Abstract
Purpose
The Organisation for Economic Co-operation and Development (OECD) Adverse Outcome Pathway (AOP) Development Programme is being explored in the radiation field, as an overarching framework to identify and prioritize research needs that best support strengthening of radiation risk assessment and risk management strategies. To advance the use of AOPs, an international horizon-style exercise (HSE) was initiated through the Radiation/Chemical AOP Joint Topical Group (JTG) formed by the OECD Nuclear Energy Agency (NEA) High-Level Group on Low Dose Research (HLG-LDR) under the auspices of the Committee on Radiological Protection and Public Health (CRPPH). The intent of the HSE was to identify key research questions for consideration in AOP development that would help to reduce uncertainties in estimating the health risks following exposures to low dose and low dose-rate ionizing radiation. The HSE was conducted in several phases involving the solicitation of relevant questions, a collaborative review of open-ended candidate questions and an elimination exercise that led to the selection of 25 highest priority questions for the stated purpose. These questions were further ranked by over 100 respondents through an international survey. This final set of questions was judged to provide insights into how the OECD’s AOP approach can be put into practice to meet the needs of hazard and risk assessors, regulators, and researchers. This paper examines the 25 priority questions in the context of hazard/risk assessment framework for ionizing radiation.
Conclusion
By addressing the 25 priority questions, it is anticipated that constructed AOPs will have a high level of specificity, making them valuable tools for simplifying and prioritizing complex biological processes for use in developing revised radiation hazard and risk assessment strategies.
Introduction
The Organisation for Economic Cooperation and Development (OECD) launched the Adverse Outcome Pathway (AOP) Development Programme in 2012 following a call to modernize toxicity testing of chemicals intended for industrial uses (NRC Citation2007; Seidle and Stephens Citation2009; Krewski et al. Citation2010). A framework was created within which multiple types of data characterizing toxicants could be combined and synthesized for subsequent use by hazard/risk assessors and risk management authorities. The overall approach provides an understanding of critical events across multiple levels of biological organization anchored to an adverse health or biological outcome of interest to regulatory decision-making (Ankley et al. Citation2010).
An AOP is defined by an adverse outcome (AO), the induction of which is described by a series of key or necessary events across multiple biological levels of organization. This progression is considered to arise from an exposure-related molecular initiating event (MIE) along the pathway via cellular, tissue and/or organ and organismal levels, with each step being associated with one or more key events (KEs). Thus, these KEs provide a proposed connectivity from the MIE to an AO. In the process, qualitative, semi-quantitative or quantitative data are used to support the causality of linked KEs, through the development of key event relationships (KERs). The modular structuring of available knowledge is then deposited within the AOP-Wiki (https://aopwiki.org/) where it undergoes open scientific peer-review for assessment of essentiality, plausibility and empirical evidence utilizing the modified Bradford-Hill criteria, to determine the strength of an association between the AO and its presumed causative agent (Becker et al. Citation2015). The AOP framework also provides traceability and transparency in the creation, approval, review and endorsement process of new AOPs (OECD Citation2021).
In June 2021, the OECD Nuclear Energy Agency (NEA) Committee on Radiological Protection and Public Health (CRPPH) established the High-Level Group on Low Dose Research (HLG-LDR) Radiation (Rad)/Chemical (Chem) AOP Joint Topical Group (JTG) (Chauhan, Beaton et al. Citation2022). The JTG aims to promote AOP use and its integration into planning and outcome interpretation of radiation research and subsequently into the hazard/risk assessment process. To meet this goal, a horizon-style exercise (HSE) was initiated in September 2021. The overall objective of this HSE was to generate a shortlist of priority questions that, if addressed through research programs, would help define the strengths and constraints of the AOP framework as applied to low dose/low dose-rate hazard/risk assessment. Thus, the goal of the AOP JTG is that uncertainties in estimating the risk of adverse human health and ecological outcomes from exposures to low dose and low dose-rate ionizing radiation will be reduced.
The process and methodology used in generating the shortlist of 25 priority questions and survey results are described by Burtt et al. (Citation2022). Briefly, survey respondents were presented with a randomized survey and repeatedly shown a list of four of the 25 questions for ranking. Aggregate responses revealed the relative importance of the 25 questions based on counts and a hierarchical Bayesian score. Although no specific questions were clearly identified to be significantly more important and separated from the list of 25 questions, the list can guide the future program of work of the JTG. The 25 questions relate generally to: (1) how the use of AOPs can improve existing approaches to hazard/risk assessment; (2) methods for evidence gathering and review; (3) defining AOP components; (4) AOP networks, radiation phenomena (e.g., non-targeted effects), multigenerational effects, dual property stressors; (5) individualized risk assessments (6) AOP communication, acceptance, and future directions (). This is a follow-up paper to Burtt et al. (Citation2022) which discusses the 25 questions in the context for the use of AOPs in a hazard/risk assessment framework for ionizing radiation. The 25 questions are thematically grouped into six main categories as described above and examined in this context.
Figure 1. Twenty-five key questions categorized to guide future areas of AOPs in radiation research and policy work.
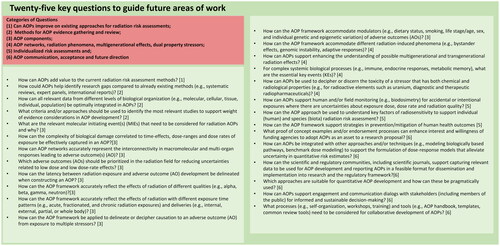
How can the use of AOPs improve existing approaches to hazard/risk assessment?
Central among the 25 questions from the HSE is to understand how AOPs have added value to existing approaches used in the interpretation of radiation risk estimates and the associated uncertainties at low doses and dose-rates. Over the last few decades, an abundance of biological data has been generated to explore the mechanisms of radiation-induced effects in both humans and non-human biota. These data complements the wealth of information gathered from epidemiological and field (monitoring) studies, offering a strengthened foundation for radiation regulatory decision-making. Currently however, there is a general lack of integration of the diverse sets of data into a holistic and mechanistically informed hazard assessment framework. Such integration is crucial to make the fullest use of the most relevant data and scientific knowledge to support radiation hazard/risk assessments (NCRP Citation2020).
The field of radiation protection also faces the significant challenge of predicting health risks associated with low linear energy transfer (LET) exposures at low doses (less than 100 mGy) and low dose-rates (<0.1 mGy/min or <6 mGy/h) (UNSCEAR Citation2010; Rühm et al. Citation2015). At present, estimates of radiation-induced adverse health effects in humans largely rely on epidemiological studies that analyze cancer and non-cancer endpoints in various exposed populations, notably survivors of the Hiroshima and Nagasaki atomic bombs and individuals with known or estimated doses from medical, occupational, and environmental exposures (ICRP Citation2012; Little et al. Citation2018; NCRP Citation2020; Little et al. Citation2022; Hamada & Zablotska Citation2023; Hauptmann et al. Citation2023). Past research has provided valuable data to quantify the relationship between radiation absorbed dose and cancer risk, including the impact of modifying factors like sex or age at exposure. Recent studies have also yielded significant results for cancer (Rühm et al. Citation2022; Hauptmann et al. Citation2023; Richardson et al. Citation2023) and non-cancer effects (Little et al. Citation2020, Citation2023; Hamada, Citation2023) in the low dose range. While the data implies non-linear dose associations for specific cancers, overall, the linear-non-threshold (LNT) model is generally considered not to appreciably overestimate the hazards at low doses (NCRP Citation2018; Laurier et al. Citation2023). The influence of mechanisms that are not rooted in gene mutations on the sensitivity to radiation-induced cancer at low doses remains a complex evaluation. Addressing this challenge is essential for reducing uncertainties in selecting specific models for specific types of cancer used in risk assessment (ICRP Citation2007; UNSCEAR Citation2021).
Interpreting human cancer data in the low dose range presents challenges due to variation of high baseline cancer rates, confounding factors such as lifestyle, preexisting diseases, age, sex, ethnicity, multiple exposures, uncertainties in exposure and dose reconstruction, as well as a lack of sufficiently large population datasets to achieve statistical power. The quality and quantity of biological studies at low dose exposures also make it more difficult to understand the mechanistic relationship between exposure and effect. Addressing these uncertainties requires more research. The involvement of prior radiation exposures (or other exposure types, e.g., radiation types, chemical stressors) further complicates accurate risk interpolations, limiting the understanding of how specific exposures contribute to cancer outcomes (ICRP Citation2007, Citation2012; Cool et al. Citation2019; Hauptmann et al. Citation2020). The consideration of non-cancer risks from radiation exposures is an evolving field of research, some of which have been judged as tissue reactions with a dose-response threshold (ICRP Citation2012; Hamada Citation2023).
To complement human epidemiological data and address their associated uncertainties, assessment of adverse risk may benefit by incorporating more mechanistically informed knowledge generated from laboratory animal and cellular/molecular radiobiological studies. The adoption and use of AOPs (Ankley et al. Citation2010) can be instrumental in this effort as it serves to prioritize the most reliable mechanistic data to derive mechanistically informed risk models as well as to support the existing biologically-based, epidemiology-derived models of cancer and non-cancer diseases, notably for cardiovascular diseases (Simonetto et al. Citation2022). Such models termed, biologically-based dose-response (BBDR) models (Preston Citation2017) provide an important interface by harnessing the biological information from AOPs for quantitative risk assessment (Stainforth et al. Citation2021).
AOPs can also serve as one practical solution for managing the vast amounts of data derived from radiobiological research conducted in diverse fields such as environmental, occupational, medical, and space exploration research. These AOPs can be constructed initially using data from epidemiological and field studies and integrating both traditional endpoints and information obtained from newer high throughput technological advancements like broad-content analysis (e.g., omics). By defining AOPs across various biological levels of organization, taking into consideration different stressors and organisms, this integrated approach can effectively organize decades of research into an informative framework for effects of single and multiple stressors that affect different toxicity pathways leading to adverse effects of relevance to risk assessment (e.g., cancer and non-cancer diseases).
With the systematic organization of information, several steps can be undertaken to increase confidence in the data and evidence before utilizing that endpoint to predict an AO for risk assessment purposes. This includes a thorough evaluation of the data quality, conducting validation studies to confirm the reliability and reproducibility of the endpoint measurement methods, and exploring potential confounding factors or uncertainties. Furthermore, consideration of species extrapolation, can enhance the confidence in the predictive value of the endpoint. Collaborative experimental studies can be coordinated across institutions to gather data for integration into reliable quantitative risk models, using the most relevant KEs identified from AOPs. The interconnected data in AOPs, covering various diseases and biological levels, will further provide insights into the applicability of these models across animal species and demographics (e.g., sex, age, species) and stressor initiation parameters (e.g., absorbed dose, dose-rate, period elapsed following exposure to initial dose). This information can then be utilized to:
reduce (or address) uncertainties associated with current risk estimates;
identify bioindicator/biomarkers/endpoints/test methods most informative for human and environmental/ecological health (safety) assessments;
identify endpoints related to KEs/KERs that are influenced by confounding factors (e.g., age, sex);
identify synergistic/additive/antagonistic effects and biological mechanisms relevant for adversity progression from complex exposure scenarios (e.g., multiple stressors);
provide opportunity for more mechanistically informed knowledge dissemination to international radiation protection governing bodies.
These aspects are viewed as essential for increasing the robustness of radiation dose criteria (e.g., dose limits, reference levels) for protection purposes. Although examples provided illustrate relevance for human health assessments, the challenges and applications of AOPs presented are equally applicable to environmental health assessments.
Methods for AOP evidence gathering and review
The credibility of the AOPs developed heavily depends on the process of evidence gathering and synthesis. A robust and systematic approach for gathering evidence is essential to ensure that the information used to construct AOPs is of high quality, unbiased, scientifically sound, and able to assess accurately the certainty. The HSE identified four questions specific to the approach for evidence gathering to support the weight of evidence (). The strength of an AOP comes from the evidence evaluation informed from studies that meet the modified Bradford-Hill criteria (Becker et al. Citation2015). These criteria originally proposed for epidemiological assessments use nine considerations (strength, consistency, specificity, time concordance, incidence-concordance, biological plausibility, biological gradient, dose-concordance, and coherence). Of these, four (biological plausibility, dose-, time-, incidence-concordance) support AOP construction alongside the essentiality of KEs (Becker et al. Citation2015). This approach differs from how information is presented and assessed in systematic reviews or reports generated by international regulatory organizations. These latter reports serve to distill available knowledge to address a precisely defined research question. AOPs possess a broader scope and offer a graphical view for depicting and conveying the sequence of biological events and interactions that arise from an MIE – such as the deposition of energy – and lead to more complex AOs, such as organ toxicity, as well as identification of causal connections based on the Bradford-Hill criteria. Within an AOP narrative, the empirical substantiation underpinning the AOP is summarized, and information on modulators, the range of applicability across each KER, and even the prototypic stressors that trigger the perturbation of events along the AOP are presented. The comprehensive detailing of empirical evidence, critical for establishing a mechanistic understanding, forms a foundation for constructing and supporting risk assessment models and tools. The AOP approach can therefore be seen as complementary to approaches such as systematic and expert organization reviews.
Although many criteria and/or approaches could be used to identify the most relevant studies for providing evidence for AOP development, systematic tools alongside expert consultations are key to capturing the most relevant data in support of a specific AOP (). Working groups formed through a workshop organized by the Multidisciplinary European Low Dose Initiative (MELODI) and the European Radioecology Alliance associations held in 2021, highlighted how bringing experts together on a particular topic area could facilitate the process of identifying potential KEs in an AOP (Chauhan, Hamada et al. Citation2021; Chauhan, Villeneuve et al. Citation2021; Azimzadeh et al. Citation2022; Jaylet et al. Citation2022; Klokov et al. Citation2022; Tollefsen et al. Citation2022). Artificial intelligence (AI) typically using various forms of machine learning (ML) and natural language processing (NLP), may also help distill data to support AOP development (Jaylet et al. Citation2023). AI can automate literature screening to improve efficiency, potentially playing a role in identifying informative data across different levels of biological organization as seen in tools such as the AOP-helpFinder (Jornod et al. Citation2022).
Figure 2. Hypothetical example of how radiation exposures conditions (acute or chronic) can lead to a common molecular initiating event of deposition of energy (DOE) to multiple different key events (KEs) depending on the radiation exposure parameters. Not all KEs are sufficient to progress to adverse outcome (AO). Red X represents a no-go to the AO, the blue zig zag represents multiple KEs. For the purposes of this example low dose and low dose-rate refers to <100 mGy/<0.1 mGy/min and high dose and high dose-rate refers to >1Gy/>0.01 Gy/min.
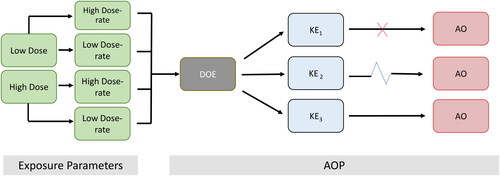
For efficient AOP development, an investment in resources and tools used to transparently identify the most informative data, including documenting the collated information alongside the appropriate evaluation through expert consultation is essential (Chauhan, Wilkins et al. Citation2021). Systematic review tools are available to help increase efficiency of the workflow (Leist et al. Citation2017; Svingen et al. Citation2021), and different approaches spanning from narrative reviews to more advanced approaches such as scoping reviews and full systematic reviews are currently being explored. A recent scoping review example in the radiation field (Kozbenko et al. Citation2022) provides steps to retrieve relevant studies in a transparent and documented manner, without being resource intensive. The process involves four levels of screening using automated tools beginning with a prioritization step that narrows down the literature search to select the most relevant peer-reviewed studies, followed by screening based on title and full text using a defined “population, exposure, endpoint and outcome” statement, and data extraction for studies that meet the Bradford-Hill criteria.
Conducting full systematic reviews may be somewhat challenging due to the diversity of data that is used to support AOPs and the broad scope for risk of bias analysis. Although narrative reviews are considered the default, these may come with certain types of biases and lack of transparency in data retrieval. Therefore, other formats such as scoping reviews may serve the purpose and could help identify biases in the evidence gathering and/or interpretation process. Discussions on this topic continue among AOP developers, and in the field of scientific review in general, to develop effective, transparent, and standardized approaches. Development of case studies that use different systematic tools and more automated evidence gathering (e.g., AI-informed approaches), will likely emerge to demonstrate standardized and fit-for-purpose approaches for robust data retrieval for AOP development. By following a rigorous evidence-gathering process, AOPs can be constructed with a high level of specificity. The validity of any AOP can be regularly updated and revised to reflect the advancements in scientific knowledge and understanding. This dynamic nature ensures that regulatory decisions are based on the most reliable and credible information available.
AOP components
The HSE identified multiple questions on a) the representative MIE for radiation exposure; b) the most essential KEs and how parameters such as time-effects could be deciphered within the AOPs construct and c) the interpretation of more complex biological processes (e.g., inflammation). Together 8 key questions () related to AOP components were seen as essential for better characterizing the necessary steps along the pathway from an MIE to an AO alongside the necessary KEs at the level of cells, tissues, or organs along the AOP.
MIE
Identifying an MIE for radiation exposures over a range of doses, dose rates and radiation types is considerably less complex than it is for environmental chemicals for which the AOP construct was originally proposed. There are numerous MIE’s that have been identified for chemicals with several being quite plausible for any chemical or chemical class. For ionizing radiation, the initial interaction with the cell is stochastic and likely multiple in nature. Therefore, incorporating a physical MIE for radiation AOPs would universally capture the effects of primary ionization events and then also accurately describe the correlated events that occur following exposure to any radiation stressor on targeted and non-targeted cells (Preston Citation2017).
For the recent NEA-endorsed lung cancer AOP (Sherman et al. Citation2023), an MIE of “deposition of energy” was selected as it was proposed to accurately reflect the first event that initiates the adverse effect of interest and to be most relevant to radiation stressors (Chauhan, Sherman et al. Citation2021). Deposition of energy (https://aopwiki.org/events/1686) is measurable and is supported through the decades of historical data for numerous ensuing KEs. The selection of the MIE (and other AOP events for that matter) would largely benefit by following a definition principle of “as wide as possible, but as specific as needed” to ensure flexibility to incorporate other stressors that share one or more events along the AOP continuum, stimulate reuse and reduce redundancy of events and event descriptions. This is amply demonstrated by the coherence between AOPs and toxicity pathways for ionizing radiation and non-ionizing radiation (e.g., UVB) in various species (Song et al. Citation2020; Cao et al. Citation2023).
Relevant KEs, complex biology
Due to the non-linear dose response for many radiation effects and the uncertainty surrounding the magnitude of low-dose effects, concerns have been raised about the oversimplification of AOPs and the associated KEs for defining health effects at low doses. What is needed is a systematic approach for establishing the relevant biology under a range of exposure scenarios for AOs relevant hazard/risk assessment scenarios. For such a requirement, the AOP approach is clearly valuable as it helps identify the essential early KEs in a pathway that are crucial for understanding the biological responses to radiation exposure. The necessity of a KE for a specific AO can be assessed by its essentiality, such as addressing how inhibiting or modulating the KE can affect a downstream event linked to the AO of interest. It is such KEs that can be utilized as parameters in a BBDR model to allow for extrapolation from higher doses at which biological events can be measured to doses relevant for protection standards (NCRP Citation2020). As an example, for the recent development of a space AOP network for non-cancer health outcomes (AOP# 483 (https://aopwiki.org/aops/483); 478 (https://aopwiki.org/aops/478); 482 (https://aopwiki.org/aops/4820; 470 (https://aopwiki.org/aops/470)), some common macromolecular events were identified across the varied outcomes of vascular effects, cataracts, bone loss and cognitive deficits (Carrothers et al. Citation2024). The KEs within the AOP network are common for a range of radiation-induced injuries and could be categorized into compensatory mechanisms (e.g., DNA repair, reduction in antioxidant potential), direct damage to macromolecules (e.g., DNA, proteins), peripheral systemic events i.e., inflammation and immune suppression) and overt phenotypic effects (e.g., organ dysfunction, remodeling). As such, these AOPs have already given knowledge gap insights into mechanistic understanding of non-cancer effects. Translating these to radiation protection practices could be achieved with both additional studies and data in the form of dose and time-concordance assessments. More directed research in these areas could be a future priority.
While biological approaches are challenged when being overly reductionist, the OECD recognizes these hurdles and is working toward approaches to integrate complex processes within AOPs. A recent consortium called the “mystery of reactive oxygen species”, contemplated how oxidative stress, an overarching KE within the framework in many diseases, requires refinement and focus on its descriptions to capture mechanistic understanding (Tanabe, Beaton et al. Citation2022; Tanabe, O’Brien et al. Citation2022), and thus promote a common understanding of KE ontology. This type of consensus approach is recommended for any types of KEs that are characterized as being too broad and complex (e.g., immune response) to be defined as one KE. Therefore, where consensus on a single KE is difficult to identify, the best approach in developing an AOP is to characterize it through multiple diverse endpoints (e.g., radical formation, antioxidant production, oxidative macromolecular damage) connected within a broader KE (a super or umbrella KE).
Radiation quality dose/dose-rate effects
A feature of the AOP framework is that it is “stressor agnostic”, meaning that an AOP is not limited to a specific type of stressor or exposure thus enabling new and emerging stressors to be categorized by their mechanistic profiles. Under the AOP construct, knowledge of the primary energy deposition events (e.g., during the physical and physicochemical stages of interaction of radiation with biological matter) and the magnitude and nature of biological responses they lead to will be influenced by the dose, the dose-rate, and the radiation type (Azzam et al. Citation2012; Rühm et al. Citation2015). Different radiation types may trigger the same linear AOP but have different trajectories to the AO depending on the characteristics of a specific radiation type. Such parameters are “exposure parameters” () and will only directly influence the first critical KE following initiation from deposition of energy. However, once the dose (dose-rate)-response and time-response relationships across KERs become more clearly defined (e.g., the responses/KERs are likely to be strongly dependent on the microenvironment of the impacted tissue), it will be feasible to construct a quantitative AOP, which will then fully account for the exposure parameters.
Time effects
The HSE also identified a prioritized question on how the AOP approach and framework could account for the induction and latency periods or the time from the exposure to disease initiation/detection and then to (clinical or field) manifestation of health effects (e.g., AO). By focusing on latency, the assumption is that the disease state exists but has not been detected or is at the level of being detected. In radiation protection, acute early effects and early tissue reactions observed within days to a few months following an acute exposure to high doses are reasonably well understood, although some tissue reactions and stochastic effects may have extended latency periods (months to decades). It is feasible that an AOP could be instructive in predicting the frequency of late tissue reaction effects by utilizing KEs such as considering and comparing the time-course of KEs occurring early along the pathway (predictive) to those that occur later, as assessed using time-concordance data.
Prioritized AOs
The HSE also generated questions on which AOs can be considered as a priority for radiation protection purposes based on health and ecological impacts. Priority setting for AOs from a regulatory perspective can be established from radiation-exposed populations (UNSCEAR Citation2010). Aside from cancer, Publication 118 of the International Commission on Radiological Protection (ICRP) emphasizes that research should also be directed to the better understanding of radiation-induced “tissue reactions” such as those occurring in the eye, circulatory, respiratory, nervous, and reproductive systems (ICRP Citation2012). In the coming years, these areas are expected to be the primary focus for radiation-AOP development, with relevance to both humans and wildlife. In support of this view, efforts are underway through the United Nations Scientific Committee on the Effects of Atomic Radiation (UNSCEAR) and ICRP to better understand radiation-induced tissue reactions particularly regarding the responses at low doses. On a separate note, future research will also extend ongoing work in radioecology where AOs such as effects on growth development, survival and reproduction are key apical outcomes relevant to population dynamics.
AOP networks, radiation-induced phenomena, multigenerational effects, dual property stressors
The HSE identified 4 questions () pertinent to understanding complex biological processes, radiation-induced phenomena, dual-property stressors, and multigenerational/transgenerational effects that need to be addressed to enhance the application of AOPs.
Complex biological processes
AOP networks offer a structured and simplified way to represent complex biological processes. AOP networks are composed of multiple AOPs that share common KEs and KERs and can also provide a record of a multitude of potential events for the subsequent identification of those being key to low dose (and low dose-rate) perturbations when compared with those triggered at higher doses (and dose-rates). AOP networks identify relationships, dependencies, and interactions among various biological processes that contribute to an AO. An AOP network is also a potential map for multiple stressor effects as stressors may be characterized by different AOPs and share events along the AOP continuum (Beyer et al. Citation2014; Salbu et al. Citation2019; Xie et al. Citation2022). Thus, the AOP networks provide an attractive means to simplify complex biological processes triggered by multiple stressors leading to one common or more diverse sets of AOs.
Radiation-induced phenomena
The HSE also led to important questions on how radiation-induced phenomena such as bystander effects, genomic instability, and adaptive responses, collectively termed as non-targeted effects (Hamada et al. Citation2011; Matsumoto et al. Citation2011) could be integrated into AOPs. These effects may be considered as “modulators”, potentially acting across select KEs/KERs in an AOP. Although these phenomena are important for mechanistic understanding, and there is ample evidence for their existence in laboratory experiments, they have not been implicated in radiation cancer risk (UNSCEAR Citation2021). At present, there are no clear examples within the AOP-Wiki where radiation AOPs have clearly been used to address these complex ‘non-targeted effects’. The absence of such AOPs, however, should not be interpreted as lack of relevance, but rather a reflection that the radiation AOP developer community, albeit still small, has focused on less complex radiation effects where information is more widely documented. Generally, if mechanisms in the context of radiation protection are not clear due to significant inconsistency in experimental findings and lack of experimental reproducibility in data, there will be a limit to their applicability for use in AOPs. In addition, it needs to be established as to how these non-targeted effects might play a role in informing risks for the protection of humans and non-human biota.
Modulating factors
A notable challenge with any type of biological or epidemiological study is the influence of modifiers on the estimated association between exposure and an AO. To account for modulating factors, the AOP framework described in the AOP handbook (OECD Citation2018) includes sections that can inform the domain of applicability (e.g., sex, species). Although modulating factors are important attributes that need to be captured in the building of qualitative AOPs, currently no method has been implemented to incorporate these parameters in quantitative response-response relationships for AOPs. However, models such as the sufficient component cause (SCC) model (Flanders et al. Citation2006) are being explored for environmental toxicants and Bayesian networks (BNs) (Moe et al. Citation2021, Cao et al. Citation2023) serve as examples of how relationships between direct and indirect events leading to an AO can be characterized. The SCC model recognizes that, on its own, a stressor does not necessarily result in a disease outcome and that a minimal set of conditions needs to be present (KEs) and these will be variable among individuals. The BNs are graphical models that represent probabilistic relationships among variables, and useful for understanding complex systems of multiple interacting factors. Both SCC and BN model may be translatable to quantitative AOPs, and modifiers could be included amongst the component causes leading to the AO (Rothman and Greenland, Citation2005; Moe et al. Citation2021).
Dual property compounds
Dual-property compounds that have both radiological and chemical effects such as radioactive elements (e.g., uranium, plutonium, thorium), or diagnostic and therapeutic radiopharmaceuticals, can be represented in AOPs based on their overall biological effects, rather than solely on the toxicity induced by their individual physical or chemical properties. This approach is necessary because the perturbation of MIEs, KEs, and the eventual AO is often integrative in nature when dealing with such compounds. While these stressors may have different initiating events, they can either converge or diverge to produce similar or dissimilar KEs and AOs. Therefore, it is important to consider the overall biological impact of a compound considering the combined effects of its chemical and physical properties that accounts for the divergent and similar KEs. For example, as an alpha particle emitter, uranium can induce dense ionization events leading to oxidative stress and bind to macromolecules due to its metal ion properties (Sabolić Citation2006; Guéguen and Frerejacques Citation2022). Both of these actions contribute to the overall toxicity of uranium. Therefore, understanding the integrated dose-response relationships for both the chemical and radiation properties is essential when assessing the overall impact of dual-property compounds, which helps advise the health protection approaches. However, if experimental data consistently highlights distinct mechanistic aspects of the individual isotopes of dual-property elements, then these delineations can be represented as new and separate KEs in an AOP and independent risk models derived could be used to assess additive risk.
Transgenerational/multigenerational effects
AOPs have the potential to enhance an improved understanding of multigenerational and transgenerational radiation effects. Incorporating these effects into AOPs can enhance our understanding of the broader impact of exposures on biological systems and provide insights into the long-term consequences of exposures. In addition to the germ-line transmission of genetic mutations, transgenerational effects such as transmission of changes in phenotype or gene expression may involve epigenetic modifications, such as DNA methylation or histone modifications, that can be passed on to subsequent generations. Incorporation of epigenetic and other transgenerational processes is still in its infancy, but efforts to demonstrate how these effects may be included in an AOP are underway (Thaulow et al. Citation2020; Song et al. Citation2021).
Multigenerational effects extend beyond transgenerational effects by spanning multiple generations, often involving exposures and responses that affect more than just the immediate offspring. AOPs can be extended to include a series of KEs that account for the effects observed in multiple generations. For instance, an AOP representing a multigenerational effect might include KEs related to heritable mutations in the first generation, with subsequent non-genomic transferable effects characterized as KEs in the second generation. Currently the AOP-Wiki has an endorsed AOP (e.g., https://aopwiki.org/aops/15) developed for heritable mutations from genotoxic compounds. Representing both transgenerational and multigenerational effects in AOPs can be more complex due to the need to consider interactions between generations and the potential for cumulative effects, including selection for/against these effects. Additionally, the empirical evidence supporting such effects is still evolving, and this could impact the level of detail and certainty in the AOP representation. The issue of how to account for heritable effects in radiation risk assessments has been discussed in detail by UNSCEAR (Citation2010).
Overall, this section highlights that understanding complex processes and phenomena is an important topic for radiation protection. In many cases, the AOP framework can support these areas; however, as most topics are still developing, consistent evidence and understanding of the critical mechanistic aspects need to evolve further.
Individualized risk assessment
Currently, the estimates of adverse health risks for the stochastic effects of radiation (cancers and heritable effects) that inform judgements, particularly on regulatory dose limits, are nominal (averaged over age, sex and population) (Ban et al. Citation2022). In parallel, there is increasing interest in the use of more individualized or stratified approaches, particularly in medicine, that can provide more accurate risk information for individuals. ICRP in its Publication 147 provided a method for cancer risk estimation that varies by age and sex (ICRP Citation2021), based on the data from the last general recommendations (ICRP Citation2007). This change has occurred over a relatively short time frame because of two key factors: (a) dose measurement has become both more accurate and rapid, and the use of artificial intelligence and deep learning to improve dose estimation is increasingly more common; (b) patients and their physicians have always desired individualized care, however, this was somewhat limited by a lack of mature science on the radiation biology, genetics, and pharmacology which are beginning to provide more stratified or individualized care.
This interest in a more individualized approach to imaging and therapeutic care is shared by the patient/family, the medical community, researchers, and the policy makers to decide how best to prioritize resources. The ‘individualized risk assessment approach’ includes a stratified one where some patients may be tested for their tumor radiosensitivity prior to treatment and then cared for in a treatment protocol based on these results. Only a limited number of cancers currently provide this approach. It is therefore of interest to consider how AOP approaches could be useful in this context. Although it is recognized that the current state of experimental knowledge limits application of AOPs for individualized hazard/risk assessment purposes, two stimulating questions from the HSE () relate to how AOPs could support strategies for individual dose estimation, avoidance, and understanding key factors in radiation sensitivity.
The AOP framework indeed may offer valuable insights into how stratified/individual dose estimation may be improved. By identifying essential biological events and their progression toward AOs, AOPs can inform on relevant biomarkers at the organism-level. Robust, sensitive, and accurate biomarkers may then enable development of tools, similar to cytogenetic assays that can help derive dose-estimates (biodosimetry) or point of departure that can be used to understand radiation sensitivities. Furthermore, since AOPs identify multiple endpoints for each critical KE, this information can be more informative than a single biomarker. For instance, when examining oxidative stress, an essential KE to radiation-injury, multiple aspects such as radical formation, radical generating enzymes, radical removing enzymes, and macromolecular damage can be considered in the assessment. In terms of radiation sensitivity, the doses at which KEs are triggered will be particularly important to inform on individual sensitivity, although this is envisioned as a long-term goal as it is recognized to come with several challenges related to accurate and consistent dose estimation.
AOP communication, acceptance, and future directions
Several prioritized questions (6 in total) from the HSE () related to how knowledge transfer and communication on AOPs will be critical to their acceptance. A recent article published in Nature has suggested that to speed scientific progress, researchers, regulators and policy makers must learn to communicate to understand each other (Clancy et al. Citation2023). One recommendation was ‘…to encourage more science-policy research that seeks fundamental understanding of problems while having immediate use for society…’. Engaging stakeholders and collaborators during AOP development will be critical for acceptance and use of AOPs for radiation hazard/risk assessments. Indeed, international organizations with a role in radiation protection (e.g., ICRP, National Council on Radiation Protection and Measurements (NCRP), UNSCEAR, OECD/NEA) have suggested AOPs as one potential tool to integrate population-based studies with molecular level data (NCRP Citation2020; Laurier et al. Citation2021). Additionally, as more radiation AOPs progress in various stages of development, funding agencies may recognize their value and adopt them as a necessary aspect for building sound proposals. To achieve this, active promotion and communication on the potential value of AOPs will be needed (Chauhan, Hamada et al. Citation2022) by informing funding agencies and bringing attention to the ongoing discussions and advancements from the chemical field and the work underway in the radiation field. Endorsement of an AOP by an international agency such as the NEA would greatly enhance interest in the potential added value of an AOP for knowledge consolidation and directing future research.
To advance some of these areas, the scientific, policy and regulatory communities will need to promote AOP development and reporting of AOPs in a format that is applicable for dissemination and implementation into a research framework. Scientific journals can also broadly support AOP development, by providing a platform to help with author submission and the review of AOPs such as with the development of a standard checklist (Chauhan, Stricklin et al. Citation2021; Chauhan, Villeneuve et al. Citation2021) alongside the dissemination and publication of the AOP reports. This would expedite AOP review and provide broader dissemination of the information.
Future directions should focus on integrating AOPs within other approaches to help derive quantitative risk estimates. These approaches may include the use of KERs (e.g., as dose-response functions) into BBDR models and tools used in the toxicity field, such as benchmark dose (BMD) modeling, regression modeling and BNs that have shown utility both for chemical and non-chemical stressors (Moe et al. Citation2021; Bajard et al. Citation2023; Cao et al. Citation2023; Chauhan et al. Citation2023).
BBDR models have thus far largely been developed for cancer incidence but have been shown to have application to other health outcomes (Kaiser et al. Citation2021). In future research, the relation between AOPs and BBDR models should be mapped out in greater detail. At least, AOPs can guide the broad conceptual design of BBDR models; for example, by suggesting sub-models for the most relevant disease pathways which might be driven by different stressors or by differential radiosensitivity. With more ambition, mechanistic information in AOPs could be applied as parameters for KEs and/or as dose response functions for KERs to improve the biological plausibility of conventional risk estimates (NCRP Citation2020).
Insight from AOPs on oncogenic mechanisms may confirm whether low dose radiation causes additional cancer cases (e.g., via initiating driver mutations) or shortens the latency time to cancer by accelerating the growth of preneoplastic lesions (e.g., via reduced clearing of precancerous cells caused by inflammation). For retrospective risk assessment, Greenland (Citation1999) discussed the need to distinguish between initiating and promoting radiation action. Eidemüller et al. (Citation2023) quantified Greenland’s conjecture with novel risk measures in a simulation study based on BBDR models for radiation-induced breast cancer. Ultimately, the biological plausibility of the dose responses applied in the BBDR models should be substantiated by trusted KERs originating from adequate AOPs.
BNs can be used to represent AOPs by modeling the relationships between MIE, KEs, and specifically infer the probability of an AO occurring on the basis of conditional dependencies between events. Additionally, a BN may support estimating the likelihood of an AOP and identify the KEs, modifiers and AOs within the pathway (Xie et al. Citation2018; Song et al. Citation2020; Cao et al. Citation2023). Approaches such as BMD modeling could also be used to support quantitative risk predictions from early macromolecular events in an AOP (Yu et al. Citation2022; Chauhan et al. Citation2023). Although the workflows of these approaches are well established using chemical datasets, there remains a need to understand the applicability using radiation biology and epidemiology datasets, and confirming the best correlative endpoints for deriving meaningful outcomes.
Conclusion
The HSE provided a significant number of priority questions. Most of the pertinent questions, which there are twenty-five, can be addressed within the AOP framework. A few of the questions were deemed out of scope to support an understanding of the biology to a pre-defined outcome of interest, anchored to an MIE. The out-of-scope questions where data is currently limited (e.g., protective effects, transgenerational effects) to support aspects of the Bradford-Hill criteria were seen as an area for advancement.
In an environment of increasing complexity, the AOP knowledge base can become a practical tool for better predicting effects on both human health and the environment, potentially better understanding of causality, reduction of uncertainty and improved protection. Currently there are no clear examples of how AOPs have been applied for regulatory purposes in the radiation protection field. This is partly due to a consequence of relatively limited mechanistic data to support a quantitative understanding for an AO and the infancy of application of AOPs to radiation. With enhanced knowledge, the AOP approach aims to integrate experimental radiobiological studies with AOs based on epidemiological and clinical data to inform safety assessment decisions. relevant to human and environmental health. The implementation of an AOP framework with its transparent and stringent reviews provides an accessible and reliable source of information that is designed to be continually updated as science progresses.
It is evident that the AOP framework is not to be used in isolation; it is a tool to be used in combination with other approaches, particularly taking into consideration exposure metrics (quality, intensity etc.) to develop quantitative relationships between events and identify points of departure (e.g., the smallest dose at which a pre-defined change occurs from the control) of KERs and regulatory needs and protective goals. The current work that is progressing through the JTG in relation to outcomes from case studies (Chauhan, Villeneuve et al. Citation2021; Azimzadeh et al. Citation2022; Jaylet et al. Citation2022; Klokov et al. Citation2022; Tollefsen et al. Citation2022) is expected to provide some valuable insights for the future development, application, and implication of AOPs in research and regulatory approaches.
As AOP development gains momentum in the radiation research and policy communities, several challenges are expected to arise, especially as case studies are identifying constraints to larger scale implementation in research and regulations. Resolving some of these issues may specifically involve focal areas as described in . By concentrating on these areas and fostering interdisciplinary collaboration, the radiation field can advance the development and application of AOPs, enabling more accurate hazard/risk assessments, informed decision-making, and enhanced protection against radiation-induced adverse health outcomes for humans and non-human species.
Table 1. Focus areas for future advancements of AOPs.
Acknowledgments
The authors wish to acknowledge the assistance of Dr. Ruth Wilkins and Dr. Katya Feder for critical review of the manuscript.
Disclosure statement
The authors report there are no competing interests to declare. In addition, it is noted that the opinions expressed here are those of the authors and do not necessarily reflect those of their respective organizations.
Correction Statement
This article has been corrected with minor changes. These changes do not impact the academic content of the article.
Additional information
Funding
Notes on contributors
Vinita Chauhan
Vinita Chauhan, Ph.D., is a Senior Research Scientist at the Consumer and Clinical Radiation Protection Bureau of Health Canada. She is a Canadian delegate of the Nuclear Energy Agency High-Level Group on Low Dose Research (HLG-LDR) and Emerging Science in Chemical Risk Assessment Working Party of the OECD. She also co-chairs the OECD/NEA/HLG-LDR Rad/Chem AOP Joint Topical Group.
Danielle Beaton
Danielle Beaton, Ph.D., is a Research Scientist. Her current research focuses on the effects of low dose radiation on biological systems. She is on the NEA HLG-LDR committee and assists the co-chairs of the HLG-LDR Rad/Chem AOP Joint Topical Group.
Knut Erik Tollefsen
Knut Erik Tollefsen, Ph.D., is a Chief Scientist at the Norwegian Institute for Water Research (NIVA), an Adjunct professor at the Norwegian University of Life Sciences (NMBU) and is a principal investigator and research area co-lead in the Center for Environmental Radioactivity (CERAD CoE). He is also the Norwegian delegate of the HLG-LDR and OECD EAGMST, co-chairs the HLG-LDR Rad/Chem AOP Joint Topical Group, co-ordinates NIVA’s Computational Toxicology Program, NCTP (www.niva.no/nctp) and is a registered OECD AOP coach.
Julian Preston
Julian Preston, Ph.D., has a Special Government Employee (Expert, Radiation Effects) appointment at the U.S. Environmental Protection Agency (EPA). He previously served as the Associate Director for Health (2007-2012) and the Director of the Environmental Carcinogenesis Division at EPA. Dr. Preston’s research and current activities have focused on how knowledge of the mechanisms of induction of radiation-induced adverse health effects can be used in cancer risk assessments. He has served in various capacities with NCRP, ICRP (including Chair, Committee 1, and Radiation Effects) and UNSCEAR (Representative and Head of Delegation). Dr Preston was the 2002 Lauriston S. Taylor Lecturer awarded by the NCRP and is a Fellow of the UK’s Royal Society for Radiation Protection. Dr. Preston has published over 250 peer-reviewed papers. He has served on National Academies’ committees including in 2021 as Vice Chair of the committee “Space Radiation and Astronaut Health: Managing and Communicating Cancer Risks”. He also serves on an OECD/NEA committee addressing the use of Adverse Outcome Pathways in cancer risk assessment at low doses and dose-rates. He received his B.A. and M.A. from Peterhouse, Cambridge University, England, in genetics and his Ph.D. from Reading University, England, in radiation genetics.
Julie J. Burtt
Julie J. Burtt, MSc., is a radiation biologist with the Canadian Nuclear Safety Commission. Her research focuses on the potential health effects of exposure to low doses of ionizing radiation. She has experience as an advisor for Canada to the United Nations Scientific Committee on the Effects of Atomic Radiation (UNSCEAR) as well as a technical expert for the UNSCEAR Secretariat. She also serves as vice-chair of the National Energy Agency’s Expert Group on Non-radiological Public Health Aspects of Radiation Emergency Planning and Response. She is also a member of Committee 4 for the International Commission on Radiological Protection.
Julie Leblanc
Julie Leblanc, Ph.D., is a radiation biologist with the Canadian Nuclear Safety Commission. Her research focuses on the potential health effects of exposure to low doses of ionizing radiation. She is a Canadian Delegate on the High-Level Group on Low Dose Research and International Commission on Radiological Protection mentee and member of Task Group 111.
Nobuyuki Hamada
Nobuyuki Hamada, RT, Ph.D., is a Senior Research Scientist at CRIEPI and a Visiting Professor at Hiroshima University Research Institute for Radiation Biology and Medicine and at Hokkaido University Graduate School of Health Sciences. He serves on ICRP Task Groups 111, 119 and 123, the UNSCEAR Expert Group on Effects of Ionizing Radiation on the Nervous System, NCRP PAC 1, OECD/NEA/CRPPH/HLG-LDR/Rad/Chem AOP Joint Topical Group, IRPA Task Group on Tissue Reactions, and Consultation Committee on AOP development for space flight health outcomes (Canadian project).
Edouard I. Azzam
Edouard Azzam is a Radiation Biologist at Canadian Nuclear Laboratories. He is also Professor Emeritus at Rutgers New Jersey Medical School and a member of the US National Council on Radiation Protection and Measurements. His research over the past three decades has focused on the role of intercellular communication and oxidative metabolism in expression of adaptive responses and bystander effects induced by low doses/low fluences of ionizing radiation from environmental, occupational, or medical sources.
Olivier Armant
Olivier Armant, Ph.D, is a senior scientist at the French Institute of Radioprotection and Nuclear Safety (IRSN). His studies focus on the molecular mechanisms deregulated upon exposure to low dose of radiations during embryonic development and post-accidental effects on wild life in Chernobyl and Fukushima. He is a member of the High-Level Group on Low-Dose Research (HLG-LDR) Rad/Chem Joint Topical Group.
Simon Bouffler
Simon Bouffler is the deputy director of the Radiation Protection Science at the UK Health Security Agency. He was trained as a biologist, receiving a BSc and Ph.D. from the University of Southampton. He is a member of the main commission of ICRP and the UK representative to UNSCEAR.
Omid Azimzadeh
Omid Azimzadeh, Ph.D, PD, is a senior researcher in the section of Radiation Biology at the German Federal Office for Radiation Protection (BfS) and an Adjunct Professor (Docent) of Radiation Biology at Technical University Munich. He is a member of the working group CircuDis (Circulatory Diseases from Radiation Exposure) at the United Nations Scientific Committee on the Effects of Atomic Radiation (UNSCEAR) and the High-Level Group on Low Dose Research (HLG-LDR) Rad/Chem AOP Joint Topical Group.
Simone Moertl
Simone Moertl, Ph.D., PD, is head of the section of Radiation Biology at the Federal Office for Radiation Protection (BfS) and an Adjunct Professor (Docent) of Radiation Biology at Technical University Munich. Until September 2019 she led the group of Clinical Radiobiology at the Institute of Radiation Biology, Helmholtz Zentrum München. Her research focuses on the cellular radiation response of tumors and normal tissues with the aim to understand the mechanisms of individual radiation sensitivity. She is particularly interested in the vesicle-mediated transfer (e.g., by exosomes) of RNAs between cells. She is a member of the HLG-LDR Rad/Chem AOP Joint Topical Group.
Yutaka Yamada
Yutaka Yamada DVM, Ph.D., is a research scientist of the Department of Radiation Effects Research, Institute for Radiological Science, QST, Japan. His main field is radiation biology and radiotoxicology, and his studies have focused on radiation-induced pulmonary carcinogenesis.
Ignacia B. Tanaka
Ignacia Braga-Tanaka, DVM, Ph.D., a trained veterinarian with a PhD in Comparative Pathology (and an M.S. in Ruminant Nutrition) and is currently a senior researcher in the Department of Radiobiology at the Institute for Environmental Sciences. She investigates the late effects (neoplastic and non-neoplastic disease) of chronic low dose-rate radiation exposure on mice.
Jan Christian Kaiser
Jan Christian Kaiser, PhD, is a scientific officer with the German Federal Office of Radiation Protection. His research is concerned with the development of biologically based risk models for radiation-induced cancer and cardiovascular disease. Currently, he is the lead writer of the epidemiology section for the upcoming UNSCEAR scientific annex “Evaluation of diseases of the circulatory system from radiation exposure” (CircuDis).
Kimberly Applegate
Kimberly Applegate, MD, Ph.D is the chair of Committee 3 of the ICRP, focusing on radiation protection in medicine and a member of the National Council for Radiation Protection and Measurements (NCRP). Dr. Applegate is a retired professor of radiology and pediatrics from the University of Kentucky in Lexington. Dr. Applegate’s policy and research work, including 200 publications, has contributed to an improved understanding of the structure, process, and outcomes of how imaging, especially pediatrics, is practiced, including the volume and variation of imaging practice, toward its improvement and standardization. She has worked collaboratively around the world across medical specialties and geographic boundaries to improve access to best practices. From its start in 2007 to the present, she has worked on the Steering Committee for the Image Gently Campaign to improve children’s care worldwide. She has received several awards that include the 2019 AAPM’s Honorary Membership and the American Association for Women in Radiology’s Marie Sklowdoska Curie Award for her unique roles in leadership and outstanding contributions to the advancement of women in the Radiology professions.
Dominique Laurier
Dominique Laurier, Ph.D., is the Deputy Head of the Health Division at the French Institute of Radioprotection and Nuclear Safety (IRSN). He is the Chair of the International Commission on Radiological Protection (ICRP) Committee 1. He is also a member of the French delegation to the United Nations Scientific Committee on the Effects of Atomic Radiation (UNSCEAR), and the Chair of the Nuclear Energy Agency (NEA) High-Level Group on Low Dose Research (HLG-LDR).
Jacqueline Garnier-Laplace
Jacqueline Garnier-Laplace, Ph.D, a Senior Radiation Protection Specialist, is on secondment from the French Institute of Radiation Protection and Nuclear Safety (IRSN) to the Scientific Secretary of the Committee on Radiological Protection and Public Health, and of the NEA HLG-LDR. Previously, she headed Radiation Protection research at IRSN. She served as scientific secretary of ICRP Committee 1 from 2017 to 2021 and is currently serving on Committee 4 with the same function..
References
- Ankley GT, Bennett RS, Erickson RJ, Hoff DJ, Hornung MW, Johnson RD, Mount DR, Nichols JW, Russom CL, Schmieder PK, et al. 2010. Adverse outcome pathways: A conceptual framework to support ecotoxicology research and risk assessment. Environ Toxicol Chem. 29(3):730–741. doi:10.1002/etc.34
- Azimzadeh O, Moertl S, Ramadan R, Baselet B, Laiakis EC, Sebastian S, Beaton D, Hartikainen JM, Kaiser JC, Beheshti A, et al. 2022. Application of radiation omics in the development of adverse outcome pathway networks: an example of radiation-induced cardiovascular disease. Int J Radiat Biol. 98(12):1722–1751. doi:10.1080/09553002.2022.2110325
- Azzam EI, Jay-Gerin JP, Pain D. 2012. Ionizing radiation-induced metabolic oxidative stress and prolonged cell injury. Cancer Lett. 327(1-2):48–60. doi:10.1016/j.canlet.2011.12.012
- Bajard L, Adamovsky O, Audouze K, Baken K, Barouki R, Beltman JB, Beronius A, Bonefeld-Jørgensen EC, Cano-Sancho G, de Baat ML, et al. 2023. Application of AOPs to assist regulatory assessment of chemical risks - Case studies, needs and recommendations. Environ Res. 217:114650., doi:10.1016/j.envres.2022.114650
- Ban N, Cléro E, Vaillant L, Zhang W, Hamada N, Preston D, Laurier D. 2022. Radiation detriment calculation methodology: summary of ICRP Publication 152. J Radiol Prot. 42(2), doi:10.1088/1361-6498/ac670d
- Becker RA, Ankley GT, Edwards SW, Kennedy SW, Linkov I, Meek B, Sachana M, Segner H, Van Der Burg B, Villeneuve DL, et al. 2015. Increasing scientific confidencein adverse outcome pathways: Application of tailored Bradford-Hill considerations for evaluating weight of evidence. Regul Toxicol Pharmacol. 72(3):514–537. doi:10.1016/j.yrtph.2015.04.004
- Beyer J, Petersen K, Song Y, Ruus A, Grung M, Bakke T, Tollefsen KE. 2014. Environmental risk assessment of combined effects in aquatic ecotoxicology: a discussion paper. Mar Environ Res. 96:81–91. doi:10.1016/j.marenvres.2013.10.008
- Burtt JJ, Leblanc J, Randhawa K, Ivanova A, Rudd MA, Wilkins R, Azzam EI, Hecker M, Horemans N, Vandenhove H, et al. 2022. Radiation adverse outcome pathways (AOPs) are on the horizon: advancing radiation protection through an international Horizon-Style exercise. Int J Radiat Biol. 98(12):1763–1776. doi:10.1080/09553002.2022.2121439
- Cao Y, Moe SJ, De Bin R, Tollefsen KE, Song Y. 2023. Comparison of piecewise structural equation modeling and Bayesian network for de novo construction of a quantitative adverse outcome pathway network. ALTEX. 40(2):287–298.
- Carrothers E, Appleby M, Lai V, Kozbenko T, Alomar D, Smith BJ, Hamada N, Hinton P, Ainsbury EA, Hocking R, et al. 2024. AOP report: Development of an adverse outcome pathway for deposition of energy leading to cataracts. Environ Mol Mutagen. doi:10.1002/em.22594. Epub ahead of print.
- Chauhan V, Said Z, Daka J, Sadi B, Bijlani D, Marchetti F, Beaton D, Gaw A, Li C, Burtt J, et al. 2019. Is there a role for the adverse outcome pathway framework to support radiation protection? Int J Radiat Biol. 95(2):225–232. doi:10.1080/09553002.2019.1532617
- Chauhan V, Hamada N, Monceau V, Ebrahimian T, Adam N, Wilkins RC, Sebastian S, Patel ZS, Huff JL, Simonetto C, et al. 2021. Expert consultation is vital for adverse outcome pathway development: a case example of cardiovascular effects of ionizing radiation. Int J Radiat Biol. 97(11):1516–1525. doi:10.1080/09553002.2021.1969466
- Chauhan V, Sherman S, Said Z, Yauk CL, Stainforth R. 2021. A case example of a radiation-relevant adverse outcome pathway to lung cancer. Int J Radiat Biol. 97(1):68–84. doi:10.1080/09553002.2019.1704913
- Chauhan V, Stricklin D, Cool D. 2021. The integration of the adverse outcome pathway framework to radiation risk assessment. Int J Radiat Biol. 97(1):60–67. doi:10.1080/09553002.2020.1761570
- Chauhan V, Villeneuve D, Cool D. 2021. Collaborative efforts are needed among the scientific community to advance the adverse outcome pathway concept in areas of radiation risk assessment. Int J Radiat Biol. 97(6):815–823. doi:10.1080/09553002.2020.1857456
- Chauhan V, Wilkins RC, Beaton D, Sachana M, Delrue N, Yauk C, O'Brien J, Marchetti F, Halappanavar S, Boyd M, et al. 2021. Bringing together scientific disciplines for collaborative undertakings: a vision for advancing the adverse outcome pathway framework. Int J Radiat Biol. 97(4):431–441. doi:10.1080/09553002.2021.1884314
- Chauhan V, Beaton D, Hamada N, Wilkins R, Burtt J, Leblanc J, Cool D, Garnier-Laplace J, Laurier D, Le Y, et al. 2022. Adverse outcome pathway: a path toward better data consolidation and global co-ordination of radiation research. Int J Radiat Biol. 98(12):1694–1703. doi:10.1080/09553002.2021.2020363
- Chauhan V, Hamada N, Garnier-Laplace J, Laurier D, Beaton D, Tollefsen KE, Locke PA. 2022. Establishing a communication and engagement strategy to facilitate the adoption of the adverse outcome pathways in radiation research and regulation. Int J Radiat Biol. 98(12):1714–1721. doi:10.1080/09553002.2022.2086716
- Chauhan V, Yu J, Vuong N, Haber LT, Williams A, Auerbach SS, Beaton D, Wang Y, Stainforth R, Wilkins RC, et al. 2023. Considerations for application of benchmark dose modeling in radiation research: workshop highlights. Int J Radiat Biol. 99(9):1320–1331. doi:10.1080/09553002.2023.2181998
- Clancy M, Correa D, Dworkin J, Niehaus P, Watney C, Williams H. 2023. Want to speed up scientific progress? First understand how science policy works. Nature. 620(7975):724–726. doi:10.1038/d41586-023-02602-9
- Cool DA, Kase KR, Boice JD. 2019. NCRP Report No.180-management of exposure to ionizing radiation: NCRP radiation protection guidance for the United States. J Radiol Prot. 39(3):966–977. doi:10.1088/1361-6498/ab1826
- Eidemüller M, Becker J, Kaiser JC, Ulanowski A, Apostoaei AI, Hoffman FO. 2023. Concepts of association between cancer and ionising radiation: accounting for specific biological mechanisms. Radiat Environ Biophys. 62(1):1–15. doi:10.1007/s00411-022-01012-1
- Flanders WD. 2006. On the relationship of sufficient component cause models with potential outcome (counterfactual) models. Eur J Epidemiol. 21:847–853. doi:10.1007/s10654-006-9048-3
- Greenland S. 1999. Relation of probability of causation to relative risk and doubling dose: a methodologic error that has become a social problem. Am J Public Health. 89(8):1166–1169. doi:10.2105/ajph.89.8.1166
- Guéguen Y, Frerejacques M. 2022. Review of knowledge of uranium-induced kidney toxicity for the development of an adverse outcome pathway to renal impairment. Int J Mol Sci. 23(8):4397. Apr 15 doi:10.3390/ijms23084397
- Hamada N, Zablotska LB. 2023. New evidence for brain cancer risk after a single paediatric CT scan. Lancet Oncol. 24(1):2–3. Jan doi:10.1016/S1470-2045(22)00696-9
- Hamada N. 2023. Noncancer effects of ionizing radiation exposure on the eye, the circulatory system and beyond: developments made since the 2011 ICRP statement on tissue reactions. Radiat Res. 200(2):188–216.
- Hamada N, Maeda M, Otsuka K, Tomita M. 2011. Signaling pathways underpinning the manifestations of ionizing radiation-induced bystander effects. Curr Mol Pharmacol. 4(2):79–95. doi:10.2174/1874467211104020079
- Hauptmann M, Daniels RD, Cardis E, Cullings HM, Kendall G, Laurier D, Linet MS, Little MP, Lubin JH, Preston DL, et al. 2020. Epidemiological studies of low-dose ionizing radiation and cancer: Summary bias assessment and meta-analysis. J Natl Cancer Inst Monogr. 2020(56):188–200. Jul 1 Erratum in: J Natl Cancer Inst Monogr. 2023 May 4;2023(61):e1 doi:10.1093/jncimonographs/lgaa010
- Hauptmann M, Byrnes G, Cardis E, Bernier MO, Blettner M, Dabin J, Engels H, Istad TS, Johansen C, Kaijser M, et al. 2023. Brain cancer after radiation exposure from CT examinations of children and young adults: results from the EPI-CT cohort study. Lancet Oncol. 24(1):45–53. Jan doi:10.1016/S1470-2045(22)00655-6
- ICRP. 2007. The 2007 recommendations of the International Commission on Radiological Protection. Oxford: Pergamon Press.
- ICRP. 2012. ICRP Statement on Tissue Reactions/Early and Late Effects of Radiation in Normal Tissues and Organs – Threshold Doses for Tissue Reactions in a Radiation Protection Context. ICRP Publication 118. Ann. ICRP 41(1/2).
- ICRP. 2021. Use of dose quantities in radiological protection. ICRP Publication 147. Ann. ICRP. 50(1).
- Jaylet T, Quintens R, Benotmane MA, Luukkonen J, Tanaka IB, 3rd, Ibanez C, Durand C, Sachana M, Azimzadeh O, Adam-Guillermin C, et al. 2022. Development of an Adverse Outcome Pathway for radiation-induced microcephaly via expert consultation and machine learning. Int J Radiat Biol. 98(12):1752–1762. doi:10.1080/09553002.2022.2110312
- Jaylet T, Quintens R, Armant O, Audouze K. 2023. An integrative systems biology strategy to support the development of adverse outcome pathways (AOPs): a case study on radiation-induced microcephaly. Front Cell Dev Biol. 11:1197204. Jun 22 doi:10.3389/fcell.2023.1197204
- Jornod F, Jaylet T, Blaha L, Sarigiannis D, Tamisier L, Audouze K. 2022. AOP-helpFinder webserver: a tool for comprehensive analysis of the literature to support adverse outcome pathways development. Bioinformatics. 38(4):1173–1175. doi:10.1093/bioinformatics/btab750
- Kaiser JC, Blettner M, Stathopoulos GT. 2021. Biologically based models of cancer risk in radiation research. Int J Radiat Biol. 97(1):2–11. doi:10.1080/09553002.2020.1784490
- Klokov D, Applegate K, Badie C, Brede DA, Dekkers F, Karabulutoglu M, Le Y, Rutten EA, Lumniczky K, Gomolka M. 2022. International expert group collaboration for developing an adverse outcome pathway to radiation induced leukaemia. Int J Radiat Biol. 98(12):1802–1815. doi:10.1080/09553002.2022.2117873
- Kozbenko T, Adam N, Lai V, Sandhu S, Kuan J, Flores D, Appleby M, Parker H, Hocking R, Tsaioun K, et al. 2022. Deploying elements of scoping review methods for Adverse Outcome Pathway development: A space travel case example. Int J Radiat Biol. 98(12):1777–1788. doi:10.1080/09553002.2022.2110306
- Krewski D, Acosta D, Andersen M, Anderson H, Bailar JC, Boekelheide K, Brent R, Charnley G, Cheung VG, Green S, et al. 2010. Toxicity testing in the 21st century: a vision and a strategy. J Toxicol Environ Health B Crit Rev. 13(2-4):51–138. doi:10.1080/10937404.2010.483176
- Laurier D, Billarand Y, Klokov D, Leuraud K. 2023. The scientific basis for the use of the linear no-threshold (LNT) model at low doses and dose rates in radiological protection. J Radiol Prot. 43(2). doi:10.1088/1361-6498/acdfd7
- Laurier D, Rühm W, Paquet F, Applegate K, Cool D, Clement C, International Commission on Radiological Protection (ICRP). 2021. Areas of research to support the system of radiological protection. Radiat Environ Biophys. 60(4):519–530. doi:10.1007/s00411-021-00947-1
- Leist M, Ghallab A, Graepel R, Marchan R, Hassan R, Bennekou SH, Limonciel A, Vinken M, Schildknecht S, Waldmann T, et al. 2017. Adverse outcome pathways: opportunities, limitations and open questions. Arch Toxicol. 91(11):3477–3505. doi:10.1007/s00204-017-2045-3
- Little MP, Wakeford R, Borrego D, French B, Zablotska LB, Adams MJ, Allodji R, de Vathaire F, Lee C, Brenner AV, et al. 2018. Leukaemia and myeloid malignancy among people exposed to low doses (<100 mSv) of ionising radiation during childhood: a pooled analysis of nine historical cohort studies. Lancet Haematol. 5(8):e346–e358. Augdoi:10.1016/S2352-3026(18)30092-9
- Little MP, Cahoon EK, Kitahara CM, Simon SL, Hamada N, Linet MS. 2020. Occupational radiation exposure and excess additive risk of cataract incidence in a cohort of US radiologic technologists. Occup Environ Med. 77(1):1–8. doi:10.1136/oemed-2019-105902
- Little MP, Azizova TV, Richardson DB, Tapio S, Bernier MO, Kreuzer M, Cucinotta FA, Bazyka D, Chumak V, Ivanov VK, et al. 2023. Ionising radiation and cardiovascular disease: systematic review and meta-analysis. BMJ. 380:e072924. doi:10.1136/bmj-2022-072924
- Little MP, Wakeford R, Bouffler SD, Abalo K, Hauptmann M, Hamada N, Kendall GM. 2022. Review of the risk of cancer following low and moderate doses of sparsely ionising radiation received in early life in groups with individually estimated doses. Environ Int. 159:106983. doi:10.1016/j.envint.2021.106983
- Matsumoto H, Tomita M, Otsuka K, Hatashita M, Hamada N. 2011. Nitric oxide is a key molecule serving as a bridge between radiation-induced bystander and adaptive responses. Curr Mol Pharmacol. 4(2):126–134. doi:10.2174/1874467211104020126
- Moe SJ, Wolf R, Xie L, Landis WG, Kotamäki N, Tollefsen KE. 2021. Quantification of an adverse outcome pathway network by Bayesian regression and Bayesian network modeling. Integr Environ Assess Manag. 17(1):147–164. doi:10.1002/ieam.4348
- NCRP. 2018. Implications of Recent Epidemiologic Studies for the Linear-Nonthreshold Model and Radiation Protection. NCRP Commentary No. 27, National Council on Radiation Protection and Measurements, 2018.
- NCRP. 2020. Approaches for integrating information from radiation biology and epidemiology to enhance low-dose health risk assessment. NCRP Report No. 186, National Council on Radiation Protection and Measurements, 2020.
- NRC. 2007. Toxicity Testing in the 21st Century: A Vision and a Strategy. Washington, DC: The National Academies Press. doi:10.17226/11970
- OECD. 2018. Users’ Handbook supplement to the Guidance Document for developing and assessing Adverse Outcome Pathways. OECD Series on Adverse Outcome Pathways, No. 1, Organisation for Economic Co-operation and Development Publishing, Paris, France.
- OECD. 2021. Guidance document for the scientific review of adverse outcome pathways. Series on Testing and Assessment, No. 344. Series on Adverse Outcome Pathways, No. 20. ENV/CBC/MONO. 22, Organisation of Economic Co-operation and Development, Paris, France.
- Preston RJ. 2017. Can radiation research impact the estimation of risk? Int J Radiat Biol. Oct93(10):1009–1014. doi:10.1080/09553002.2017.1290848
- Richardson DB, Leuraud K, Laurier D, Gillies M, Haylock R, Kelly-Reif K, Bertke S, Daniels RD, Thierry-Chef I, Moissonnier M, et al. 2023. Cancer mortality after low dose exposure to ionising radiation in workers in France, the United Kingdom, and the United States (INWORKS): cohort study. BMJ. 382:e074520. doi:10.1136/bmj-2022-074520
- Rothman KJ, Greenland S. 2005. Causation and causal inference in epidemiology. Am J Public Health. 95 Suppl 1:S144–S50. doi:10.2105/AJPH.2004.059204
- Rühm W, Woloschak GE, Shore RE, Azizova TV, Grosche B, Niwa O, Akiba S, Ono T, Suzuki K, Iwasaki T, et al. 2015. Dose and dose-rate effects of ionizing radiation: a discussion in the light of radiological protection. Radiat Environ Biophys. 54(4):379–401. doi:10.1007/s00411-015-0613-6
- Rühm W, Laurier D, Wakeford R. 2022. Cancer risk following low doses of ionising radiation - Current epidemiological evidence and implications for radiological protection. Mutat Res Genet Toxicol Environ Mutagen. 873:503436. doi:10.1016/j.mrgentox.2021.503436
- Sabolić I. 2006. Common mechanisms in nephropathy induced by toxic metals. Nephron Exp. Nephrol. 104:107–114.
- Salbu B, Teien HC, Lind OC, Tollefsen KE. 2019. Why is the multiple stressor concept of relevance to radioecology? Int J Radiat Biol. 95(7):1015–1024. doi:10.1080/09553002.2019.1605463
- Seidle T, Stephens ML. 2009. Bringing toxicology into the 21st century: a global call to action. Toxicol in Vitro. 23(8):1576–1579. doi:10.1016/j.tiv.2009.06.012
- Sherman S, Said Z, Sadi B, Yauk C, Beaton D, Wilkins R, Stainforth R, Adam N, Chauhan V. 2023. Adverse Outcome Pathway on deposition of energy leading to lung cancer, OECD Series on Adverse Outcome Pathways, No. 32, OECD Publishing, Paris. doi:10.1787/a8f262c2-en
- Simonetto C, Kaiser JC, van den Bogaard VAB, Langendijk JA, Crijns APG. 2022. Breast Cancer Radiation Therapy and the Risk of Acute Coronary Events: Insights from a Process-Oriented Model. Int J Radiat Oncol Biol Phys. 114(3):409–415. doi:10.1016/j.ijrobp.2022.06.082
- Song Y, Xie L, Lee Y, Tollefsen KE. 2020. De novo development of a quantitative adverse outcome pathway (qAOP) network for ultraviolet B (UVB) radiation using targeted laboratory tests and automated data mining. Environ Sci Technol. 54(20):13147–13156. doi:10.1021/acs.est.0c03794
- Song Y, Kamstra JH, Cao Y, Asselman J, Anglès d‘Auriac M, Friberg N. 2021. High-throughput analyses and Bayesian network modeling highlight novel epigenetic Adverse Outcome Pathway networks of DNA methyltransferase inhibitor mediated transgenerational effects. J Hazard Mater. 408:124490. Apr 15 doi:10.1016/j.jhazmat.2020.124490
- Stainforth R, Schuemann J, McNamara AL, Wilkins RC, Chauhan V. 2021. Challenges in the quantification approach to a radiation relevant adverse outcome pathway for lung cancer. Int J Radiat Biol. 97(1):85–101. doi:10.1080/09553002.2020.1820096
- Svingen T, Villeneuve DL, Knapen D, Panagiotou EM, Draskau MK, Damdimopoulou P, O'Brien JM. 2021. A Pragmatic Approach to Adverse Outcome Pathway Development and Evaluation. Toxicol Sci. 184(2):183–190. doi:10.1093/toxsci/kfab113
- Tanabe S, Beaton D, Chauhan V, Choi I, Danielsen P, Delrue N, Esterhuizen M, Filipovska J, FitzGerald R, Fritsche E. 2022. Report of the 1st and 2nd mystery of reactive oxygen species conferences. ALTEX. 39(2):336–338. doi:10.14573/altex.2203011
- Tanabe S, O'Brien J, Tollefsen KE, Kim Y, Chauhan V, Yauk C, Huliganga E, Rudel RA, Kay JE, Helm JS, et al. 2022. Reactive oxygen species in the adverse outcome pathway framework: towards creation of harmonized consensus key events. Front Toxicol. 4:887135. doi:10.3389/ftox.2022.887135
- Thaulow J, Song Y, Lindeman LC, Kamstra JH, Lee Y, Xie L, Aleström P, Salbu B, Tollefsen KE. 2020. Epigenetic, transcriptional and phenotypic responses in Daphnia magna exposed to low-level ionizing radiation. Environ Res. 190:109930. doi:10.1016/j.envres.2020.109930
- Tollefsen KE, Alonzo FR, Beresford NA, Brede DA, Dufourcq-Sekatcheff E, Gilbin R, Horemans N, Hurem S, Laloi P, Maremonti E, et al. 2022. Adverse outcome pathways (AOPs) for radiation-induced reproductive effects in environmental species: state of science and identification of a consensus AOP network. Int J Radiat Biol. 98(12):1816–1831. doi:10.1080/09553002.2022.2110317
- UNSCEAR. 2010. Report of the United Nations Scientific Committee on the Effects of Atomic Radiation: 57th Session: Summary of Low_Dose Radiation Effects on Health. UNSCEAR; New York NY, USA: 2010.
- UNSCEAR. 2021. Annex C. Biological mechanisms relevant for the inference of cancer risks from low-dose and low-dose-rate radiation UNSCEAR 2020/2021 Report (Vienna: UNSCEAR).
- Xie L, Gomes T, Solhaug KA, Song Y, Tollefsen KE. 2018. Linking mode of action of the model respiratory and photosynthesis uncoupler 3,5-dichlorophenol to adverse outcomes in Lemna minor. Aquat Toxicol. 197:98–108. doi:10.1016/j.aquatox.2018.02.005
- Xie L, Song Y, Petersen K, Solhaug KA, Lind OC, Brede DA, Salbu B, Tollefsen KE. 2022. Ultraviolet B modulates gamma radiation-induced stress responses in Lemna minor at multiple levels of biological organisation. Sci Total Environ. 846:157457. doi:10.1016/j.scitotenv.2022.157457
- Yu J, Tu W, Payne A, Rudyk C, Cuadros Sanchez S, Khilji S, Kumarathasan P, Subedi S, Haley B, Wong A, et al. 2022. Adverse Outcome Pathways and Linkages to Transcriptomic Effects Relevant to Ionizing Radiation Injury. Int J Radiat Biol. 98(12):1789–1801. doi:10.1080/09553002.2022.2110313