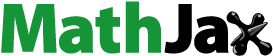
ABSTRACT
Biochar (BC) is a soil fertility enhancer, regarded as a potential carbon sequester and nutrient leaching preventer. Various studies have shown better phosphorus (P) availability with adding biochar. However, limited reporting is available on absorption–desorption behaviour of added P and dose optimisation of biochar. Rice straw biochar (RSB) and acacia biochar (ACB), prepared by slow pyrolysis at optimised temperature and pyrolysis time, were incubated at different rates (0, 5, and 10 g kg−1) in loamy sand and clay loam soils to study P sorption compared to the unamended control. The data was observed to be best fitted into Langmuir adsorption isotherm for P adsorption. The effects on maximum P adsorption, bonding energy, and adsorption rate constants were lower in loamy sand compared to clay loam soil. The values of maximum P desorption (Dm) and desorption rate constant (Kd) decreased with the application of biochar. A linear regression equation optimised the amount of P addition for soil amendments. For the soil with inherent P content, rice biochar was the best option (5 g kg−1), while for soils lacking in P, ACB is optimised at 5 g kg−1 with external P input of 1 mg P kg−1 of test soil. This data can be recommended to the farmers as a best practice for the P addition, along with managing farm wastes as biochars to enhance nutrient availability.
Introduction
Plant-available P and other nutrients are mandatory for optimum crop yields (Poulton et al. Citation2013). Application of P doses above optimum limits has caused low P use efficiency and thus an increasing environmental risk (Azizi et al. Citation2020) associated with the eutrophication of aquatic systems (Schindler et al. Citation2016). The dissolved reactive P (DRP) in drainage from high-P soils has often been higher than P concentrations associated with eutrophication (Bin et al. Citation2016). Several studies have identified a significant relationship between P loss in tile drainage and soil test P content (Osterholz et al. Citation2020). Various soil amendment strategies for sustainable agriculture require re-assessing P fertiliser application for optimum results. Since P application and uptake are subject to the type of soil and soil amendment, optimum P sorption dynamics are mandated prior to its use in a particular soil amendment project.
Biochar is a carbon-rich material produced by pyrolysis under minimal or zero oxygen supply for its application to the soil and is recognised as a sustainable methodology to improve soil properties and nutrient availability (Zhang et al. Citation2021). The addition of biochar is reported for better P availability in case of use of internal reserve of P, generally amounting above 10 Mg ha−1 for the biochar produced at temperatures lower than 600°C for significant enhancement in the P content in agricultural soils. The application of biochar to acid (pH < 6.5) and neutral soils (pH 6.5–7.5) is reported to increase plant-P availability by a factor of 5.1 and 2.4, respectively (95% confidence interval of 3.5–6.7 and 1.4–3.4, respectively), while there was no significant effect in alkaline soils (pH > 7.5), Glaser and Lehr (Citation2019).
Various studies have highlighted the affirmative impacts of biochar application on the soil and enhanced soil productivity (Takaya et al. Citation2016). The combination of nitrogen fertilisers with biochar resulted in higher fresh and dry biomass among all treatments with significant positive effects on crop growth (Rehman et al. Citation2021). The application of biochar mixed composites has significantly improved the growth, physiology, antioxidant activity, and biochemistry of maize plants in soils emanating from tanneries (Bashir et al. Citation2020). The combination of biochar and phosphorous fertilisers can be hypothesised as an effective technology for the effective assimilation of P. It needs to study sorption-desorption behaviour through kinetics studies (Zhang et al. Citation2009) to understand its losses through non-assimilative pathways of pollution (Li et al. Citation2021) and for better assimilation of phosphorus (Azevedo et al. Citation2018). Understanding P retention and release mechanisms provides vital information for effective P management to enhance crop production and sustain the soil.
Biochar has different adsorption effects (Bashir et al. Citation2021) and therefore a comprehensive approach is required to validate the biochar types and doses to obtain the best fertiliser input effects. With this view, the incubation trials were planned for soil amendments with the most feasible and available biochar prepared from rice straw and Acacia nilotica wood to present a comparative account of P sorption and desorption dynamics in clay loam and loamy sand soils under laboratory conditions. The experiment was designed and performed in triplicate to optimise the amounts of biochar application and P for the best adsorption and availability.
Material and methods
Soil and biochar
The bulk soil samples (0–15 cm) were collected from two fields under the rice-wheat system located at Punjab Agricultural University, Ludhiana research farms (loamy sand soil) and from a nearby village Barewal, Ludhiana (clay loam) in Punjab. The soil sample was air-dried and ground to pass through a 60-mesh sieve. Both soils were non-saline. The selected properties of the investigated soils are listed in .
Table 1. The initial soil properties of the experimental sites.
Rice (Oryza sativa L.) straw was collected from the research farms of Punjab Agricultural University, Ludhiana, Punjab. The straw was air-dried at room temperature and ground to pass through a 10-mesh sieve. The biochar was then prepared as per the protocol (Gupta et al. Citation2020a) at a temperature of 380°C for an incubation time of 3 h ().
The ACB was prepared using the procedure outlined in (Gupta et al. Citation2020b). Rationally, a pyramid shape structure (earth kiln) of wood is piled for its preparation. Vents are provided to allow the combustion products to escape, from the top to downwards. When smoke production is ceased, the cooling process is initiated by pouring a layer of moist earth on this structure. The cooling process completes in two to three days. Then the biochar is separated from the surrounding carbonised portions before removing the earth, finely grounded, and subsequently analyzed for the basic properties ().
Table 2. Chemical composition of rice straw biochar and acacia biochar.
Incubation experiments and treatments
The laboratory incubation study was conducted to evaluate the effect of two types of biochar (RSB and ACB) at two application rates (5 and 10 g kg−1 soil) on P adsorption and desorption in two soil types. An additional unamended control treatment was also included in the study.
Phosphorus adsorption and desorption
P adsorption studies
Five-g soil or biochar mixture samples were added to a 50-mL centrifuge tube. To each tube, 25 ml of 0.2 M KCl solution containing initial P concentrations of 1.0, 2.0, 5.0, 10, 20, 40, and 60 µg P mL−1 was added. Two drops of chloroform were added to each tube to inhibit microbial growth. The soil suspension was equilibrated for 48 h with end-over-end horizontal shaking for one hour twice a day at room temperature. The samples were centrifuged at 5000 rpm for 5 min to obtain the clear supernatant. The solution was filtered through Whatman No. 1 filter paper and the concentration of P in the filtrate was determined using the ascorbic acid yellow colour method, Watanabe and Olsen (Citation1965). The amount of P sorbed was determined as the difference between the initial amount of P added and the amount in the equilibrium solution. The P sorption data were then fitted to the Langmuir equation as follows:
(1)
(1) where C = the equilibrium P concentration (µg P ml−1), x/m = mass of P adsorbed per unit mass of soil (µg g−1 soil), b = Langmuir adsorption maxima (µg g−1); obtained from the slope, k = Langmuir bonding energy constant, i.e. the ratio of specific rate constants for adsorption and desorption at equilibrium. The constants ‘k’ and ‘b’ were obtained from the intercept and slope, respectively, of the straight-line plot of C/(x/m) versus C.
The linearised form of the Langmuir equation is:
(2)
(2) where S is the total amount of P sorbed (mg kg−1).
Phosphorus desorption studies
Soil samples used for P sorption were air-dried, and 20 mL of 0.01M CaCl2 was added. The soil was then shaken for 6 h, as described by, Singh and Jones (Citation1976). The soil suspension was centrifuged and filtered. Phosphorus concentration in the solution was determined as described above. The data obtained on P desorption was fitted in accordance with the equation.
(3)
(3) where S is the amount of P adsorbed (µg g−1), De is the amount of P adsorbed (µg ml−1), Dm is desorption maxima maximum P desorption (µg g−1 soil), and Kd is a constant related to the mobility of P in soil.
Statistical analysis
The data from the P adsorption studies were fitted to the Langmuir equation. Least–square linear regression analysis was used to compute the Langmuir equation. The multiple regression equation was used to optimise the adsorption–desorption study with the amount of added biochar and added P as independent variables using SPSS software at p ≤ 0.05.
Results and discussion
Clay loam and loamy sand under investigation were almost neutral () (pH 7.62 and 6.95, respectively) with higher P adsorption capacity than the biochar-amended soils. The rationale of this behaviour relied on alteration in soil physiological characters on biochar-mediated amendments. The prepared biochar was basic () and expectedly rose the pH of the soil (Lu et al. Citation2022). The augmented pH value results in greater negative zeta potential on soil, fixing positive ions on its surface (Méndez et al. Citation2014). For the negative ions, like phosphate ion, adsorption was low with pH rise due to electrostatic repulsive effects, Ganie et al. (Citation2021).
Moreover, the P species, stability, and dominance are pH dependent. At a pH of 7, the concentration of H2PO4- and HPO42- are approximately equal (Trazzi et al. Citation2016). The reduced adsorption with the increase in pH explicit the ion exchange mechanisms in the adsorption (Kołodyńska et al. Citation2012). With an increase in pH, OH__ competes firmly with phosphate for active sites, affecting the adsorption capacity. This implies that adsorption is favoured by low pH values, and the capacity would be higher at low pH (Xue et al. Citation2009).
Adsorption of phosphate
The phosphorus adsorption data revealed better P adsorption capacity of both the soils i.e. Clay loam and Loamy sand. The biochar-amended soils showed lower P adsorption by adding rice straw biochar (RSB) and acacia biochar (ACB). The amount of adsorbed P increased significantly with the increasing amount of P added in both soils, irrespective of the applied biochar treatment at the concentration of P (<60 mg kg−1) (). The results align with (Xu et al. Citation2014), where at lower applied P concentrations, the adsorption increased proportionately with increased P application in non-acidic soils. Biochar can also directly adsorb cations such as Al3+, Fe3+, and Ca2+, resulting in delayed P adsorption or precipitation in soil (Mrunalini et al. Citation2022). The amendment of two test soils with RSB and ACB revealed similar results where adding biochar reduced the P adsorption. The impact of amended soil properties on P retention and release depends on soil acidity or alkalinity. As evident by our results, under no basic environments, the P is fixed by high-energy sorption surfaces such as oxides and hydroxides of Fe and Al by the formation of insoluble Fe and Al phosphates by ligand exchange and precipitation reactions (Rehman et al. Citation2021), making them fixed on soil particles. Adding biochar neutralises the soil’s acidity by providing basic conditions, which makes P sparingly soluble due to the formation of metal complexes (Bacelo et al. Citation2020). These results are usually observed as low adsorption of P in soil on the amendment with biochar.
Table 3. Amount of P adsorbed (mg P kg−1) in biochar-treated soils at different levels of applied P.
The greatest amount of P was adsorbed in the unamended treatment, followed by RSB and ABC at all levels of added P in both soils. In clay loam soil (unamended), P adsorption was 0.82 mg kg−1 and 33.8 mg kg−1 showing 82.2% and 56.3% P adsorption at 1 mg kg−1 and 60 mg P kg−1, respectively. The corresponding values for loamy sand are 0.71 and 30.2 mg kg−1 with 71% and 50.3% adsorption of added P. Application of RSB and ABC at 10 g kg−1 decreased the P adsorption over the unamended control at all levels of P, except for 1 mg P kg−1 to the soil. Adding RSB (10 g kg−1) increased the amount of P adsorbed from 0.74 to 31.5 mg kg−1 in unamended treatment in clay loam soil (). Similarly, in loamy sand soil, with the addition of RSB at 10 g kg−1, P adsorption decreased from 56 to 41.6 per cent, and the amount of P adsorbed increased from 0.56 to 25 mg kg−1. At the same concentration of P in the untreated soils, the amount of P adsorbed was higher in clay loam soil than in loamy sand soil. Therefore, the amount of P adsorbed increased significantly with the increasing amount of P added in both soils, irrespective of the biochar treatment ().
Langmuir equation is experimentally proven to be a sound theoretical approach to soil phosphorus retention (Hadgu et al. Citation2014) ( and ). The present case also responded well to the Langmuir adsorption isotherm, adequately describing the P adsorption (r2 values ranged from 0.810 to 0.882 for clay loam and 0.810 to 0.962 for loamy sand soils) (). The Langmuir maximum phosphorous adsorption (b) and bonding energy constant (k) showed a wide variation among different rates of biochar application (). In both the soils where no amendment was added, the values of both adsorption maxima ‘b’ and bonding energy ‘k’ were higher compared to the addition of 10 g kg−1 RSB (). There were differences between the two types of biochar for both ‘b’ and ‘k’ constants. The significant fitting into the models stated that the adsorbate-adsorption mechanism is limited to a unimolecular layer corresponding to chemical interactions between the two counterparts. After biochar amendment, the variation in b and k reduced to 50–52.6 mg kg−1 and 0.043–0.039 L mg−1, respectively, in clay-loam soil and 39.8–34.4 mg kg−1 and 0.033–0.043 L mg−1 in loamy sand soil. These values were lower in amended soils than in unamended soils, indicating that biochar amendment could reduce soil P adsorption capacity (Han et al. Citation2018). The equilibrium between the adsorbate and adsorbent system described by Langmuir adsorption isotherm assumed uniform sorption at all binding sites. Biochar is known to respond best to Langmuir adsorption isotherm (Kilpimaa et al. Citation2014).
Figure 2. Langmuir P desorption isotherm in (A) clay loam soil, (B) Loamy sand soil. RSB: Rice straw biochar, ACB: Acacia biochar.

Table 4. Effect of biochar application on adsorption maxima (b) and bonding energy (k) in Langmuir equation in clay loam and loamy sand soil.
The results showed that the adsorption of P increased significantly with the increasing levels of added P in both soils, irrespective of the biochar treatment (). Ahmed et al. (Ahmed et al. Citation2008) also reported a similar increase in P adsorption by the soil with increasing levels of added P. Xu et al. (Citation2014) reported that P sorption data of biochar-treated soil could be described by the Langmuir equation. Applying biochar lowered the values of both adsorption maxima and bonding energy, indicating a favourable effect on P availability in the soil. However, the decrease in maximum P adsorption, which is a measure of the P adsorption capacity of soil due to biochar addition, may lead to greater leaching losses of P compared to the control. Bonding energy, which is a measure of resistance to P release in soils, also followed the same trend in both soils. These results suggested that biochar application can be crucial in keeping the applied P in a more available form by checking P adsorption.
Phosphorus desorption
The amount of P desorbed increased with the increased concentration of added P ().
Table 5. Amount of P desorbed (mg P kg−1 soil) in biochar-treated soils with various P levels.
The amount of P desorbed in the soil was far less than that of P adsorbed, indicating that desorption is a slow process and may follow different behaviour in soil than that of adsorption (). The P desorption was lower in control compared to soils amended with biochar. The soils that adsorbed a high proportion of applied P during the adsorption reaction tended to release a lower proportion during the desorption reaction and reciprocally.
Table 6. Effect of biochar on maximum P desorption (Dm) and Desorption rate (Kd) constants in clay loam and loamy sand soil.
There is disagreement as to whether sorption–desorption reactions are reversible or not (White and Taylor Citation1977). The results revealed that the amount of desorbed P was lesser than that of adsorbed P and was relatively higher under the RSB application than ACB. It has also been observed that desorbed P increased with added fertiliser P. The addition of biochar increased P desorption as compared to the control. The results agree with (Hale et al. Citation2013), where the hardwood biochar was observed to contribute significantly by reducing the P sorption capacity of the soil. The reduction in P sorption in biochar-amended soils might be due to various functional groups, such as phenolic-OH and carbonyl groups, on the surface of biochar and might have competed with P for the sorption sites (Mia et al. Citation2017).
Applying biochar compared to control soil increased the values of P desorption maximum, and higher values were recorded in soil amended with 10 g kg−1 of RSB compared to 5 g kg−1 soil. The enhanced P desorption indicates the high mobility and leaching potential of the newly sorbed P in biochar-amended soils (Singh and Gilkes Citation1991). The results explicitly point out that adsorption and desorption of applied P are inversely related, and the soils that adsorb P most readily release it the least in the soil solution. This conformed with the results reported by (Khurana Citation2001). The addition of fertiliser P decreased the resistance of soil to P release (kd), thus increasing the P supply. A study (Xu et al. Citation2014) reported that a lower dose of biochar amendment fixed the adsorbed P more strongly but did not easily desorb it.
Statistical analysis and optimisation of doses for soil amendment
The quantitative analysis of the results by multiple regression equation for RSB and ACB gave relative efficiency of both the biochars for P adsorption and desorption.
The data of adsorption in both the test soils for RSB () fitted well into the regression equation:
(4)
(4) where P represents added P and RSB = Added rice straw biochar
The equation had both the independent factors as significant (p ≤ 0.05), representing the best explanation (r2 = 97.72%) of variation of P adsorption with rice biochar amendment in terms of linear variation in adsorption with P and BC. Adding biochar, as per the equation, reduced the adsorption, but the effect was nullified by adding phosphorus (greater value of coefficient = 0.4926 than for added BC = 0.1887). The equation showed a well-fitted model even on combining the data of both the soils, inflicting that the behaviour of rice straw biochar was similar in both the test soils.
The parameters presented the best-fitted multiple linear regression model (r2 = 98.1%) at (p ≤ 0.05) for ACB as:
(5)
(5) These results are similar to RSB with slight variations in the coefficients. Since the data of both the test soils were clubbed, the results indicated that the addition of ACB had a negative impact on P adsorption. However, adding phosphorus augmented the adsorption significantly (coefficient 0.506) than rice biochar.
The quantitative analysis of desorption (dependent factor) using multiple regression equation as a statistical tool for RSB in both the test soils gave the equation:
(6)
(6) whereas for ACB, the desorption equation came out to be:
(7)
(7) where P represents the added P and BC represents the added biochar.
Both the desorption equations had a negative desorption coefficient, meaning that P retention is an inherent tendency of the soil. The desorption potential of both soils was affected significantly and proportional to the addition of biochar and added P. The RSB carries more potential for P desorption (coefficient 0.0764) than ACB (coefficient 0.0424) and RSB-amended soils responded better to the desorption on the addition of P (coefficient 0.0356) than ACB-amended soils (coefficient 0.0243).
The farmers apply biochar for soil amendment without factual data on the effective dose. These amendments carry no meaning in the light that the effect of biochar on P availability is generally inconsistent (Xu et al. Citation2014). The linear regression equation revealed that for the soils having inherent P content, the biochar addition is helpful as it decreases the adsorption of the P from the soil. Out of two test concentrations, adding 5 g kg−1 of RSB can help unlock the available P in the soil. This is the case, especially in acidic soils, where the zeta potential of the soil becomes positive and P as phosphate ions remains in electrostatic bonding with the soil particles. As per the equation, the RSB helps in P desorption better than the ACB.
Contrarily, the soils deficient in P content need different treatments. The externally added P necessitates adsorption for better retention (to reduce leaching) and desorption (for more bioavailability). These two pertinent aspects must be taken care of simultaneously for the soil amendments. The ACB became a better option when P content is to be added. From the adsorption–desorption dynamics, keeping this amount of biochar fixed at 5 g kg−1, the amount of P adsorbed and desorbed was analyzed for the maximum and minimum application of P. The data revealed that for ACB, the amount adsorbed and desorbed P came out to be the same on the application of 1 mg P kg−1 of the soil, i.e. at this concentration, the amount added will be retained and made available to the soil system as well. This dose is optimised for clay loam and loamy sand soils. Statistical tools can optimise these values for different systems by calculating the adsorption–desorption dynamics.
No data from the incubation study is available to compare our results in the present form. The results can be translated into field calculations by calculating the appropriate conversion factor by soil chemists and agronomists. The study provides valuable information on soil amendment before the actual high-cost field experimentation but needs further exploration to get meaningful conclusions for the farming community.
Conclusions
The results demonstrate that applying RSB and ACB at 5 g kg−1 soil reduced the P adsorption, Langmuir adsorption maxima (b), and bonding energy constants (k) compared to the unamended control. Maximum P desorption was higher in loamy sand than in clay loam soil due to more P release and less P retention. Applying 10 g RSB kg−1 soil will maximise the desorption of P in soils. RSB is an option for soils rich in P content as it carries the higher potential of unlocking P for better bioavailability. ACB shows the most potent effect for externally supplied P in neutral clay systems at a fixed dose of 5 g kg−1. It can help the agricultural agencies to deliver the advisories to the farmers for the appropriate dose of soil amendments by the locally available biochar. This approach, besides environmental benefits, will help reduce economic costs and gain more profit for farmers. However, further research is needed to evaluate the agronomical values at the farmer’s field scale.
Acknowledgements
Authors would like to extend their sincere appreciation to the Researchers Supporting Project number (RSP2023R194), King Saud University, Riyadh, Saudi Arabia. Conceptualisation, RKG; Methodology; Software, Validation, Writing – Original Draft Preparation. No humans and animals were used in this research.
Data availability statement
Data is contained within the article.
Disclosure statement
No potential conflict of interest was reported by the author(s).
Additional information
Funding
Notes on contributors
R. K. Gupta
Dr. R. K. Gupta, Principal Soil Chemist and former head, Department of Soil Science, Punjab Agricultural University, Ludhiana India, vast experience on integrated nutrient and crop residue management.
Monika Vashisht
Monika Vashisht is a seed testing officer in Punjab Govt. She did her MS on optimization of rice straw.
Anjali Sidhu
Dr. Anjali Sidhu, Nanotechnologist (Chemistry) is working on green synthesis of novel nanomaterials as fungicidal and health boosting material in plants.
R.K. Naresh
Dr R.K. Naresh, Professor of Agronomy, devastatingly working on conservation agriculture and water management.
Nitish Dhingra
Dr. Nitish Dhingra, Nanotechnologist (Physics), is a particle physicist working on attenuation coefficient and density of soil using gamma ray spectrometry.
Mehra S. Sidhu
Dr. Mehra S. Sidhu, Nanotechnologist (Physics) is a laser physicist working on data analytics and nano-bioprocessing of biomaterials for their optimal use in sensing applications.
Manzer H. Siddiqui
Dr. Manzer H. Siddiqui, Professor of Plant Physiology. Dr. Siddiqui's research focuses on the crop production with special emphasis on the management strategies of different fertilizers and plant growth regulators under different environmental conditions.
Saud Alamri
Dr. Saud Alamri Associated Professor of Ecophysiology. He is also interested in exploring the physiological and molecular basis of mechanisms leading to loss of pollen viability due to drought and heat stresses.
P.K. Singh
Dr P.K. Singh, Director Extension, Vallabhbhai Patel University of Agriculture, Meerut, UP India, is an extension specialist and well-known agronomist working on sustainable agriculture and crop management practices.
Md Atikur Rahman
Dr. Md Atikur Rahman has been working as a Post-Doctoral Researcher at Grassland and Forages Division, National Institute of Animal Science, Rural Development Administration (RDA), Cheonan, South-Korea since 2016. Dr. Rahman completed his BSc (Hons.) and MSc (Thesis) in Botany from the University of Rajshahi, Bangladesh securing first class. Afterward, he successfully completed his PhD in Molecular Plant Physiology from Gyeongsang National University, South-Korea in 2015. Dr. Rahman received Rural Development fellowship for post-doctoral research at Grassland and Forages Division, South-Korea. He has published more than 50 research articles in reputed international journals affiliated with world's renowned publishers, such as Nature Publishing, Elsevier, Springer, Taylor and Francis, and Wiley.
References
- Ahmed MF, Kennedy IR, Choudhury ATMA, Kecskés ML, Deaker R. 2008. Phosphorus adsorption in some Australian soils and influence of bacteria on the desorption of phosphorus. Commun Soil Sci Plant Anal. 39:1269–1294. doi:10.1080/00103620802003963.
- Azevedo RP, Salcedo IH, Lima PA, da Silva Fraga V, Lana RMQ. 2018. Mobility of phosphorus from organic and inorganic source materials in sandy soil. Int J Recycl Org Waste Agric. 7:153–163. doi:10.1007/s40093-018-0201-2.
- Azizi MZ, Yaseen M, Naveed M, Wang X, Fatima K, Saeed Q, Mustafa A. 2020. Polymer-ParaburkholderiaphytofirmansPsJN coated diammonium phosphate enhanced microbial survival, phosphorus use efficiency, and production of wheat. Agronomy. 10:1344. doi:10.3390/agronomy10091344.
- Bacelo H, Pintor AM, Santos SC, Boaventura RA, Botelho CM. 2020. Performance and prospects of different adsorbents for phosphorus uptake and recovery from water. Chem Eng J. 381:122566. doi:10.1016/j.cej.2019.122566.
- Bashir MA, Naveed M, Ashraf S, Mustafa A, Ali Q, Rafique M, Alamri S, Siddiqui MH. 2020. Performance of Zea mays L. cultivars in tannery polluted soils: management of chromium phytotoxicity through the application of biochar and compost. Physiol Plant. 173:129–147. doi:10.1111/ppl.13277.
- Bashir S, Qayyum MA, Husain A, Bakhsh A, Ahmed N, Hussain MB, Diao ZH. 2021. Efficiency of different types of biochar to mitigate Cd stress and growth of sunflower (Helianthus; L.) in wastewater irrigated agricultural soil. Saudi J Biol Sci. 28(4):2453–2424. doi:10.1016/j.sjbs.2021.01.045.
- Bin XI, Zhai LM, Jian LIU, Shen LIU, Wang HY, Luo CY, Liu HB. 2016. Long-term phosphorus accumulation and agronomic and environmental critical phosphorus levels in Haplic Luvisol soil, northern China. J Integr Agric. 15:200–208. doi:10.1016/S2095-3119(14)60947-3.
- Ganie ZA, Khandelwal N, Tiwari E, Singh N, Darbha GK. 2021. Biochar-facilitated remediation of nano plastic contaminated water: effect of pyrolysis temperature-induced surface modifications. J Hazard Mater. 417:126096. doi:10.1016/j.jhazmat.2021.126096.
- Glaser B, Lehr VI. 2019. Biochar effects on phosphorus availability in agricultural soils: a meta-analysis. Sci Reports. 9:9338–9346.
- Gupta RK, Hussain A, Sooch SS, Kang JS, Sharma S, Dheri GS. 2020a. Rice straw biochar improves soil fertility, growth, and yield of rice–wheat system on sandy loam soil. Exp Agric. 56:118–131. doi:10.1017/S0014479719000218.
- Gupta RK, Kang JS, Singh H. 2020b. Adopt straw management practices to enhance soil health and crop productivity. Progress Farming. 56(10):10–21.
- Hadgu F, Gebrekidan H, Kibret K, Yitaferu B. 2014. Study of phosphorus adsorption and its relationship with soil properties analyzed with Langmuir and Freundlich models. Agri For Fish. 1:40. doi:10.11648/j.aff.20140301.18.
- Hale SE, Alling V, Martinsen V, Mulder J, Breedveld GD, Cornelissen G. 2013. The sorption and desorption of phosphate-P, ammonium-N and nitrate-N in cacao shell and corn cob biochars. Chemosphere. 91:1612–1619. doi:10.1016/j.chemosphere.2012.12.057.
- Han Y, Choi B, Chen X. 2018. Adsorption and desorption of phosphorus in biochar-amended black soil as affected by freeze-thaw cycles in Northeast China. Sustainability. 10(5):1574. doi:10.3390/su10051574.
- Khurana HS. 2001. Kinetics of phosphorus release from soils fertilized with various phosphatic fertilizers materials under different cropping systems. M.Sc. Thesis, Punjab Agricultural University, Ludhiana, Punjab.
- Kilpimaa S, Runtti H, Kangas T, Lassi U, Kuokkanen T. 2014. Removal of phosphate and nitrate over a modified carbon residue from biomass gasification. Chem Eng Res Des. 92:1923–1933. doi:10.1016/j.cherd.2014.03.019.
- Kołodyńska D, Wnętrzak R, Leahy JJ, Hayes MHB, Kwapiński W, Hubicki ZJCEJ. 2012. Kinetic and adsorptive characterization of biochar in metal ions removal. Chem Eng J. 197:295–305. doi:10.1016/j.cej.2012.05.025.
- Li F, Jin Y, He S, Jin J, Wang Z, Khan S, Liang X. 2021. Use of polyacrylamide-modified biochar coupled with organic and chemical fertilizers for reducing phosphorus loss under different cropping systems. Agric Ecosyst Environ. 310:107306. doi:10.1016/j.agee.2021.107306.
- Lu HL, Li KW, Nkoh JN, He X, Hong ZN, Xu RK. 2022. Effects of the increases in soil pH and pH buffering capacity induced by crop residue biochars on available Cd contents in acidic paddy soils. Chemosphere. 301:134674. doi:10.1016/j.chemosphere.2022.134674.
- Méndez JP, García FP, Sandoval OAA, Marzo MAM. 2014. Correlation of Moisture, pH and Zeta Potential ([Zeta]) in Sandy-Clay-Loam Soils of the Southern State of Hidalgo, Mexico. Asian J Chem. 26:3001. doi:10.14233/ajchem.2014.16343.
- Mia S, Dijkstra FA, Singh B. 2017. Ageing induced changes in biochar’s functionality and adsorption behaviour for phosphate and ammonium. Env Sci Technol. 51:8359–8367. doi:10.1021/acs.est.7b00647.
- Mrunalini K, Behera B, Jayaraman S, Abhilash PC, Dubey PK, Swamy GN, SrinivasaRao C. 2022. Nature-based solutions in soil restoration for improving agricultural productivity. Land Degrad Dev. 33:1269–1289. doi:10.1002/ldr.4207.
- Osterholz WR, Hanrahan BR, King KW. 2020. Legacy phosphorus concentration–discharge relationships in surface runoff and tile drainage from Ohio crop fields. J Environ Qual. 49:675–687. doi:10.1002/jeq2.20070.
- Poulton PR, Johnston AE, White RP. 2013. Plant-available soil phosphorus. Part I: the response of winter wheat and spring barley to Olsen P on a silty clay loam. Soil Use Manag. 29:4–11. doi:10.1111/j.1475-2743.2012.00450.x.
- Rehman M, Saleem MH, Fahad S, Bashir S, Peng D, Deng G, Alamri S, Siddiqui MH, Khan SM, Shah RA. 2021. Effects of rice straw biochar and nitrogen fertilizer on ramie (Boehmeria nivea L.) morpho-physiological traits, copper uptake and post-harvest soil characteristics, grown in an aged-copper contaminated soil. J Plant Nutr. 45:11–24. doi:10.1080/01904167.2021.1943675.
- Schindler DW, Carpenter SR, Chapra SC, Hecky RE, Orihel DM. 2016. Reducing phosphorus to curb lake eutrophication is a success. Environ Sci Technol. 50:8923–8929. doi:10.1021/acs.est.6b02204.
- Singh B, Gilkes RJ. 1991. Phosphorus sorption in relation to soil properties for the major soil types of South-Western Australia. Soil Res. 29:603–618. doi:10.1071/SR9910603.
- Singh BB, Jones JP. 1976. Phosphorus sorption and desorption characteristics of soil as affected by organic residues. Soil Sci Soc Am J. 40:389–394. doi:10.2136/sssaj1976.03615995004000030025x.
- Takaya CA, Fletcher LA, Singh S, Anyikude KU, Ross AB. 2016. Phosphate and ammonium sorption capacity of biochar and hydrochar from different wastes. Chemosphere. 145:518–527. doi:10.1016/j.chemosphere.2015.11.052.
- Trazzi PA, Leahy JJ, Hayes MH, Kwapinski W. 2016. Adsorption and desorption of phosphate on biochars. J Environ Chem Eng. 4:37–46. doi:10.1016/j.jece.2015.11.005.
- Watanabe FS, Olsen SR. 1965. Test of an ascorbic acid method for determining phosphorus in water and NaHCO3 extracts from soil. Soil Sci Soc Am J. 29(6):677–678. doi:10.2136/sssaj1965.03615995002900060025x.
- White RE, Taylor AW. 1977. Reactions of soluble phosphate with acid soils: the interception of adsorption-desorption isotherm. J Soil Sci. 28:314–328. doi:10.1111/j.1365-2389.1977.tb02240.x.
- Xu G, Sun J, Shao H, Chang SX. 2014. Biochar had effects on phosphorus sorption and desorption in three soils with differing acidity. Ecol Eng. 62:54–60. doi:10.1016/j.ecoleng.2013.10.027.
- Xue Y, Hou H, Zhu S. 2009. Characteristics and mechanisms of phosphate adsorption onto basic oxygen furnace slag. J Hazard Mater. 162:973–980. doi:10.1016/j.jhazmat.2008.05.131.
- Zhang W, Faulkner JW, Giri SK, Geohring LD, Steenhuis TS. 2009. Evaluation of two Langmuir models for phosphorus sorption of phosphorus-enriched soils in New York for environmental applications. Soil Sci. 174:523–530. doi:10.1097/SS.0b013e3181be9a4c.
- Zhang Y, Wang J, Feng Y. 2021. The effects of biochar addition on soil physicochemical properties: a review. Catena. 202:105284. doi:10.1016/j.catena.2021.105284.