.
- Berihun ML, Tsunekawa A, Haregeweyn N, Meshesha DT, Adgo E, Tsubo M, Masunaga T, Fenta AA, Sultan D, Yibeltal M. 2019. Exploring land use/land cover changes, drivers and their implications in contrasting agro-ecological environments of Ethiopia. Land Use Policy. 87:104052.
- Blagodatskaya E, Blagodatsky S, Khomyakov N, Myachina O, Kuzyakov Y. 2016. Temperature sensitivity and enzymatic mechanisms of soil organic matter decomposition along an altitudinal gradient on mount Kilimanjaro. Sci Rep. 6:1–11.
- Bond-Lamberty B, Bailey VL, Chen M, Gough CM, Vargas R. 2018. Globally rising soil heterotrophic respiration over recent decades. Nature. 560:80–83.
- Bouyoucos GJ. 1962. Hydrometer method improved for making particle size analyses of soils. Agron J. 54:464–465.
- Bukombe B, Fiener P, Hoyt AM, Kidinda LK, Doetterl S. 2021. Heterotrophic soil respiration and carbon cycling in geochemically distinct African tropical forest soils. Soil. 7:639–659.
- Cai C, Yang Z, Liu L, Lai Y, Lei J, Fan S, Tang X. 2021. Consistent effects of canopy vs. Understory nitrogen addition on soil respiration and net ecosystem production in moso bamboo forests. Forests. 12:1–15.
- Chen Z, Xu Y, Fan J, Yu H, Ding W. 2017. Soil autotrophic and heterotrophic respiration in response to different N fertilization and environmental conditions from a cropland in Northeast China. Soil Biol Biochem. 110:103–115.
- Cui Y, Feng J, Liao L, Yu R, Zhang X, Liu Y, Yang L, Zhao J, Tan Z. 2020. Controls of temporal variations on soil respiration in a tropical lowland rainforest in hainan island, China. Trop Conserv Sci. 13:1–14.
- Epule TE. 2015. A New compendium of soil respiration data for Africa. Challenges. 6:88–97.
- Fan Z, Neff JC, Hanan NP. 2015. Modeling pulsed soil respiration in an African savanna ecosystem. Agric For Meteorol. 200:282–292.
- Fekadu G, Adgo E, Meshesha DT, Tsunekawa A, Haregeweyn N, Peng F, Tsubo M, Masunaga T, Tassew A, Mulualem T, Demissie S. 2023. Seasonal and diurnal soil respiration dynamics under different land management practices in the sub-tropical highland agroecology of Ethiopia. Environ Monit Assess. 195:1–17.
- Getahun M. 2016. Soil characterization, classification and mapping of three twin watersheds in the Upper Blue Nile basin (Aba Gerima, Guder and Dibatie). Bahir Dar, Ethiopia.
- Hao Q, Jiang C, Zhang J. 2010. Effect of marsh reclamation on heterotrophic soil respiration in Sanjiang Plain, Northeast China, in: World Congress of Soil Science. pp. 1993–1996.
- Herbst M, Tappe W, Kummer S, Vereecken H. 2016. The impact of sieving on heterotrophic respiration response to water content in loamy and sandy topsoils. Geoderma. 272:73–82.
- Hinko-Najera N, Fest B, Livesley SJ, Arndt SK. 2015. Reduced throughfall decreases autotrophic respiration, but not heterotrophic respiration in a dry temperate broadleaved evergreen forest. Agric For Meteorol. 200:66–77.
- Hu S, Li Y, Chang SX, Li Y, Yang W, Fu W, Liu J, Jiang P, Lin Z. 2018. Soil autotrophic and heterotrophic respiration respond differently to land-use change and variations in environmental factors. Agric For Meteorol. 250–251:290–298.
- Huang S, Ye G, Lin J, Chen K, Xu X, Ruan H, Tan F, Chen HYH. 2018. Autotrophic and heterotrophic soil respiration responds asymmetrically to drought in a subtropical forest in the Southeast China. Soil Biol Biochem. 123:242–249.
- Li Y, Xu M, Zou X. 2006. Heterotrophic soil respiration in relation to environmental factors and microbial biomass in two wet tropical forests. Plant Soil. 281:193–201.
- Meyer N, Meyer H, Welp G, Amelung W. 2018a. Soil respiration and its temperature sensitivity (Q10): rapid acquisition using mid-infrared spectroscopy. Geoderma. 323:31–40.
- Meyer N, Welp G, Amelung W. 2018b. The temperature sensitivity (Q10) of soil respiration: controlling factors and spatial prediction at regional scale based on environmental soil classes. Global Biogeochem Cycles. 32:306–323.
- Michelsen A, Andersson M, Jensen M, Kjøller A, Gashew M. 2004. Carbon stocks, soil respiration and microbial biomass in fire-prone tropical grassland, woodland and forest ecosystems. Soil Biol Biochem. 36:1707–1717.
- Mihretie FA, Tsunekawa A, Haregeweyn N, Adgo E, Tsubo M, Masunaga T, Meshesha DT, Ebabu K, Nigussie Z, Sato S, Berihun ML, Hashimoto Y, Kawbota A, Bayable M. 2022. Exploring teff yield variability related with farm management and soil property in contrasting agro-ecologies in Ethiopia. Agric Syst 196: 103338.
- Mills A, Fey M, Kgope B, Donaldson J, Bosenberg J. 2011. Detecting the onset of rangeland degradation using soil respiration and boundary lines: preliminary findings from the nama karoo. African J Range Forage Sci. 28:87–92.
- Moyano FE, Vasilyeva N, Bouckaert L, Cook F, Craine J, Yuste JC, Don A, Epron D, Formanek P, Franzluebbers A, et al. 2012. The moisture response of soil heterotrophic respiration: interaction with soil properties. Biogeosciences. 9:1173–1182.
- Mulualem T, Adgo E, Meshesha DT, Tsunekawa A, Haregeweyn N, Tsubo M, Ebabu K, Kebede B, Berihun ML, Walie M, et al. 2021. Exploring the variability of soil nutrient outflows as influenced by land use and management practices in contrasting agro-ecological environments. Sci Total Environ. 786:147450.
- Neina D. 2019. The role of soil pH in plant nutrition and soil remediation. Appl Environ Soil Sci. 1–9.
- Nelson DW, Sommers LE. 1980. Total nitrogen analysis of soil and plant tissues. J Aoac Int. 63:770–778.
- Peech M. 1965. Hydrogen-Ion activity. Amer Soc. Agron. 60:914–926.
- Peng F, You QG, Xu MH, Zhou XH, Wang T, Guo J, Xue X. 2015. Effects of experimental warming on soil respiration and its components in an alpine meadow in the permafrost region of the qinghai-Tibet plateau. Eur J Soil Sci. 66:145–154.
- Rochette P, Ellert B, Gregorich EG, Desjardins RL, Pattey E, Lessard R, Johnson BG. 1997. Description of a dynamic closed chamber for measuring soil respiration and its comparison with other techniques. Can J SOIL Sci. 77:195–203.
- Savage K, Davidson EA, Richardson AD. 2008. A conceptual and practical approach to data quality and analysis procedures for high-frequency soil respiration measurements. Funct Ecol. 22:1000–1007.
- Schindlbacher A, Sechmeister-Boltenstern S, Jandl R. 2009. Carbon losses due to soil warming: Do autotrophic and heterotrophic soil respiration respond equally? Glob Chang Biol. 15:901–913.
- Shahbaz M, Bengtson P, Mertes JR, Kulessa B, Kljun N. 2022. Spatial heterogeneity of soil carbon exchanges and their drivers in a boreal forest. Sci Total Environ. 831:154876.
- Sultan D, Tsunekawa A, Haregeweyn N, Adgo E, Tsubo M, Meshesha DT, Masunaga T, Aklog D, Fenta AA, Ebabu K. 2018. Impact of soil and water conservation interventions on watershed runoff response in a tropical humid highland of Ethiopia. Environ Manage. 61:860–874.
- Tahir S, Marschner P. 2016. Clay addition to sandy soil-effect of clay concentration and ped size on microbial biomass and nutrient dynamics after addition of low C / N ratio residue. J Soil Sci Plant Nutr. 16:864–875.
- Takahashi M, Hirai K, Limtong P, Panuthai S, Suksawang S, Marod D, Takahashi M, Hirai K, Limtong P. 2011. Topographic variation in heterotrophic and autotrophic soil respiration in a tropical seasonal forest in Thailand. Soil Sci Plant Nutr. 57:452–465.
- Teklay T, Nordgren A, Malmer A. 2006. Soil respiration characteristics of tropical soils from agricultural and forestry land-uses at wondo genet (Ethiopia) in response to C, N and P amendments. Soil Biol Biochem. 38:125–133.
- Thomas AD, Hoon SR, Dougill AJ. 2011. Soil respiration at five sites along the kalahari transect: effects of temperature, precipitation pulses and biological soil crust cover Botswana. Geoderma. 167-168:284–294.
- Valente FDA, Castro MF, Gomes LC, Neves JCL, Silva IR, Oliveira TS. 2021. Influence of different tree species on autotrophic and heterotrophic soil respiration in a mined area under reclamation. Land Degrad Dev. 32:1–2.
- Verchot LV, Dannenmann M, Kengdo SK, Njine-bememba B, Rufino MC, Sonwa DJ, Verchot LV, Dannenmann M, Kengdo SK, Njine-bememba B, et al. 2020. Land-use change and Biogeochemical controls of soil CO2, N2O and CH4 fluxes in Cameroonian forest landscapes. J Integr Environ Sci. 17:45–67.
- Walkley A, Black IA. 1934. An examination of the Degtjareff method for determining soil organic matter, and a proposed modification of the chromic acid titration method. Soil Sci. 37:29–38.
- Warner D, Bond-Lamberty B, Jian J, Stell E, Vargas R. 2019. Spatial predictions and associated uncertainty of annual soil respiration at the global scale. Am Geophys Union. 33:1733–1745.
- Wei H, Chen X, Xiao G, Guenet B, Vicca S, Shen W. 2015. Are variations in heterotrophic soil respiration related to changes in substrate availability and microbial biomass carbon in the subtropical forests? Sci Rep. 5:1–11.