Abstract
Although dysregulated stress biology is becoming increasingly recognized as a key driver of lifelong disparities in chronic disease, we presently have no validated biomarkers of toxic stress physiology; no biological, behavioral, or cognitive treatments specifically focused on normalizing toxic stress processes; and no agreed-upon guidelines for treating stress in the clinic or evaluating the efficacy of interventions that seek to reduce toxic stress and improve human functioning. We address these critical issues by (a) systematically describing key systems and mechanisms that are dysregulated by stress; (b) summarizing indicators, biomarkers, and instruments for assessing stress response systems; and (c) highlighting therapeutic approaches that can be used to normalize stress-related biopsychosocial functioning. We also present a novel multidisciplinary Stress Phenotyping Framework that can bring stress researchers and clinicians one step closer to realizing the goal of using precision medicine-based approaches to prevent and treat stress-associated health problems.
Introduction
The world is facing highly concerning increases in mental and physical illnesses (Dragioti et al., Citation2022; The Lancet, Citation2020), and a growing body of research points to stress biology as a key contributing factor (O’Connor et al., Citation2021). Indeed, prolonged, excessive, or repeated activation of the stress response, especially during childhood when the brain and immune system are still developing, has been called toxic stress (Bucci et al., Citation2016; National Academies of Sciences, Citation2019). Toxic stress is characterized by the prolonged or extreme activation of the stress response and persistent neurologic, endocrine, immune, metabolic and genetic regulatory disruptions that lead to poor health outcomes (Brown et al., Citation2009; Gilgoff et al., Citation2020; Hughes et al., Citation2017; McEwen, Citation2000b; Nelson et al., Citation2020; Shields & Slavich, Citation2017; Shonkoff et al., Citation2012; Slavich, Citation2016, Citation2020). Advancements in understanding the neural mechanisms of chronic conditions such as posttraumatic stress disorder (PTSD) are, in turn, paving the way for innovations in assessing and treating toxic stress (Bhushan et al., Citation2020; Kearney & Lanius, Citation2022; Lanius et al., Citation2015; Malejko et al., Citation2017; Manthey et al., Citation2021).
Experiences change our biology, which is evident from extensive research on the biology of stress (Slavich et al., Citation2023; Slavich & Cole, Citation2013). During this process, sensory inputs from the internal and external environment influence the activity of the central nervous system, and those identified as potentially threatening can lead to changes in physiologic systems that manifest as alterations in mood, behavior, and health. Indeed, multidisciplinary studies on stress, early life adversity (ELA), and trauma have demonstrated that stress is associated with dysregulation in sensorimotor, pain, vestibular, gut-brain axis, arousal and energy, reward processing, autonomic nervous system (ANS), hypothalamic-pituitary-adrenal (HPA) axis and endocrine, metabolic, immune, cognitive, and relational function (Burke et al., Citation2017; Duffy et al., Citation2018; Eisenberger & Cole, Citation2012; Lanius et al., Citation2015; Novick et al., Citation2018; Slavich, Citation2020; Teicher & Samson, Citation2016). Clinical tools and emerging research-based strategies are available for assessing stress-related dysregulations using self-report questionnaires, biomarkers, wearable devices, and brain mapping techniques (Reinertsen & Clifford, Citation2018; Shonkoff et al., Citation2022). These approaches can, in turn, be used in conjunction with instruments for assessing stressor exposure (Shields & Slavich, Citation2017; Slavich et al., Citation2019) as well as methods for assessing clinical symptoms.
In the present article, we describe the Stress Phenotyping Framework, a novel multidisciplinary conceptual framework centered around stress biology that can inform a translational cells-to-solutions approach to assessing and treating toxic stress, which has the potential to profoundly improve health outcomes. Whereas the stress literature is often simplified in its focus on behavioral (i.e., fight, flight, freeze, and fawn) or physiological (i.e., the HPA axis & ANS) responses to stress, human stress biology is much more complicated. Simplifying these complex stress response systems may thus be hampering progress in managing toxic stress. A broader systems-based stress assessment and treatment approach, in contrast, will improve predictive models and open the door to additional therapeutic targets, including more individualized treatment plans (Berens et al., Citation2017; Gilgoff et al., Citation2022; Lanius et al., Citation2015; Ortiz et al., Citation2022; Shonkoff et al., Citation2022).
To address these issues, this narrative review combines evidence and expertise from a broad variety of disciplines to (a) describe top-down and bottom-up mechanistic pathways through which stress impacts health, (b) discuss associated biological and behavioral biomarkers and assessment tools, and (c) highlight emerging and targeted evidence-based biological, psychological, and social therapeutic interventions, in turn providing a multi-disciplinary precision medicine approach that can be used in future translational research on the prevention and treatment of toxic stress (see and ). First, we define toxic stress and describe each of the stress-related systems involved, including their mechanistic pathways, potential targeted assessment, and therapeutic strategies. Second, we offer considerations for using the Stress Phenotyping Framework in clinical practice. Finally, we provide a call to action for researchers and clinicians describing the key advancements that must occur to move toward an effective process to diagnose and treat toxic stress. In covering these topics, our goal is to highlight assessment tools and therapeutic interventions that can be leveraged by researchers and clinicians from various disciplines that are impacted by stress biology. These disciplines include, but are not limited to, psychology, psychiatry, social work, public health, physical and occupational therapy, neuroscience, neurology, obstetrics and gynecology, primary care, cardiology, endocrinology, gastroenterology, and oncology.
Table 1. Stress Phenotyping Framework: Mechanistic pathways and health implications.
Table 2. Stress Phenotyping Framework: Assessments and targeted interventions.
Toxic stress and the Stress Phenotyping Framework
Toxic stress is the prolonged or extreme activation of the stress response that leads to dysregulation or impairment of at least one stress-related system (Bucci et al., Citation2016; National Academies of Sciences, Citation2019). Therefore, ELA, Adverse Childhood Experiences (ACEs), big and little “t” trauma in children and adults, and additional stressors such as discrimination, racism, and poverty may lead to toxic stress if they cause prolonged or extreme activation of the stress response and subsequent dysregulation in one or more stress-related systems. As depicted in , we present a novel multidisciplinary Stress Phenotyping Framework to allow for the integration of research and clinical expertise across disciplines, and the bridging of definitional or semantic differences.
Figure 1. The Stress Phenotyping Framework.
Ax, Example assessments; Tx, Example Treatments; GI, gastrointestinal; OT, occupational therapy; EMDR, Eye Movement Desensitization Reprocessing; LDN, low dose naltrexone; qEEG, quantitative electroencephalogram; CBT-I, Cognitive Behavioral Therapy for Insomnia; TMS, Transcranial Magnetic Stimulation; HR, heart rate; RR, respiratory rate; BP, blood pressure; HRV, heart rate variability; DHEA, dehydroepiandrosterone: HgbA1c, hemoglobin A1c; CBC with diff, complete blood count with differential; hsCRP, high-sensitivity c-reactive protein; EF, executive function; TF-CBT, Trauma-Focused Cognitive Behavioral Therapy; DBT, Dialectical Behavior Therapy
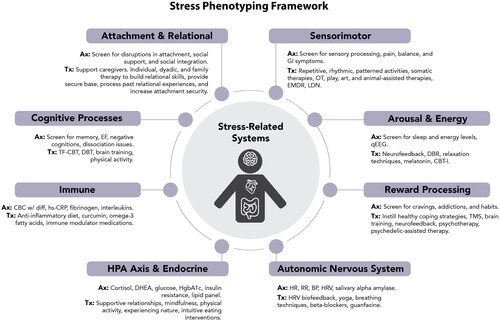
Using a framework that considers stress biology and stress-related systems enables clinicians to more clearly address both current stressors and internal stress physiology (Gilgoff et al., Citation2022). Such a framework also provides a structure to further test the effects of developmental timing, type, duration, severity, and perception of the adverse event(s), predictability, tolerance, and sensitization, environmental and biological protective factors, and predisposing vulnerabilities across a broader range of systems (see Box 1). Although understanding the specific features of external stressors is critical to better understanding mechanistic pathways, prevention strategies, and stressor-related interventions (Monroe & Slavich, Citation2016; Slavich, Citation2016, Citation2019), herein, we focus on how to assess and address internal toxic stress physiology—that is, the internal dysregulation of stress-related systems (vs. the stressors that induce such dysregulation).
Stress-related systems
Below we describe each of the systems impacted by toxic stress – sensorimotor, arousal and energy, reward processing, ANS, HPA axis and endocrine processes, immune, cognitive, and relational health – to lay the foundation for future research and a stress-biology approach to clinical interventions. Using this Stress Phenotyping Framework, a clinician could assess and identify which stress-related systems are affected for their client/patient and create a case conceptualization plan to provide tailored interventions addressing those specific stress-related systems.
Sensorimotor: Somatosensory and pain processing, vestibular balance, and gut-brain axis
The sensorimotor system has a bidirectional role in stress that carries warning signals from the environment to initiate the stress response, but also provides analgesia and alterations in mood in order to respond to the threat (Kearney & Lanius, Citation2022; Levine, Citation2015). Sensory information is transduced through cranial nerves to the lower and mid brain including the inferior and superior colliculi of the mesencephalon, thalamus, periaqueductal gray (PAG), and amygdala. Connectivity with the hippocampus and prefrontal cortex allows integration of past experience and contextual information to determine subsequent physiologic and behavioral responses: fight/flight, tonic immobility, emotional shut down, or pro-social behaviors (Kearney & Lanius, Citation2022; Kozlowska et al., Citation2015; Maren, Citation2001; Morena et al., Citation2016; Teicher & Samson, Citation2016).
The pain system is not only important in bringing aversive stimuli to our attention, but also integral to the stress response (Eisenberger, Citation2012; Slavich, Citation2016; Slavich et al., Citation2010a). In animal studies, fight or flight is associated with non-opioid (i.e., endocannabinoid) analgesia with projections blocking pain signals ascending from the spinal cord, whereas the freeze response is associated with opioid-mediated analgesia coordinated by the PAG and the rostral ventromedial medulla pain circuit (Kozlowska et al., Citation2015; Lanius et al., Citation2018). The pain system supports the stress response by (a) decreasing pain and, thus, increasing physical ability; (b) producing aversion and, thus, increasing motivation to respond to the threat; and (c) altering consciousness and increasing dissociative-type symptoms. The endocannabinoid system is also involved in memory consolidation in highly arousing situations by facilitating the extinction of emotionally aversive memories, promoting neurogenesis and synaptic plasticity, and regulating reward (for a review, see Morena et al., Citation2016).
Emerging research is also linking the stress response with our vestibular system and gut-brain axis. The vestibular system detects motion through inner ear organs, orients and navigates our bodies in space, engages the ANS and reticular activating system to maintain posture and motion, and supports a felt sense of security, grounding, physical and emotional safety, and balance (Kearney & Lanius, Citation2022; Saman et al., Citation2012; Saman, Citation2020). Our intestinal system is an additional bi-directional interface with our external environment. The microbiome provides signals to our lower brain through cranial nerve X (i.e., the vagus nerve), as well as tryptophan metabolism and serotonergic neurotransmission. Stress is associated with changes in composition, diversity, and metabolic activity of the gut microbiota (Molina-Torres et al., Citation2019; O’Mahony et al., Citation2015). In addition, changes in the microbiome have been shown to impact HPA axis development in animal model systems (O’Mahony et al., Citation2015).
Repeated activation of the sensorimotor system may lead some individuals to have increased sensitivity to pain and “feel too much” whereas others may become tolerant or numb to pain and have “chronic detachment from bodily sensations” (Kearney & Lanius, Citation2022; Lanius et al., Citation2015). In addition, if threatening stimuli are repeatedly experienced, they can come to feel familiar, thus breaking down the ability to detect what is threatening or not (Perry & Szalavitz, Citation2017; Perry & Winfrey, Citation2021; Young et al., Citation2016). Chronic detachment and numbness is also associated with thrill seeking behavior as a way to engage our salience network (see Box 2) and gain access to our inner feelings (Lanius et al., Citation2015). These alterations in sensory processing can lead to feelings of being disconnected from, unsafe in, and unable to control one’s own body (Kearney & Lanius, Citation2022; Van der Kolk, Citation2015). In this way, clinicians can better understand the biological underpinnings of stress-related pain disorders, somatization issues, self-harming, and thrill-seeking behaviors, as well as aspects of dissociation (in particular derealization and depersonalization), as each of these pain-related disorders, issues, and behaviors involve somatosensory, endocannabinoid, and endogenous opioid pathways.
Assessment
Numerous self-report assessment tools exist for measuring sensory processing, balance, and pain, such as the Sensory Processing Measure (SPM) (Brown et al., Citation2023), Sensory Profile (Dean et al., Citation2016; Dunn, Citation1999), Sensory Processing Scale Inventory (Schoen et al., Citation2017), Dizziness Handicap Inventory (DHI) (Zamyslowska-Szmytke et al., Citation2021), Vestibular Activities & Participation (VAP) Questionnaire (Alghwiri et al., Citation2012), Vertigo Symptom Scale (VSS) (Wilhelmsen et al., Citation2008), Pain interference PROMIS short version (Amtmann et al., Citation2010), McHill Pain Questionnaire (Melzack & Raja, Citation2005), Pain Catastrophizing Scale (Sullivan et al., Citation1995), and the Physical & Emotional Subjective Units of Discomfort Scales (SUDS) (Tanner, Citation2012). Gross sensorimotor and vestibular alterations can be evaluated using physical exam techniques such as gently touching the skin with a pin, cotton swab or vibrating tuning fork, nystagmus testing, the head impulse test, the Romberg test, and walk heel-to-toe on a straight line. Biomarkers include hair endocannabinoids (Shonkoff et al., Citation2022) and lipid extracts of serum or plasma endocannabinoids (Hillard, Citation2018).
Endogenous opioids are more difficult to measure. Positron Emission Tomography (PET) is an indirect measure of changes in opioid receptor occupancy using radiolabeled agonists (Conway et al., Citation2022). Sensory processing can also be measured through nerve conduction studies (NCS) and functional magnetic resonance imaging (fMRI) (Ahmad & Zakaria, Citation2015). In addition, EEG and Evoked Reaction Potentials (ERPs) have been shown to distinguish children with sensory processing disorders from typically developing children with 86% accuracy in a small study (Davies & Gavin, Citation2007). Wearable devices such as smartwatches and activity trackers can measure markers such as heart rate and skin conductance in response to daily sensory experiences (Black et al., Citation2020; Kaski & Seemungal, Citation2010; Roos & Slavich, Citation2023). There are also several health technologies such as diary apps that can help individuals monitor pain and vestibular symptoms (Martin et al., Citation2020), as well as emerging software to access smartphone accelerometers to assess balance (Patterson, Citation2014).
Interventions
Somatic therapies including Sensorimotor Psychotherapy (Ogden & Minton, Citation2000), Somatic Experiencing (Kuhfuß et al., Citation2021), and Sensory Motor Arousal Regulation Therapy (SMART) (Warner et al., Citation2014) help people become more aware of their somatic sensations, increase understanding and tolerance of uncomfortable sensory associations, and link new positive sensory experiences with safety and connectedness (Kearney & Lanius, Citation2022). Eye Movement Desensitization & Reprocessing (EMDR) incorporates bilateral visual or tactile sensations into the therapy practice and is hypothesized to reprocess the physical sensations, emotions, and disturbing images that arise when remembering the traumatic event (Shapiro, Citation2014; Wilson et al., Citation2018). For people with decreased body awareness, techniques such as body scan meditation and progressive muscle relaxation may be particularly useful as they can increase body awareness and help to associate body sensations with specific emotions. These can be important therapeutic interventions in overcoming emotional detachment and potentially restoring salience network function (Lanius et al., Citation2015).
Repetitive, rhythmic, patterned stimuli are soothing and can help re-associate physical sensations with safety (Perry, Citation2009). Play therapy, expressive arts therapy, and animal-assisted therapy also provide opportunities for somatic, rhythmic, and vestibular sensory experiences within the setting of a safe, supportive, nurturing relationship and environment (Fisher, Citation2019). Interventions to support skill building and regulation of the vestibular system could include occupational therapy, physical therapy, as well as physical activity such as yoga, tai chi, Qui-Gong, and adventure-based programs with balance-based activities (e.g., walking on logs, climbing rocks). Potential interventions supporting microbiome and gut-brain axis health include probiotics, prebiotics, dietary changes, and fecal transplant (Molina-Torres et al., Citation2019).
Medications that target the endocannabinoid and endogenous opioid systems deserve more research attention and may improve pain modulation and stress-related symptoms such as anxiety, depression, emotional shut down, and dissociation. Kappa receptor antagonists, for example, may disrupt dissociative symptoms including depersonalization and derealization and are a potential therapeutic target for dynorphin-associated toxic stress responses (Carlezon & Krystal, Citation2016; Lanius et al., Citation2018). A growing literature is showing the anti-inflammatory properties of low dose naltrexone as well as its ability to upregulate endogenous opioid signaling and improve pain symptoms associated with fibromyalgia, and complex regional pain syndrome (CRPS) (Toljan & Vrooman, Citation2018; Younger et al., Citation2014). Effective pain control and higher morphine doses after trauma have been associated with fewer PTSD symptoms and decreased likelihood of developing PTSD (Lanius et al., Citation2018). Cannabis use can dampen neuronal firing in the amygdala and increase reactivity of the ventro-medial prefrontal cortex (vmPFC) in response to emotional stimuli, and cannabinoid receptor agonists such as nabilone have been shown to reduce nightmares and hyperarousal in patients with PTSD (Lanius et al., Citation2018).
Understanding the mechanistic actions of different psychedelics through the lens of the Stress Phenotyping Framework could help identify which psychedelic might be best suited for different clinical phenotypes. For example, ketamine, commonly used as an anesthetic and analgesic, can facilitate psychotherapy and shows promise for the treatment of PTSD (Drozdz et al., Citation2022; Neuhaus & Slavich, Citation2022). One mechanism of action for ketamine is to act as an N-methyl-D-aspartate receptor (NMDAR) antagonist and decrease central pain sensitization (Zhou et al., Citation2011). Thus, ketamine may prove to be particularly useful in treating patients with dysregulation of somatosensory and pain pathways. In contrast, methylenedioxymethamphetamine (MDMA) is thought to influence serotonin, norepinephrine, cortisol, and oxytocin pathways (Reiff et al., Citation2020). Therefore, MDMA may prove to be more helpful in patients needing stimulation of their arousal and ANS, and regulation of their HPA axis and relational systems. (See those systems described below.)
Arousal and energy
Orienting to potential danger, being alert and sensitive to stimuli, and having energy to address potential challenges and threats are critical features of the stress response system. The superior colliculus receives somatosensory input and sends afferents to oculomotor neurons, the reticular formation and motor neurons controlling the eyes, head, and neck, to initiate a subconscious orienting response to threatening visual stimuli as well as nurturing interpersonal contact (Kearney & Lanius, Citation2022). The superior colliculus, locus coeruleus, and thalamic pulvinar can engage the amygdala and cortical orienting networks without conscious awareness to provide a rapid and automatic alert system and initiate arousal and vigilance (Liddell et al., Citation2005).
Arousal can be conceptualized as a spectrum from “excessive sleepiness, cognitive dysfunction, and inattention on one side, a wakeful state in the middle, and hypervigilance, panic, and psychosis on the other side” (Ross & Van Bockstaele, Citation2020). Our level of arousal and energy determine the intensity of our motivational responses that will facilitate behavior to preserve and ensure survival (Bradley, Citation2009). Potential mechanisms by which ELA and toxic stress may impact energy, arousal, and sleep include stress-related disruptions of our (a) homeostatic sleep drive and circadian rhythm system (Agorastos et al., Citation2019; Agorastos & Olff, Citation2021; Fuligni et al., Citation2021; Koch et al., Citation2017; Radwan et al., Citation2021); (b) gastrointestinal system digestion and processing of glucose and lipids (Tosato et al., Citation2020); (c) mitochondrial energy production (glucose and oxygen metabolism, which can also lead to oxidative stress) (Bersani et al., Citation2020; Picard & McEwen, Citation2018; Zitkovsky et al., Citation2021); (d) connectivity changes of the salience network, default mode network (see Box 2) (Zhang et al., Citation2022), and reticular activation system function (Thome et al., Citation2019); (e) ANS regulation (see below) (Jerath et al., Citation2018); and (f) neuronal electrical activity recorded as delta, theta, alpha, beta, and gamma waves in order of increasing frequency (Jokić-begić & Begić, Citation2003). Repeated activation of the stress response, put in motion by past ACEs and trauma, is associated with dysregulation of the aforementioned mechanisms and increases the risk for chronic fatigue syndrome (Heim et al., Citation2006), sleep problems including increased and decreased sleep as well as nightmares and disruptive nocturnal behaviors (e.g., moaning, thrashing, tossing and turning) (Brock et al., Citation2019; Greenfield et al., Citation2011; Kajeepeta et al., Citation2015; Sadeh, Citation1996), and behavioral and mental health problems such as depression, anxiety, and bipolar disorder, which all involve arousal dysregulation (MacKinnon, Citation2008).
Assessments
Given the complexity of arousal as a construct, there are numerous potential biomarkers including: (a) levels of neurotransmitters such as orexin, catecholamines, gamma aminobutyric acid (GABA), glutamate, acetylcholine, serotonin, adenosine (Chellappa & Aeschbach, Citation2022; Linnstaedt et al., Citation2019), and melatonin (Caumo et al., Citation2019); (b) markers of oxidative stress such as F2 isoprostane (Horn et al., Citation2019); (c) imaging techniques including quantitative electroencephalogram (qEEG), evoked response potentials (ERPs), and fMRI; and (d) polysomnography (Fabbri et al., Citation2021). However, this field of stress biomarker research is still in its infancy, and there is a pressing need for additional studies to identify the best methods for collecting and analyzing biomarkers to yield clinically actionable metrics.
Self-report questionnaires for arousal include the Profile of Mood States arousal subscale (Boyle, Citation1987), Activation-Deactivation Adjective Check List (AD ACL) (Thayer, Citation1986), and Self-Assessment Manikin (Bradley & Lang, Citation1994). Common self-reported sleep questionnaires include the Pittsburgh Sleep Quality Index (PSQI) used to assess overall sleep quality, quantity, and disturbances; Epworth Sleepiness Scale (ESS) for assessing excessive daytime sleepiness; Insomnia Severity Index (ISI) to measure severity of insomnia symptoms; and STOP-Bang questionnaire for assessing presence of obstructive sleep apnea (Chung et al., Citation2016; Fabbri et al., Citation2021). Circadian rhythm is commonly measured through the Morningness-Eveningness Questionnaire (Horne & Ostberg, Citation1976). Additionally, there are many wearable sleep trackers that have yielded promising results (Berryhill et al., Citation2020). In more severe cases, polysomnography (sleep study) can identify alterations in slow wave & REM sleep and sleep efficiency as well as other medical conditions such as Obstructive Sleep Apnea (Kim & Dimsdale, Citation2007; Zhang et al., Citation2019).
Interventions
Neurofeedback is a form of biofeedback using EEG data or blood oxygenation levels (functional near infrared spectroscopy or fNIRS) that primarily targets arousal. EEG or fNIRS data are used to create images on a computer screen that have been programmed to provide positive feedback when the individual is creating the desired brain pattern of arousal. Alpha neurofeedback training has been shown to improve anxiety, attention, and modulate SN and default mode network (DMN) functional connectivity (Fisher, Citation2019; Lanius et al., Citation2015). Deep Brain Reorienting (DBR) is an emerging therapy that specifically focuses on muscle activation and tension in the neck associated with trauma and is hypothesized to integrate past orienting response experiences with current safety (Kearney & Lanius, Citation2022).
Sleep interventions can include cognitive and behavioral interventions, relaxation techniques, physical activity, balanced nutrition as well as medications and supplements that target stress-related disruptions (Briguglio et al., Citation2020; Murawski et al., Citation2018). When possible, it is important to address current stressors that may keep people from being able to sleep. Techniques that can increase the feeling of safety include nightlights, weighted blankets, or co-sleeping with a trusted adult. Strategies that can calm an overactive arousal or ANS include breathing techniques or mindfulness practices (see next section) (Rusch et al., Citation2019). Journaling and creating “to do” lists can help release worries and decrease nighttime rumination (Scullin et al., Citation2018). Light therapy can help reset the circadian rhythm and homeostatic sleep drive (Blume et al., Citation2019). Medications such as melatonin may help counter the dysregulation of circadian rhythms in traumatic stress (Agorastos et al., Citation2020) and prazosin may improve PTSD-associated nightmares (Akinsanya et al., Citation2017; Bhushan et al., Citation2020; De Berardis et al., Citation2015; George et al., Citation2016). Targeted cognitive-behavior therapy for insomnia (CBT-I), considered the gold-standard for insomnia treatment, can also treat PTSD-related insomnia, and online and mobile apps (iCBT-I) can be an adequate alternative when needed (Muench et al., Citation2022). Tai Chi, a moving meditation, can improve sleep, pain, and psychological well-being (Raman et al., 2013) and was shown to be non-inferior to CBT-I in cancer survivors (Irwin et al., Citation2014, Citation2017).
Reward processing
Although ELA and PTSD are associated with disruptions of the reward processing pathways, less is known about the relation between the reward processing pathway and the acute stress response. Both reward and fear pathways are involved in approach (fight) or avoid (flee, freeze) behaviors. In animal models, the PAG, a key structure in the stress response, has been shown to project to both dopamine and GABA neurons in the ventral tegmental area (VTA), a key structure in the reward processing pathway (Ntamati et al., Citation2018). Mild-to-moderate controllable stressors have been shown to activate dopamine release, whereas severe, chronic, unavoidable, and unpredictable stressors tend to inhibit dopamine release (Baik, Citation2020). Neurobiological changes associated with ELA include alterations in connectivity between the ventral striatum, VTA, and PFC, VTA morphology, dopaminergic and GABAergic signaling and receptor expression, as well as transcription factors and epigenetics (Hanson et al., Citation2021). Behaviorally, ELA has been associated with decreased approach motivation and reward responsivity (Hanson et al., Citation2021; Le et al., Citation2023; Novick et al., Citation2018). Blunted reward responsivity may increase reward-seeking and thrill-seeking behavior as it takes more reward to induce pleasure. Reward-seeking can be an adaptive strategy in high-adversity, resource-scarce environments (Duffy et al., Citation2018). In addition, ACEs & ELA are associated with increased risk for addictions including smoking, alcohol, drugs, gambling, and food (Birnie et al., Citation2020; Hanson et al., Citation2021; Hendrikse et al., Citation2022; Leza et al., Citation2021; Lokshina et al., Citation2021; Wiss et al., Citation2020), which have been described as “self-medication” and “self-soothing” coping behaviors in response to emotional pain (Schimmenti et al., Citation2022).
Assessments
Potential biomarkers for the reward system include dopamine, GABA, and adenosine (Deighton et al., Citation2018; Hanson et al., Citation2021; Linnstaedt et al., Citation2019). Testing paradigms have been used in research settings to evaluate constructs of reward including reward responsiveness, learning, and valuation. Examples of tests include Delay Discounting (Lempert et al., Citation2012), Willingness to Pay Task (Plassmann et al., Citation2007), Effort Expenditure for Rewards Task (Treadway et al., Citation2009), and the Go/No-Go task (Korgaonkar et al., Citation2021). In addition, resting state fMRI can help visualize the activity of the insula and salience network, as well as analyze the VTA and substantia nigra, and dopaminergic reward regions (Herzberg & Gunnar, Citation2020).
Interventions
Interventions for reward processing dysregulation and addiction using the Stress Phenotyping Framework could include (a) providing skills for healthy coping strategies and distress tolerance, and (b) offering therapeutic interventions that specifically target reward processing pathways (Dutcher, Citation2023; Garland, Citation2020; Maté, Citation2008; Ryan et al., Citation2022). Dual diagnosis treatment therapy recognizes the need for multidisciplinary treatment and is a step toward an integrated Stress Phenotyping Framework that treats dysregulation in the reward processing system, as well as other stress-related systems. Emerging therapies that may be specifically helpful in targeting reward processing include meditation (Kjaer et al., Citation2002), Transcranial Magnetic Stimulation (Ryan et al., Citation2022), positive affect treatment (Craske et al., Citation2023), brain training for cognitive reappraisal for cravings (Fisher & Berkman, Citation2015), neurofeedback (Greer et al., Citation2014), ketamine-assisted psychotherapy (Drozdz et al., Citation2022), and other psychedelic-assisted therapies (Reiff et al., Citation2020).
Autonomic nervous system
Sensory information is brought to the amygdala, which detects threat and further signals ANS and HPA-axis activation (Kozlowska et al., Citation2015; Teicher et al., Citation2016). As the earliest actor in the response to threat, the ANS is intricately connected to arousal pathways and elicits activation of the stress response through its sympathetic (SNS) and parasympathetic (PNS) branches (Elbers et al., Citation2018; Slavich et al., Citation2010b). Bidirectional pathways connect the ANS with hormonal, cardiovascular, digestive, immune, and inflammatory systems, which further mediate physiological stress responses in the body; at the same time, there is complex interplay between the ANS and cognitive and emotional centers in the brain (Thayer et al., Citation2009). Under resting conditions, the parasympathetic system predominates, eliciting the body’s vital rest, repair, and digest functions. Acute stress triggers a shift in the autonomic balance typically characterized by parasympathetic withdrawal and sympathetic activation, which, in conjunction with activation of the HPA axis, prepare the body for “fight or flight” (Kim et al., Citation2018).
In animal models, under conditions of extreme threat, concurrent reactivation of the parasympathetic system can instead activate a state of bradycardia, hypotension, attentive immobility with hypothalamic muscle tone maintained (Kozlowska et al., Citation2015). In the setting of restraint, and perceived inescapable threat, there is tonic immobility associated with withdrawal of SNS activity, increased PNS activity, heart rate and blood pressure decrease, and one remains very still with continued hypothalamic controlled muscle tone. Ultimately, the increased PNS activity can lead to collapsed immobility (fainting is an extreme example) when the associated bradycardia leads to hypoxia and a loss of muscle tone (Kozlowska et al., Citation2015; Lanius et al., Citation2018; Roelofs & Dayan, Citation2022).
Autonomic dysregulation is commonly observed in chronic stress studies. In a literature review looking at childhood maltreatment, studies suggested a general trend of blunted cardiovascular activity in response to stress compared to children who had not been maltreated (Young-Southward et al., Citation2020). Results of sympathetic responses were more mixed, with some studies showing sympathetic activation, whereas others showed blunted sympathetic responses (Young-Southward et al., Citation2020). The authors hypothesized that differences in ANS responsivity may influence psychopathology risk for maltreated children (Young-Southward et al., Citation2020).
Assessments
Disturbances of ANS activity play a critical role in stress-related conditions; therefore, assessing autonomic function is essential for detecting toxic stress. Relevant tests may include measures of cardiovascular, adrenergic, cardiovagal, and sudomotor functioning (Cheshire et al., Citation2021). Given the complexity of the ANS, however, a thorough evaluation often involves a battery of tests and provocative maneuvers.
Analysis of heart rate variability has recently become the most popular and accessible method of testing, now being included in wearable devices (Grégoire et al., Citation2023). Heart rate variability is a measure of the variation between successive heart beats and reflects the dynamic interplay between the sympathetic and parasympathetic branches of the ANS. High HRV is generally associated greater emotional and physical health, indicating autonomic flexibility and adaptability to stress and other energetic demands. On the other hand, reduced HRV occurs with age but also due to chronic stress or illness, and has been identified as an independent predictor of all-cause mortality (Tsuji et al., Citation1994).
Other tests of autonomic cardiovascular reflexes include the Valsalva maneuver, deep breathing, isometric handgrip test, cold pressor test, active standing (orthostatic), head-up tilt test, baroreflex sensitivity testing, and mental arithmetic. Simple measures that reflect autonomic function include heart rate, respiratory rate, and blood pressure (Deighton et al., Citation2018). Biomarkers that can assess ANS activity include urine and plasma norepinephrine, epinephrine, and salivary alpha amylase (Ali & Nater, Citation2020; Deighton et al., Citation2018; Djuric et al., Citation2008; Linnstaedt et al., Citation2019; Wiley et al., Citation2016; Zygmunt & Stanczyk, Citation2010).
Interventions
Body-based therapies are a complementary or alternative treatment modality that regulate the nervous system through a “bottom-up” approach. Autonomic responsivity is a lower brain, automatic, instinctual process that is outside of our conscious awareness, and thus harder to target with “top-down” approaches that involve higher level cognitive “thinking brain” functions (Perry & Hambrick, Citation2008). Therefore, bottom-up interventions that involve the body may more directly target physiologic stress activation, and facilitate awareness and experience of somatic sensations (Kearney & Lanius, Citation2022).
Bottom up approaches that help to regulate the body’s physiologic stress response include interventions such as heart rate variability biofeedback (e.g., HeartMath) (Fournié et al., Citation2021; Lehrer et al., Citation2020), yoga (Kearney & Lanius, Citation2022), and somatic sensory-based psychotherapies (Kearney & Lanius, Citation2022) such as Somatic Experiencing, Sensorimotor Psychotherapy, and Sensory Motor Arousal Regulation Therapy (SMART). In addition, breathing practices such as prolonged expiratory or coherent breathing can activate parasympathetic function, which, over time, helps to increase self-regulatory capacity of the ANS (Balban et al., Citation2023; Kearney et al., Citation2023; Komori, Citation2018).
Patients who experience severe symptoms of dysautonomia, including orthostatic hypotension or neurocardiogenic syncope, may benefit from pharmacological management with beta-blockers or midodrine, a selective peripherally acting alpha-receptor agonist (Raj et al., Citation2009; Thijs & van Dijk, Citation2006). Arnsten and colleagues (Citation2011) have found that alpha-2-adrenergic agonists including clonidine and guanfacine, can balance noradrenaline release and functionally increase limbic connectivity with the prefrontal cortex. This is an important consideration for children mis-diagnosed with ADHD who actually have developmental trauma associated with increased noradrenaline activity and decreased prefrontal cortex connectivity (Arnsten & Pliszka, Citation2011; Bhushan et al., Citation2020; Neuchat et al., Citation2023; Ortiz et al., Citation2022).
Hypothalamic-pituitary-adrenal axis and endocrine processes
Stress and ELA impact hormonal and endocrine processes, the most notable of which is the HPA axis. In response to a stressor, the amygdala signals the periventricular nucleus of the hypothalamus to release corticotropin releasing factor (CRF), which signals the anterior pituitary release of adrenocorticotropin hormone (ACTH), resulting in the release of cortisol from the adrenal cortex (Berens et al., Citation2017; Bucci et al., Citation2016). Whereas the ANS response to a potential threat is within milliseconds to seconds, the downstream effects of cortisol are apparent on the scale of minutes to days.
Generally, cortisol effects include increasing blood pressure, cardiac output, water excretion, blood sugar levels, and appetite (specifically for energy-dense foods such as carbohydrates and fats), as well as suppressing sleep, the immune system, reproduction, and growth (Sapolsky et al., Citation2000). Over time, ELA and chronic stress result in altered cortisol signaling, including both under and overproduction of cortisol (). The exact biological mechanisms that lead to these disparate patterns vary based on sex, stressor type, and stressor timing; however, prenatal stress and threat-based ELA are more likely to result in hyper-reactivity, whereas severe stress, abuse, and deprivation-based ELA are more likely to result in hypo-reactivity (Van Bodegom et al., Citation2017). Although the body will attempt to adapt to these conditions through altered gene expression of cortisol receptors, these adaptations often result in ineffective and dysregulated HPA axis-mediated stress responses and glucocorticoid resistance (Bhushan et al., Citation2020; Herman et al., Citation2016; Jarcho et al., Citation2013; Miller et al., Citation2007; Miller & Chen, Citation2006).
Figure 2. The Stress Phenotyping Framework and the potential for identifying stress phenotypes. (A) Commonly used stress response cluster model. Stress reactivity clusters are often simplified to reactive or blunted and focus on ANS and HPA axis reactivity. This model can be very helpful in the clinical encounter to quickly describe complex processes to clients and patients. However, people likely differ in their stress reactivity across systems and may need a broader assessment and treatment strategy. (B) The Stress Phenotyping Framework. Within each of the stress-related systems described in this narrative review, an individual could have a reactive (↑), blunted (↓), disorganized (bi-directional arrow), or well-regulated (∼) stress response. We suggest that clinicians can provide more targeted interventions by identifying individual differences across different stress-related systems. In addition, we hypothesize that future research can identify clinically distinct stress-response clusters or “stress phenotypes” that could further predict health behaviors and disease risks and provide an opportunity to advance therapeutic interventions. (C) Potential patterns of cortisol reactivity to acute stress (adapted from McEwen, Citation2000a, Citation2000b, Citation2006). This graph depicts an example of differential regulation of HPA-axis reactivity. Mapping the patterns of dysregulation for all stress-related systems following ELA and chronic stress may better inform classification of common stress phenotypes and targeted therapeutic interventions.
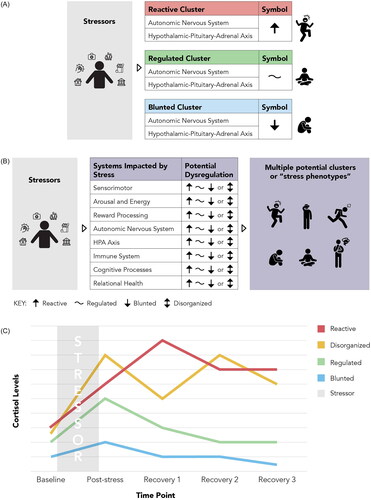
Beyond cortisol dysregulation, ELA and chronic stress are associated with altered metabolic hormone signaling as well. Evidence suggests that glucagon-like peptide-1 (GLP-1) and leptin (satiety cueing hormones) and ghrelin (hunger cueing hormone) become altered in response to chronic stress, in part due to acute stress responses (Raspopow et al., Citation2010, Citation2014; Tomiyama, Citation2019; Tomiyama et al., Citation2012). For example, leptin is released during the acute stress response, functioning to curb appetite while organisms manage the stressor at hand; over time, however, its release in the absence of satiety can lead to insensitivity to leptin, such that organisms begin to eat in the absence of hunger. Indeed, children as young as seven years old who have experienced ELA have been found to eat in the absence of hunger (Proffitt Leyva et al., Citation2020). Beyond cortisol and metabolic hormone dysregulation, ELA and chronic stress are associated with reduced sex steroid hormones levels (Jasienska et al., Citation2017; Kreuz et al., Citation1972; O’Brien et al., Citation2007; Palm-Fischbacher & Ehlert, Citation2014; Retana-Márquez et al., Citation2003; Schliep et al., Citation2015), decreasing fertility for both sexes and altering women’s ovulatory cycle characteristics.
Assessments
Biomarkers of HPA-axis function include salivary and serum cortisol, diurnal cortisol assessments (taken multiple times per day across multiple days), the cortisol awakening response, dehydroepiandrosterone (DHEA), ACTH, CRF, arginine vasopressin (AVP), calculating a cortisol/DHEA ratio (Ahmed et al., Citation2023; Deighton et al., Citation2018; Djuric et al., Citation2008; Linnstaedt et al., Citation2019; Piazza et al., Citation2010), as well as assessments of hair cortisol levels, cortisone, and DHEA (Shonkoff et al., Citation2022). In laboratory-based research, salivary cortisol levels before, during, and after an acute stress task are the gold standard for assessing cortisol reactivity, whereas diurnal assessments are more standard for generalized HPA axis function assessments. Assessment of hair cortisol levels are an emerging approach to better understand circulating levels of cortisol on a longer timescale (i.e., over the last few months) as opposed to salivary or serum levels of cortisol, which are more momentary assessments.
Assessments of metabolic dysregulation include fasting serum levels of metabolic hormones (e.g., leptin, ghrelin, GLP-1), along with blood pressure, glucose, hemoglobin A1c, insulin resistance, cholesterol levels, high-density lipoprotein, low-density lipoprotein, and triglycerides (Deighton et al., Citation2018; Joung et al., Citation2014; McEwen, Citation2015; Tomiyama et al., Citation2012; Wiley et al., Citation2016; Yam et al., Citation2015; Yousufzai et al., Citation2018). Further, functional assessments, in which blood samples and self-reported hunger assessments are collected at baseline and following a personally tailored food intake session, can provide deeper insights into how an individual’s metabolic hormones respond to eating, and how changes in hormone levels correspond with changes in hunger and satiety.
Interventions
Interventions in this category should focus on stress-reduction strategies that target the HPA axis and cortisol production, as cortisol dysregulation has many downstream effects. First, external stressors that impact safety must be identified and resolved whenever possible. Next, trauma-informed lifestyle interventions—also called “stress busters” by the ACEs Aware initiative in California—can generally help regulate the brain-body stress pathways (Bhushan et al., Citation2020; Gilgoff et al., Citation2020). Supportive relationships, quality sleep, and regular physical activity have all been associated with HPA axis regulation (Bhushan et al., Citation2020; Gilgoff et al., Citation2020), and intervention approaches that incorporate these factors should be considered.
Mindfulness and meditation (Koncz et al., Citation2021; Pascoe et al., Citation2017), experiencing nature (Jones et al., Citation2021), and psychosocial interventions (Purewal Boparai et al., Citation2018; Slopen et al., Citation2014) have been shown to help regulate cortisol levels as well. Specifically, mindfulness has been shown to improve physiologic markers of stress including cortisol, C-reactive protein (CRP), tumor necrosis factor-α (TNF-α), blood pressure, heart rate, and triglycerides, and may be particularly helpful for patients with elevated cortisol levels (Black & Slavich, Citation2016; Gilgoff et al., Citation2022; Koncz et al., Citation2021; Pascoe et al., Citation2017). Intuitive eating interventions are a promising method of regulating eating behavior in those with dysregulated metabolic hormone levels, although further work with a dietician or disordered eating specialist may be necessary. MDMA has been shown to increase cortisol levels, decrease anxiety, and reduce impaired fear recognition (Dolder et al., Citation2018), and MDMA-assisted psychotherapy has been shown to be efficacious in treating PTSD (Reiff et al., Citation2020).
Immune system
One of the primary ways that stressor exposure impacts health across the lifespan is by activating the immune system (Elwenspoek et al., Citation2017; Furman et al., Citation2019; Slavich, Citation2015; Slavich & Auerbach, Citation2018). Maternal stressors impact the immune development of offspring in-utero and can result in the development of a Th2-biased immune system, along with elevated allergic rates of disease (Entringer et al., Citation2012, Citation2015; Marques et al., Citation2013; Suh et al., Citation2017) and accelerated biological aging (Mayer et al., Citation2023). ELA (experienced ages 0–8) is also associated with the development of a proinflammatory phenotype (Miller et al., Citation2002, Citation2009; Miller & Chen, Citation2010) and allergic sensitization (Lendor et al., Citation2008; Rowe et al., Citation2007) that persists into adulthood and across generations (Chen et al., Citation2017).
The impacts of ELA on immune function likely occur through multiple mechanistic pathways that include neurobiological changes (Brady et al., Citation2022), epigenetic modifications (e.g., increased methylation) (Chen et al., Citation2019; Danese & McEwen, Citation2012; Harb & Renz, Citation2015; Miller & Cohen, Citation2001; Vinkers et al., Citation2015; Wright, Citation2011), changes in gene expression (e.g., upregulated proinflammatory gene expression and downregulated anti-viral gene expression) (Miller & Chen, Citation2006; Slavich et al., Citation2023; Slavich & Cole, Citation2013), and changes in health behaviors (e.g., engaging in riskier health behaviors which elevate health risks, like smoking) (Wright, Citation2011). Acute stress activates the secretion of pro-inflammatory cytokines, which further activate the immune response through the stimulation of both systemic acute-phase proteins (McEwen Citation2006; Steptoe et al., Citation2007) and glucocorticoids, which over time, can promote the development of glucocorticoid resistance or insensitivity (Miller & Chen, Citation2006; Slavich & Irwin, Citation2014). The low-grade inflammatory state that follows ELA or chronic stressor exposure is a risk factor for peripheral immune dysregulation that persists throughout the lifespan (Carpenter et al., Citation2010; Chida et al., Citation2007; Danese et al., Citation2007; Danese & Lewis, Citation2017; Kuhlman et al., Citation2017; Rooks et al., Citation2012; Wei et al., Citation2012), leading to increased risk of chronic diseases of immune origin (Danese & Lewis, Citation2017; Glaser & Kiecolt-Glaser, Citation2005; Kuhlman et al., Citation2017; McEwen, Citation2006). Further, because the upregulation of inflammation and innate immune function often come at the cost of downregulation of anti-viral immune function, those with elevated inflammation are also more susceptible to viral disease (Slavich & Cole, Citation2013).
Assessments
Currently available assessment tools for immune system function that can be used in clinical practice include complete blood count with differential to evaluate for eosinophilia, high-sensitivity CRP, and fibrinogen as markers of general inflammation, Total IgE, Aeroallergen panel, FeNO as markers of airway inflammation (may be performed by specialist), and pulmonary function tests (may be performed by specialist). Research biomarkers include interleukins, interferon, TNF-α and its downstream marker soluble TNF-receptor type 2 (sTNF-R2) (Deighton et al., Citation2018; Djuric et al., Citation2008; Linnstaedt et al., Citation2019). Generally, inflammation levels should be assessed at baseline using CRP, or in response to a laboratory-based stressor using cytokine responses, as cytokine levels change rapidly, whereas CRP levels are more stable over time.
Patients with asthma and a history of ELA or chronic stress may not respond as well to traditional medications of albuterol and steroids. As such, those who have experienced major life stressors may benefit from dexamethasone suppression tests to determine if glucocorticoid insensitivity might impact medication efficacy. Although these are clinically performed in vivo, in vitro assessments may also prove useful in this context. Moreover, researchers may want to assess more than just inflammation levels and might also consider using functional immune challenges to assess things like natural killer cell cytotoxicity, or phagocytosis capacity of white blood cells, in vitro. This assay enables researchers to expose immune cells to tumors or bacterial threats to measure their functional responses to challenges, providing a model of how an individual’s immune cells likely behave in response to naturally occurring, ecologically valid immune challenges.
Interventions
Often, interventions assessed to regulate or improve inflammation and immune function are designed to improve another outcome (e.g., disease symptoms, depression, stress) and use inflammation levels as a marker or mediator of these effects. However, several studies have shown that stress-mitigation strategies (e.g., mindfulness, meditation, yoga, Tai Chi) as well as psychosocial interventions (e.g., CBT, behavior therapy, mindfulness), and medication (e.g., SSRIs) can help normalize immune function (Black & Slavich, Citation2016; Gilgoff et al., Citation2020; Hewson-Bower & Drummond, Citation2001; Shields et al., Citation2020; Wang & Young, Citation2016). Positive psychological and behavioral states including positive affect, eudaimonic well-being (meaning and engagement), physical activity, and sleep have all been demonstrated to have beneficial effects on the immune system (Bower et al., Citation2019).
When the primary goal is to decrease inflammation, anti-inflammatory diets and immune modulating supplements including curcumin, ginger, and omega-3 fatty acids are encouraged, and may be particularly helpful for people in which ELA and stress are leading to increased inflammation (Jalali et al., Citation2020; Kiecolt-Glaser, Citation2010; Kiecolt-Glaser et al., Citation2014; Morton et al., Citation2021; Portnoy et al., Citation2018). Moreover, physical activity can increase immune cell counts and cytokine levels during the activity and decrease lymphocytes and antibody response afterwards that, over time, are associated with a general anti-inflammatory effect and improved immune function (Gleeson et al., Citation2011). Additional research should be done to evaluate whether higher dosages or additional immune modulator medications improve outcomes for patients with elevated inflammation or glucocorticoid resistance as a result of stressor exposure (Manka & Wechsler, Citation2018). Until this research is conducted, clinicians should consider that patients thought to be non-compliant (i.e., those not using medications as prescribed) might instead have decreased receptor sensitivity/expression for targets of commonly prescribed medications, in turn, causing them to realize less benefit from medications, thus decreasing compliance.
Cognitive processes
The increase in catecholamine levels associated with acute stress has been associated with shifting resources to the salience network at the expense of the executive control network and decreased structural connectivity between the limbic system and prefrontal cortex (Arnsten, Citation2015; Hermans et al., Citation2014; Weems et al., Citation2019). As catecholamine levels increase, there is an associated decrease in connectivity between the limbic system and the prefrontal cortex (Arnsten, Citation2015; Arnsten & Pliszka, Citation2011). Initially, this helps to increase focus and decrease noise; however, excessive catecholamine release leads to increasing disconnection with the prefrontal cortex (Arnsten, Citation2015, Citation2015; Arnsten & Pliszka, Citation2011). The resulting behavioral response has been colloquially called “flipping your lid,” which is beneficial for quick, instinctual, survival responses during imminent danger, and can be contrasted with slower, planning strategies.
In the context of prolonged adversity, however, repeated activation of the salience network (SN) (see Box 2) and limbic systems may lead to altered connectivity within the SN, DMN and central executive network (CEN). This may contribute to disruptions in cognitive functioning including learning, memory and executive function, attention-deficit hyperactivity disorder (ADHD), developmental delay, learning problems, dementia, and memory impairment (Burke et al., Citation2011; Fenster et al., Citation2018; Hughes et al., Citation2017; Lanius, Citation2015; Lund et al., Citation2020; McEwen, Citation2000b, Citation2007; Nelson et al., Citation2020; Ortiz et al., Citation2022). When a child is in “survival mode”, higher cognitive functions such as planning, focus, or top-down impulse control may not develop as robustly over time (Zelazo, Citation2020). In addition, experiencing childhood adversity and traumatic stress have been associated with disruptions in higher-level, abstract patterns of thinking such as (a) distorted cognitions (e.g., “I am unlovable,” “the world is dangerous,” and “I’ll never fit in,”: Slavich et al., Citation2023), (b) rumination (repetitive thinking or dwelling on negative cognitions) (Peters et al., Citation2019), and (c) alterations in the sense of life-meaning and life-purpose (Hill et al., Citation2018).
Memory
Stress impairs multiple types of memory, including working memory, declarative memory, and fear conditioning, leading to a wide variety of memory-related symptoms from intense, intrusive memories to profound amnesia (Kim & Diamond, Citation2002; Shields et al., Citation2017). Distinct neurobiological processes underlie declarative memory (Kim & Diamond, Citation2002; Tottenham & Sheridan, Citation2009), working memory (D’Esposito & Postle, Citation2015), and fear conditioning (Maren, Citation2001), and the timing and dosing of stress hormones may impact memory acquisition and storage (Tottenham & Sheridan, Citation2009). These effects, in turn, could potentially explain how an individual may have difficulty consciously recalling a threatening event [that could have happened to themselves or an ancestor (Dias & Ressler, Citation2014)] but could still have an intact, lower-brain-mediated fear response to an unconscious, conditioned stimuli.
Dissociation
Dissociation involves alterations in consciousness and occurs along a continuum of symptoms ranging from daydreaming and “spacing out” during mundane, routine tasks to depersonalization (“feeling outside of or as if you do not belong to your own body”), derealization (“feeling as though things around you are strange or unfamiliar”), and identity disturbances. Similar to animal models of tonic immobility, dissociation appears to involve the amygdala, hypothalamus, and ventrolateral-PAG leading to decreased SNS and increased PNS signaling and release of endogenous opioids (Lanius et al., Citation2018). Moreover, emerging research is finding that dissociative subtype of PTSD (PSTD-DS) is associated with increased connectivity between the vmPFC, the amygdala, and the PAG consistent with top-down, overmodulation of fear processing and reduced fight/flight type responses (Lanius et al., Citation2018, Citation2015).
Assessments
A full cognitive assessment could easily take over an hour and would involve a detailed history and physical exam (for a review, see Kipps & Hodges, Citation2005). Quick, but incomplete, cognitive rating scales include the mini mental state examination (MMSE) (Tombaugh & McIntyre, Citation1992), the mental test score (MTS) (Hodkinson, Citation1972), and the Addenbrooke’s Cognitive Examination Revised (ACE-R) (Mioshi et al., Citation2006). A formal neuropsychological assessment involves multiple mental tasks to test general ability, memory, language, visuospatial, and executive function (Kipps & Hodges, Citation2005), but may not be sensitive enough to detect subtle, impactful decrements in cognitive functioning. CNS Vital Signs provides comprehensive, computer-based neurocognitive testing and has been used in both clinical and research settings (Gualtieri & Johnson, Citation2006). Moreover, the NIH Toolbox provides a variety of free, validated neuro-behavioral measurements (NIH Toolbox, Citation2023).
There are now several consumer-based wearable devices and mobile applications that measure eye movement, attention, concentration, memory, response time, and visual processing, as well as tracking symptoms (Moore et al., Citation2017; Peake et al., Citation2018). Moreover, commercial headbands and eyewear (with sensors embedded in the ear bridges) using EEG signals and near infrared spectroscopy technology are available to measure brain patterns at home; however, further research on their validity is needed (Peake et al., Citation2018). Finally, imaging tools including fMRI, qEEG, and fNIRS can be used to identify connectivity patterns and arousal states that contribute to cognitive functioning (Lanius et al., Citation2015; Schaal et al., Citation2019; Teicher & Samson, Citation2016).
Interventions
Evidence-based interventions that use top-down approaches (i.e., involving our “thinking brain” and improving cognitive control over our behavioral responses) include Trauma-Focused Cognitive Behavioral Therapy (TF-CBT) (Kar, Citation2011; Lorenc et al., Citation2020; Ramirez de Arellano et al., Citation2014), Dialectical Behavior Therapy (DBT) (Bohus et al., Citation2020), and Prolonged Exposure Therapy (Powers et al., Citation2010). A systematic review of fMRI findings with PTSD therapies including TF-CBT, EMDR (only one combined study with TF-CBT), and exposure therapies found a suggestion of increased activation in the medial PFC and rostral anterior cingulate cortex after therapy and no convincing evidence of amygdala changes (Manthey et al., Citation2021). Another review found that successful psychotherapy of PTSD across various therapy types (e.g., CBT, EDR, exposure therapy, mindfulness) was associated with decreased amygdala and insula activity, and increased dACC and hippocampal activity suggesting “regained top-down control” (Malejko et al., Citation2017). Another review found that both EMDR and TF-CBT deactivated the amygdala and activated the hippocampus, mPFC, and ACC, and, in addition, EMDR showed more deactivation of insula and hindbrain regions (Pierce & Black, Citation2023).
Research suggests that the neurons that process fear acquisition and recovery are different than the neurons that are used for fear extinction (Lacagnina et al., Citation2019). Therefore, exposure therapy may create new neuronal pathways that suppress or circumvent the fear memory; however, the original fear memory may still be encoded in other parts of the hippocampus and may be re-activated at a later time (Lacagnina et al., Citation2019). This raises the question as to whether other therapies, such as the bottom-up approaches described earlier, could “rewrite or delete” the original fear encoding and may thus be important complimentary interventions.
In addition to psychosocial interventions, lifestyle approaches and brain training programs can also improve cognitive function. Physical activity is associated with increased hippocampal perfusion, volume, neurogenesis, and synaptic plasticity (Erickson et al., Citation2011; Firth et al., Citation2018; Kandola et al., Citation2016; Li et al., Citation2017), and further research could evaluate the role of physical activity in reversing hippocampal changes associated with ELA. In addition, brain training is an emerging intervention that can help people practice needed or lagging skills and strengthen specific brain circuits (Lanius et al., Citation2015; Nouchi et al., Citation2013; Scionti et al., Citation2019).
Attachment and relational health
On a societal level, supportive social networks are critical to our survival, especially during times of threat (Slavich et al., Citation2022). Social threats such as social evaluation, rejection, devaluation, and exclusion have been shown to strongly induce the stress response (Slavich, Citation2020, Citation2022; Slavich et al., Citation2023). Studies in children and adults have found that supportive relationships and interpersonal touch can boost oxytocin production, reduce cortisol levels and sympathetic arousal, improve immune function, and decrease the risk for heart disease (Afifi et al., Citation2011; Grewen et al., Citation2005; Holt-Lunstad et al., Citation2010; Slopen et al., Citation2014; Uchino et al., Citation2012).
On an individual level, however, the impact of social relationships can be more complex. Attachment describes the early emotional bond between an infant and their caregiver that establishes foundational safety and trust within relationship. A secure attachment pattern is established when an infant’s arousal elicits a reliable, attuned response from their caregiver. As the infant experiences trust that their needs will be met, their level of arousal diminishes, and they begin to develop the biological patterning of stress tolerance through emotional and physiological regulation. In the absence of caregiver nurturing, however, physiological arousal persists and the capacity for self-regulation fails to develop. This insecure attachment pattern can amount to emotional and behavioral problems, a fundamental distrust in others, and a pathway to psychopathology (Cooke et al., Citation2019). Emerging research suggests that attachment style also moderates an individual’s vulnerability for developing stress-related conditions. Whereas secure attachment exerts a protective effect (Turunen et al., Citation2014), insecure or disorganized attachment increases the risk of developing traumatic stress conditions following a life-threatening event (Besser & Neria, Citation2012).
The neural underpinnings of these behavioral attachment styles are complex and involve many of the systems described above. The amygdala, ANS, and reward system allow for protective hypervigilance as well as motivation and reward from relational attachment (Feldman, Citation2015). Oxytocin, produced in the hypothalamus, supports empathy, social connectedness and neuroplasticity for parental learning and bonding (Heinrichs et al., Citation2009). Cortical networks further contribute to mentalization, empathy, and emotion regulation (Feldman, Citation2015). Therefore, our hypothesis is that attachment issues could arise from different patterns of system dysregulation. For example, one person may struggle in relationships due to ANS dysregulation and a corresponding sense of fear and felt sense of lack of safety in relationships, whereas another person may have hypothalamic oxytocin dysregulation. Yet, a third person may have dysregulation in both systems as well as a disorganized cognitive system leading to struggles with top-down cortical emotion regulation. These different possibilities highlight the importance of considering attachment patterns as well as stress-related systems in the assessment and treatment of stress and trauma-related conditions.
Assessments
Although many clinicians may inquire about relational health, the assessment of social connection or attachment is not routinely performed outside research settings. The formal assessment of attachment style in children is intensive (Health UK, Citation2015). However, Hazan & Shaver (Citation1987) developed a simple tool for measuring attachment in adults called The Relationships Questionnaire (Bartholomew & Horowitz, Citation1991; Hazan & Shaver, Citation1987). Other tools that assess for relational health are available and may be used in clinical or research settings. These include the Berkman-Syme Social Network Index (Berkman & Syme, Citation1979), Loneliness Questionnaire (Ebesutani et al., Citation2012), Toronto Empathy Questionnaire (Spreng, Citation2009), Revised Dyadic Adjustment Scale (Busby et al., Citation1995), Medical Outcomes Study (MOS) Social Support Survey (Sherbourne & Stewart, Citation1991), and Convoy Circles of Support (Antonucci et al., Citation2014; Fuller et al., Citation2020).
In addition, the National Institutes of Health has developed a toolset that assesses emotional support, which can help identify people who may benefit from stronger social supports (NIH, Citation2022). The Neurosequential Model of Therapeutics provides a computer-based platform to assess relational health and adversity throughout the client/patient’s life as well as current neurologic and physiologic function (Perry, Citation2001, Citation2013, Citation2020; Perry & Dobson, Citation2013). Finally, potential biomarkers of attachment and relational health include oxytocin and vasopressin (Baracz et al., Citation2020; Heinrichs et al., Citation2009; Meyer-Lindenberg et al., Citation2011; Toepfer et al., Citation2017).
Interventions
Safe, supportive, nurturing relationships are foundational for healthy brain development and an essential part of healing after a stressful or traumatic experience (Center on the Developing Child at Harvard University, Citation2016; Garner & Yogman, Citation2021). Prevention of ELA by promoting safe stable nurturing relationships and environments is also critical for reducing risk for lifelong mental and physical health problems (Shonkoff et al., Citation2021; Shonkoff et al., Citation2012; Watson et al., 2023). When working with children with trauma or attachment wounding, it is essential to also work with caregivers. Supporting parents and caregivers with tools for self-regulation, parent education, and strengths-based approaches have been associated with increased parental warmth and attunement, decreased harsh or physical parenting practices, and the prevention of intergenerational transmission of adversity (Bellis et al., Citation2017; Bhushan et al., Citation2020; Jaffee et al., Citation2013; Marie-Mitchell & Kostolansky, Citation2019; Schofield et al., Citation2013).
Attachment patterns can heal, healthy relationship skills can be learned, and opportunities to develop trusting and safe relationships can be built over time (Bellis et al., Citation2017, Citation2018; Chiang et al., Citation2018). Individual therapy can help people build relational skills and be a model for a safe and trusting relationship. Moreover, psychoanalytic approaches including Attachment-based Therapy, Transference-focused Therapy, Interpersonal Psychotherapy, Internal Family Systems therapy, and mentalization-based treatments can provide a secure base, support the processing of past relational experiences, can be tailored to client attachment style and increase attachment security if properly used (Berry & Danquah, Citation2016; Lucero et al., Citation2018; Slade & Holmes, Citation2019). There are also many dyadic therapies that help to heal child-caregiver relationships, including Parent Child Interaction Therapy (Luby et al., Citation2020), Child Parent Psychotherapy (Lieberman et al., Citation2006; Lieberman et al., Citation2005), and Collaborative & Proactive Solutions (Greene & Winkler, Citation2019; Mulraney et al., Citation2022). For couples, Emotionally Focused Therapy & Integrative Behavioral Couples Therapy have been shown to reduce distress between couples (Doss et al., Citation2022). Recent research has also evaluated the use of oxytocin as a therapeutic intervention (Baldi et al., Citation2021). In addition, MDMA is known to increase oxytocin levels, interpersonal trust, and compassion for self and others and has been successfully used in MDMA-assisted psychotherapy to treat PTSD (Reiff et al., Citation2020).
Considerations for clinical practice and further research
Considered together, the literatures synthesized above provide the empirical basis for our Stress Phenotyping Framework, which we hope will enable researchers and clinician to better study, and intervene on, biological stress dynamics that harm health. In the clinical context, for example, if a patient with a history of ELA presenting with headaches and depression were to go to a traditional primary care clinician, the headaches would be treated with ibuprofen or a triptan, and the depression would be treated with an anti-depressant and referral to a mental health specialist. If this patient were to connect with a mental health therapist, the therapist would perform a symptoms-based, self-report assessment, diagnose using the DSM-V (a symptoms-based diagnosis model), and provide one or more of the treatment modalities for which they have received certification training. In contrast, use of our Stress Phenotyping Framework would encourage medical and mental health providers to assess across stress-related systems using self-report as well as health technology and biomarkers. Continuing the example introduced above, a provider may then learn that the patient has dysregulation in the following stress-related systems: (a) sensorimotor associated with hyper-sensitivity to pain and increased muscle tension, (b) ANS with reduced HRV, (c) HPA axis with a blunted diurnal cortisol curve, (d) immune system with elevated CRP levels, and (e) relational with an avoidant attachment style. Interventions that target specific biopsychosocial mechanisms could then be combined and layered in a modular fashion to target stress-related dysfunction in a multilevel and efficient manner.
The Stress Phenotyping Framework integrates and expands upon several approaches that have been put forth by clinical psychologists, psychiatrists, and neuroscientists including processed-based therapy (Hofmann & Hayes, Citation2019), the Neurosequential Model of Therapeutics (NMT) (Perry, Citation2006, Citation2009, Citation2020), brain plasticity-based therapeutics (Merzenich et al., Citation2014), and precision psychiatry (Mengelkoch et al., Citation2023; Williams, Citation2016). Process-based approaches aim to deconstruct manualized evidence-based cognitive behavioral therapies into their active ingredients to formularize personalized treatment plans for a given patient. Similarly, NMT, brain plasticity-based therapeutics, and Precision Psychiatry aim to provide personalized treatment plans by specifically targeting the underlying neurobiological disruption.
The Stress Phenotyping Framework builds on these concepts offering a highly integrative biopsychosocial approach that brings together the fields of medicine, mental health, neuroscience, and behavioral health to provide a shared, integrated, precision medicine approach to assessment, treatment, and research for stress-related conditions. Further, the Stress Phenotyping Framework provides a neurobiological and physiologic approach to improve mental and physical health care for conditions impacted by stress physiology such as anxiety, depression, suicidality, bipolar disorder, asthma, diabetes, obesity, chronic pain syndromes, substance misuse, and heart disease. For example, for the patient described above, a clinician could teach breathing and grounding techniques to regulate the ANS and discuss the possibility of adopting anti-inflammatory diets and taking supplements such as omega-3 fatty acids and curcumin to help normalize their stress biology. Using motivational interviewing and patient-centered care, the clinician could discuss additional evidence-based stress-mitigation strategies including ways to engage their natural support system, become more physically active, practice mindfulness and progressive muscle relaxation, and experience nature (Bhushan et al., Citation2020; Gilgoff et al., Citation2020, Citation2022).
Further discussions with the patient could address the sequencing of different psychosocial therapies or identifying a single therapy that addresses multiple dimensions. For example, deep brain reorienting may help resolve the muscle tension associated with ELA, although additional research is needed to evaluate whether it can regulate other stress-related systems. Additional, bottom-up approaches to consider include Somatic Experiencing, and Sensory Motor Arousal Regulation Therapy, EMDR, or HRV-based biofeedback. Finally, first-line psychotherapies such as CBT, Interpersonal Psychotherapy (IPT), or Psychodynamic psychotherapy may address some but not all of the stress-related systems. Therefore, we view the Stress Phenotyping Framework as critical for the integration of these additional treatment modalities into stress research, but also patient case conceptualizations and treatment plans.
A few key advancements must occur to move toward an effective process to diagnose and treat toxic stress. First, clinicians and researchers across disciplines must recognize the impact that stress, trauma, and adversity have on both mental and physical health. We need to move beyond a symptom-based approach and toward a multidisciplinary, stress-biology approach that identifies and treats underlying physiologic dysregulation in sensorimotor, arousal and energy, reward processing, ANS, HPA axis, endocrine, metabolic, immune, cognitive, and relational function. Each of these systems is a potential area for dysregulation, sensitization or tolerance, and an opportunity for targeted intervention (see and ).
Second, as stress biology awareness builds, we must advance our diagnostic tools. In addition to improving the reliability and validity of individual tools, much more research and clinical validation work needs to be done to streamline and simplify an assessment strategy that can assess all the key biopsychosocial systems involved while not overwhelming the patient or provider. Although we described many assessment tools and biomarkers here, we strongly recommend a shift toward challenge-based assessments of stress-related dysregulation to investigate their functional dynamics, as opposed to their basal, unchallenged state. Such assessments would be similar to a stress-exercise test performed by a cardiologist.
Third, although we mainly focused our intervention recommendations on those with a growing or solid evidence base, we also need to test additional innovative and scalable interventions that can improve stress-related dysregulation across systems. Finally, although those with access to healthcare and resources are already reaping the benefit of these innovative new treatment options, we must ensure that treatments are designed for, and made accessible to, those who have limited resources and healthcare access, but who would stand to benefit the most from these treatments. Moreover, policymakers must ensure that such assessments and interventions are covered benefits either through traditional fee-for-service health insurance policies or through value based care.
Conclusion
In conclusion, with increases in life expectancy over the past century, people today are much more likely to die from preventable, stress-related conditions such as suicide and depression, heart disease, and cancer than their counterparts were a hundred years ago. Indeed, nine out of ten deaths in the United States today are caused in part by stressors that disrupt social and biological functioning and lead to striking health disparities (Bhushan et al., Citation2020). Despite this fact, we presently do not have intervention solutions that are affordable, scalable, or effective enough to address this critical societal health problem.
Moreover, although there is a large literature demonstrating links between ELA, toxic stress, and poor health outcomes, there are no agreed-upon guidelines for diagnosing toxic stress, deploying therapeutic interventions, or evaluating treatment efficacy. In addition, current assessment tools and interventions are largely siloed without integration between mental health, physical health, and neuroscience. In mental health, diagnoses are based on symptoms rather than underlying neurobiology, and in clinical medicine, stress physiology is largely ignored.
Each of the systems described herein provides an opportunity for further research on potential therapeutic targets and measures of intervention efficacy to profoundly improve health outcomes. A stress biology approach can inform both “bottom up” and “top down” intervention approaches to get to the core: what works for whom and in what order (Perry, Citation2020; Perry & Dobson, Citation2013). It also explicitly calls out relational health and attachment as key elements of a comprehensive assessment and intervention approach to toxic stress. With the help of big data techniques such as artificial intelligence and machine learning, a stress biology approach provides a multidisciplinary path toward developing novel biomarkers for detecting toxic stress and identifying toxic stress phenotypes to improve predictive models of future disease risk (see ). This framework can also expand the therapeutic targets considered, and lead to prevention and intervention strategies that improve overall precision and efficacy in clinical care.
Box 1
Although stress behavior is often described as fight, flight, freeze, or affiliate, there is a broad heterogeneity in cellular-level responses and associated health outcomes. Below are additional multidisciplinary considerations and areas for further research that are beyond the scope of this review. The Stress Phenotyping Framework provides a comprehensive approach to evaluating how each of the factors below impact stress-related systems on a mechanistic level as well as providing clear next steps for prevention and treatment.
Developmental timing of stressor exposure matters. Adversity experienced at different stages of brain development will have different long-term impacts on later neurologic function (Agorastos et al., Citation2019; Perry, Citation2001, Citation2009; Perry & Hambrick, Citation2008; Teicher et al., Citation2022). (For an overview, see Nelson & Gabard-Durnam, Citation2020.)
Further exploring predictability, tolerance, and sensitization may help explain some of the discrepancies in stress research. Although predictable and controllable adverse events have been shown to have less severe impacts and are more likely to induce tolerance (i.e., higher “doses” of stress would be needed to activate the system), uncontrollable or unpredictable adversity may be more likely to lead to sensitization over time (i.e., lower “doses” of adversity can “trigger” the stress system) (Herman, Citation2013; Perry & Pollard, Citation1998; Radley & Herman, Citation2022). Indeed, whereas some studies have found that child maltreatment is associated with augmented HPA axis and autonomic responses, other studies have found blunted responses (Teicher et al., Citation2022).
The principles of neuroplasticity explain how chronic stressors and repetition of the stress response can further strengthen survival neural circuitry at the expense of other critical processes, such as executive functioning skills. (For a comprehensive review, see Merzenich et al., Citation2014.)
The type, duration, severity, and perception of stressors can influence which system is activated and how quickly the systems subsequently normalize. Although we all share the same basic components of the stress response, research suggests that different stress-related systems may get preferentially activated or deactivated based on aspects of the stressor itself, as well as how it is appraised (e.g., as a challenge vs. threat) (Kemeny, Citation2003).
It may be more helpful to frame the biological consequences of early life stress as adaptations rather than “damage” (Radley & Herman, Citation2022; Teicher et al., Citation2022). Chronic stress and ELA have been shown to confer long-term neurobiological adaptations with or without associated overt psychopathology, and that “resilient” asymptomatic individuals may be compensating through other neurobiological mechanisms (Ohashi et al., Citation2019; Teicher et al., Citation2022). The concepts of allostatic load and weathering have been used to describe this long-term wear and tear from chronic stress (Chae et al., Citation2020; Danese & McEwen, Citation2012; Shields & Slavich, Citation2017). (for an overview, see Radley & Herman, Citation2022.)
Research suggests that there are important sex differences in stress biology (Slavich & Sacher, Citation2019). For example, studies have found greater differences in corpus clausum and hippocampus in maltreated males vs. females (Teicher et al., Citation2022). (For a comprehensive review, see Bath, Citation2020.)
Studies find that connectivity patterns, biomarkers, and behaviors are dependent on the state of arousal a person is currently in when tested or tasked (Lanius et al., Citation2018; Perry et al., Citation1995; Young et al., Citation2018). These state-dependent patterns can become traits over time as they are practiced and neuroplastically embedded in neural connectivity patterns. (For a clinical review, see Perry et al., Citation1995.)
In addition to the above considerations, environmental and biological protective factors and predisposing vulnerabilities can contribute to the long-term impacts of ELA and chronic stress (Boyce, Citation2016; Epel et al., Citation2018; Garner & Yogman, Citation2021). (For a review of differential susceptibilities, see Boyce, Citation2016, and for a review of risk and protective factors for child maltreatment, see Austin et al., Citation2020.)
Box 2
Research on PTSD (vs. psychiatric control participants) is finding altered activity and connectivity within the Salience Network (SN) Default Mode Network (DMN), and Central Executive Network (CEN), demonstrating the interconnectedness across neural systems (Kearney & Lanius, Citation2022; Lanius et al., Citation2015). The SN detects salient stimuli including threats, facilitates switching between the DMN and CEN, and includes the amygdala, insula, dorsal anterior cingulate cortex. The DMN is active at rest, supports self-referential thinking, and includes the posterior cingulate cortex, ventromedial prefrontal cortex, and the medial temporal lobe (including hippocampus). The Central Executive Network is active during a task, supports cognitive control of emotions, and includes the dorsolateral prefrontal cortex (Akiki et al., Citation2017; Lanius et al., Citation2015; Menon, Citation2011; Zhang et al., Citation2022). PTSD is generally associated with increased activity and intrinsic connectivity within the SN associated with a low threshold for perceived saliency and difficulty modulating DMN and CEN activity. However, emerging research is finding that the dissociative subtype of PTSD is associated with decreased insula activation and SN activity along with impaired DMN activity associated with numbing, emotional detachment, depersonalization, and derealization (Lanius, Citation2018). This research further highlights the need to elucidate how the brain decides which system to activate in response to stimuli and the mechanisms by which it does so.
Acknowledgment
We thank Nicolette Ricker at Nicolette’s Eye for helping create the graphics in this article.
Disclosure statement
No potential conflicts of interest were reported by the authors.
Additional information
Funding
Notes on contributors
Rachel Gilgoff
Rachel Gilgoff, MD, is an adjunct Clinical Associate Professor at the Stanford University School of Medicine, an advisor with the California Aces Aware Initiative, and a clinician at GetzWell Personalized Pediatrics clinic in San Francisco. As a board-certified general pediatrician, child abuse pediatrician, and integrative medicine specialist, Dr. Gilgoff is dedicated to conducting translational research and providing patient-centered, individualized, multidisciplinary clinical care to address health issues resulting from child abuse and toxic stress.
Summer Mengelkoch
Summer Mengelkoch, PhD, is a is a postdoctoral fellow in the UCLA Laboratory for Stress Assessment and Research, within the Department of Psychiatry and Biobehavioral Sciences at the University of California, Los Angeles. Her research investigates the biological mechanisms through which stress experienced across the lifespan impacts health and behavior, with a focus on understanding stress-related processes in women.
Jorina Elbers
Jorina Elbers, MD, MS, is a pediatric neurologist with expertise in treating chronic stress and trauma. She was recently an Assistant Professor of Neurology at Stanford University and is currently the director for the Trauma Recovery Project at the HeartMath Institute, a 501(c)3 nonprofit. Her clinical research and practice have focused on nervous system dysregulation, and the evaluation and management of medical manifestations of trauma.
Krista Kotz
Krista Kotz, PhD, MPH, is an advisor and content developer for ACEs Aware, a California initiative to address Adverse Childhood Experiences in primary care. Her expertise includes educating clinicians about the physical and mental health impacts early life adversity and toxic stress, and how to mitigate those impacts.
Arielle Radin
Arielle Radin, PhD, is a health psychology researcher and the Co-Founder of Bruin Health, where she translates biopsychosocial research into intelligent technology systems with real-world clinical applications. Her work focuses on the integration of psychological and medical disciplines to create a holistic view of health.
Isha Pasumarthi
Isha Pasumarthi is currently completing a Master’s degree in Public Health at King’s College London, and is a research intern with the Sean N. Parker Center for Allergy and Asthma Research at Stanford University and the ACEs Aware Initiative at the UCLA-UCSF ACEs Aware Family Resilience Network. Her research focuses on preventing, identifying, and treating stress and stress-related health impacts, particularly through the use of biomarkers.
Reanna Murthy
Reanna Murthy, MPH, is currently a Clinical Research Coordinator at Partnerships for Research in Child Health, within the Division of General Pediatrics at the Stanford University School of Medicine. Her work with Stanford University’s Sean N. Parker Center for Allergy and Asthma Research has furthered her interest in the field of adverse childhood events and how it relates to physical and mental health in adulthood, particularly among first-generation immigrants in the United States.
Sayantani Sindher
Sayantani Sindher, MD, is a Clinical Associate Professor in the Department of Medicine at Stanford University and is the Director of the Clinical Translational Research Unit at the Sean N. Parker Center for Allery and Asthma Research. She is the principal investigator of several clinical trials assessing prevention, diagnosis, and novel therapeutic strategies for atopic conditions including food allergy, atopic dermatitis, and asthma.
Nadine Burke Harris
Nadine Burke Harris, MD, MPH, is a Senior Advisor to ACE Resource Network. She is a pediatrician specializing in treating the effects of stress and trauma in children’s developing brains and bodies, and has spent the past two decades translating research into best-practices for children and youth facing adversity. As California’s first Surgeon General, she led the implementation of the ACEs Aware initiative, which trained health care providers on best-practices for ACE screening and response with evidence-based, trauma-informed care.
George M. Slavich
George M. Slavich, PhD, is a Professor in the Department of Psychiatry and Biobehavioral Sciences at UCLA and a Research Scientist at the Semel Institute for Neuroscience and Human Behavior, where he directs the UCLA Laboratory for Stress Assessment and Research. He is a leading authority in the conceptualization, assessment, and management of life stress; in psychological and biological mechanisms linking stress with mental and physical health; and in systems and policies for reducing stress-related health disparities and achieving greater health equity.
References
- Afifi, T. D., Granger, D. A., Denes, A., Joseph, A., & Aldeis, D. (2011). Parents’ communication skills and adolescents’ salivary α-amylase and cortisol response patterns. Communication Monographs, 78(3), 1–34. https://doi.org/10.1080/03637751.2011.589460
- Agorastos, A., Nicolaides, N. C., Bozikas, V. P., Chrousos, G. P., & Pervanidou, P. (2020). Multilevel interactions of stress and circadian system: Implications for traumatic stress. Frontiers in Psychiatry, 10, 1003. https://doi.org/10.3389/fpsyt.2019.01003
- Agorastos, A., & Olff, M. (2021). Sleep, circadian system and traumatic stress. European Journal of Psychotraumatology, 12(1), 1956746. https://doi.org/10.1080/20008198.2021.1956746
- Agorastos, A., Pervanidou, P., Chrousos, G. P., & Baker, D. G. (2019). Developmental trajectories of early life stress and trauma: A narrative review on neurobiological aspects beyond stress system dysregulation. Frontiers in Psychiatry, 10, 118. https://doi.org/10.3389/fpsyt.2019.00118
- Ahmad, A. H., & Zakaria, R. (2015). Pain in times of stress. The Malaysian Journal of Medical Sciences : MJMS, 22(Spec Issue), 52–61.
- Ahmed, T., Qassem, M., & Kyriacou, P. A. (2023). Measuring stress: A review of the current cortisol and dehydroepiandrosterone (DHEA) measurement techniques and considerations for the future of mental health monitoring. Stress, 26(1), 29–42. https://doi.org/10.1080/10253890.2022.2164187
- Akiki, T. J., Averill, C. L., & Abdallah, C. G. (2017). A network-based neurobiological model of PTSD: Evidence from structural and functional neuroimaging studies. Current Psychiatry Reports, 19(11), 81. https://doi.org/10.1007/s11920-017-0840-4
- Akinsanya, A., Marwaha, R., & Tampi, R. R. (2017). Prazosin in children and adolescents with posttraumatic stress disorder who have nightmares: A systematic review. Journal of Clinical Psychopharmacology, 37(1), 84–88. https://doi.org/10.1097/JCP.0000000000000638
- Alghwiri, A. A., Whitney, S. L., Baker, C. E., Sparto, P. J., Marchetti, G. F., Rogers, J. C., & Furman, J. M. (2012). The development and validation of the vestibular activities and participation measure. Archives of Physical Medicine and Rehabilitation, 93(10), 1822–1831. https://doi.org/10.1016/j.apmr.2012.03.017
- Ali, N., & Nater, U. M. (2020). Salivary alpha-amylase as a biomarker of stress in behavioral medicine. International Journal of Behavioral Medicine, 27(3), 337–342. https://doi.org/10.1007/s12529-019-09843-x
- Amtmann, D., Cook, K. F., Jensen, M. P., Chen, W.-H., Choi, S., Revicki, D., Cella, D., Rothrock, N., Keefe, F., Callahan, L., & Lai, J.-S. (2010). Development of a PROMIS item bank to measure pain interference. Pain, 150(1), 173–182. https://doi.org/10.1016/j.pain.2010.04.025
- Antonucci, T. C., Ajrouch, K. J., & Birditt, K. S. (2014). The convoy model: Explaining social relations from a multidisciplinary perspective. The Gerontologist, 54(1), 82–92. https://doi.org/10.1093/geront/gnt118
- Arnsten, A. F. T. (2015). Stress weakens prefrontal networks: Molecular insults to higher cognition. Nature Neuroscience, 18(10), 1376–1385. https://doi.org/10.1038/nn.4087
- Arnsten, A. F. T., & Pliszka, S. R. (2011). Catecholamine influences on prefrontal cortical function: Relevance to treatment of attention deficit/hyperactivity disorder and related disorders. Pharmacology, Biochemistry, and Behavior, 99(2), 211–216. https://doi.org/10.1016/j.pbb.2011.01.020
- Austin, A. E., Lesak, A. M., & Shanahan, M. E. (2020). Risk and protective factors for child maltreatment: A review. Current Epidemiology Reports, 7(4), 334–342. https://doi.org/10.1007/s40471-020-00252-3
- Baik, J.-H. (2020). Stress and the dopaminergic reward system. Experimental & Molecular Medicine, 52(12), 1879–1890. https://doi.org/10.1038/s12276-020-00532-4
- Balban, M. Y., Neri, E., Kogon, M. M., Weed, L., Nouriani, B., Jo, B., Holl, G., Zeitzer, J. M., Spiegel, D., & Huberman, A. D. (2023). Brief structured respiration practices enhance mood and reduce physiological arousal. Cell Reports. Medicine, 4(1), 100895. https://doi.org/10.1016/j.xcrm.2022.100895
- Baldi, E., Costa, A., Rani, B., Passani, M. B., Blandina, P., Romano, A., & Provensi, G. (2021). Oxytocin and fear memory extinction: Possible implications for the therapy of fear disorders? International Journal of Molecular Sciences, 22(18), 10000. https://doi.org/10.3390/ijms221810000
- Baracz, S. J., Everett, N. A., & Cornish, J. L. (2020). The impact of early life stress on the central oxytocin system and susceptibility for drug addiction: Applicability of oxytocin as a pharmacotherapy. Neuroscience and Biobehavioral Reviews, 110, 114–132. https://doi.org/10.1016/j.neubiorev.2018.08.014
- Bartholomew, K., & Horowitz, L. M. (1991). Attachment styles among young adults: A test of a four-category model. Journal of Personality and Social Psychology, 61(2), 226–244. https://doi.org/10.1037//0022-3514.61.2.226
- Bath, K. G. (2020). Synthesizing views to understand sex differences in response to early life adversity. Trends in Neurosciences, 43(5), 300–310. https://doi.org/10.1016/j.tins.2020.02.004
- Bellis, M. A., Hardcastle, K., Ford, K., Hughes, K., Ashton, K., Quigg, Z., & Butler, N. (2017). Does continuous trusted adult support in childhood impart life-course resilience against adverse childhood experiences – a retrospective study on adult health-harming behaviours and mental well-being. BMC Psychiatry, 17(1), 110. https://doi.org/10.1186/s12888-017-1260-z
- Bellis, M. A., Hughes, K., Ford, K., Hardcastle, K. A., Sharp, C. A., Wood, S., Homolova, L., & Davies, A. (2018). Adverse childhood experiences and sources of childhood resilience: A retrospective study of their combined relationships with child health and educational attendance. BMC Public Health, 18(1), 792. https://doi.org/10.1186/s12889-018-5699-8
- Berens, A. E., Jensen, S. K. G., & Nelson, C. A. (2017). Biological embedding of childhood adversity: From physiological mechanisms to clinical implications. BMC Medicine, 15(1), 135. https://doi.org/10.1186/s12916-017-0895-4
- Berkman, L. F., & Syme, S. L. (1979). Social networks, host resistance, and mortality: A nine-year follow-up study of Alameda County residents. American Journal of Epidemiology, 109(2), 186–204. https://doi.org/10.1093/oxfordjournals.aje.a112674
- Berry, K., & Danquah, A. (2016). Attachment-informed therapy for adults: Towards a unifying perspective on practice. Psychology and Psychotherapy, 89(1), 15–32. https://doi.org/10.1111/papt.12063
- Berryhill, S., Morton, C. J., Dean, A., Berryhill, A., Provencio-Dean, N., Patel, S. I., Estep, L., Combs, D., Mashaqi, S., Gerald, L. B., Krishnan, J. A., & Parthasarathy, S. (2020). Effect of wearables on sleep in healthy individuals: A randomized crossover trial and validation study. Journal of Clinical Sleep Medicine: JCSM: Official Publication of the American Academy of Sleep Medicine, 16(5), 775–783. https://doi.org/10.5664/jcsm.8356
- Bersani, F. S., Mellon, S. H., Lindqvist, D., Kang, J. I., Rampersaud, R., Somvanshi, P. R., Doyle, F. J., Hammamieh, R., Jett, M., Yehuda, R., Marmar, C. R., & Wolkowitz, O. M. (2020). Novel pharmacological targets for combat PTSD—Metabolism, inflammation, the gut microbiome, and mitochondrial dysfunction. Military Medicine, 185(Suppl 1), 311–318. https://doi.org/10.1093/milmed/usz260
- Besser, A., & Neria, Y. (2012). When home isn’t a safe haven: Insecure attachment orientations, perceived social support, and PTSD symptoms among Israeli evacuees under missile threat. Psychological Trauma: Theory, Research, Practice, and Policy, 4(1), 34–46. https://doi.org/10.1037/a0017835
- Bhushan, D., Kotz, K., McCall, J., Wirtz, S., Gilgoff, R., Dube, S. R., Powers, C., Olson-Morgan, J., Galeste, M., Patterson, K., Harris, L., Mills, A., Bethell, C., & Burke Harris, N. (2020). Roadmap for resilience: The California surgeon general’s report on adverse childhood experiences, toxic stress, and health. Office of the California Surgeon General. https://osg.ca.gov/sg-report/
- Birnie, M. T., Kooiker, C. L., Short, A. K., Bolton, J. L., Chen, Y., & Baram, T. Z. (2020). Plasticity of the reward circuitry after early-life adversity: Mechanisms and significance. Biological Psychiatry, 87(10), 875–884. https://doi.org/10.1016/j.biopsych.2019.12.018
- Black, M. H., Milbourn, B., Chen, N. T. M., McGarry, S., Wali, F., Ho, A. S. V., Lee, M., Bölte, S., Falkmer, T., & Girdler, S. (2020). The use of wearable technology to measure and support abilities, disabilities and functional skills in autistic youth: A scoping review. Scandinavian Journal of Child and Adolescent Psychiatry and Psychology, 8(1), 48–69. https://doi.org/10.21307/sjcapp-2020-006
- Black, D. S., & Slavich, G. M. (2016). Mindfulness meditation and the immune system: A systematic review of randomized controlled trials. Annals of the New York Academy of Sciences, 1373(1), 13–24. https://doi.org/10.1111/nyas.12998
- Blume, C., Garbazza, C., & Spitschan, M. (2019). Effects of light on human circadian rhythms, sleep and mood. Somnologie: Schlafforschung Und Schlafmedizin = Somnology: Sleep Research and Sleep Medicine, 23(3), 147–156. https://doi.org/10.1007/s11818-019-00215-x
- Bohus, M., Kleindienst, N., Hahn, C., Müller-Engelmann, M., Ludäscher, P., Steil, R., Fydrich, T., Kuehner, C., Resick, P. A., Stiglmayr, C., Schmahl, C., & Priebe, K. (2020). Dialectical behavior therapy for posttraumatic stress disorder (DBT-PTSD) compared with cognitive processing therapy (CPT) in complex presentations of PTSD in women survivors of childhood abuse: A randomized clinical trial. JAMA Psychiatry, 77(12), 1235–1245. https://doi.org/10.1001/jamapsychiatry.2020.2148
- Bower, J. E., Kuhlman, K. R., Haydon, M. D., Boyle, C. C., & Radin, A. (2019). Cultivating a healthy neuro-immune network: A health psychology approach. Social and Personality Psychology Compass, 13(9), e12498. https://doi.org/10.1111/spc3.12498
- Boyce, W. T. (2016). Differential susceptibility of the developing brain to contextual adversity and stress. Neuropsychopharmacology: Official Publication of the American College of Neuropsychopharmacology, 41(1), 142–162. https://doi.org/10.1038/npp.2015.294
- Boyle, G. J. (1987). A cross-validation of the factor structure of the profile of mood states: Were the factors correctly identified in the first instance? Psychological Reports, 60(2), 343–354. https://doi.org/10.2466/pr0.1987.60.2.343
- Bradley, M. M. (2009). Natural selective attention: Orienting and emotion. Psychophysiology, 46(1), 1–11. https://doi.org/10.1111/j.1469-8986.2008.00702.x
- Bradley, M. M., & Lang, P. J. (1994). Measuring emotion: The self-assessment manikin and the semantic differential. Journal of Behavior Therapy and Experimental Psychiatry, 25(1), 49–59. https://doi.org/10.1016/0005-7916(94)90063-9.
- Brady, R. G., Rogers, C. E., Prochaska, T., Kaplan, S., Lean, R. E., Smyser, T. A., Shimony, J. S., Slavich, G. M., Warner, B. B., Barch, D. M., Luby, J. L., & Smyser, C. D. (2022). The effects of prenatal exposure to neighborhood crime on neonatal functional connectivity. Biological Psychiatry, 92(2), 139–148. https://doi.org/10.1016/j.biopsych.2022.01.020
- Briguglio, M., Vitale, J. A., Galentino, R., Banfi, G., Zanaboni Dina, C., Bona, A., Panzica, G., Porta, M., Dell’Osso, B., & Glick, I. D. (2020). Healthy eating, physical activity, and sleep hygiene (HEPAS) as the winning triad for sustaining physical and mental health in patients at risk for or with neuropsychiatric disorders: Considerations for clinical practice. Neuropsychiatric Disease and Treatment, 16, 55–70. https://doi.org/10.2147/ndt.S229206
- Brock, M. S., Powell, T. A., Creamer, J. L., Moore, B. A., & Mysliwiec, V. (2019). Trauma associated sleep disorder: Clinical developments 5 years after discovery. Current Psychiatry Reports, 21(9), 80. https://doi.org/10.1007/s11920-019-1066-4
- Brown, T., Almiento, L., Yu, M.-L., & Bhopti, A. (2023). The sensory processing measure – second edition: A critical review and appraisal. Occupational Therapy in Health Care, 1–34. https://doi.org/10.1080/07380577.2023.2280216
- Brown, D. W., Anda, R. F., Tiemeier, H., Felitti, V. J., Edwards, V. J., Croft, J. B., & Giles, W. H. (2009). Adverse childhood experiences and the risk of premature mortality. American Journal of Preventive Medicine, 37(5), 389–396. https://doi.org/10.1016/j.amepre.2009.06.021
- Bucci, M., Marques, S. S., Oh, D., & Harris, N. B. (2016). Toxic stress in children and adolescents. Advances in Pediatrics, 63(1), 403–428. https://doi.org/10.1016/j.yapd.2016.04.002
- Burke, N. N., Finn, D. P., McGuire, B. E., & Roche, M. (2017). Psychological stress in early life as a predisposing factor for the development of chronic pain: Clinical and preclinical evidence and neurobiological mechanisms. Journal of Neuroscience Research, 95(6), 1257–1270. https://doi.org/10.1002/jnr.23802
- Burke, N. J., Hellman, J. L., Scott, B. G., Weems, C. F., & Carrion, V. G. (2011). The impact of adverse childhood experiences on an urban pediatric population. Child Abuse & Neglect, 35(6), 408–413. https://doi.org/10.1016/j.chiabu.2011.02.006
- Busby, D. M., Christensen, C., Crane, D. R., & Larson, J. H. (1995). A revision of the dyadic adjustment scale for use with distressed and nondistressed couples: Construct hierarchy and multidimensional scales. Journal of Marital and Family Therapy, 21(3), 289–308. https://doi.org/10.1111/j.1752-0606.1995.tb00163.x
- Carlezon, W. A., & Krystal, A. D. (2016). Kappa-opioid antagonists for psychiatric disorders: From bench to clinical trials. Depression and Anxiety, 33(10), 895–906. https://doi.org/10.1002/da.22500
- Carpenter, L. L., Gawuga, C. E., Tyrka, A. R., Lee, J. K., Anderson, G. M., & Price, L. H. (2010). Association between plasma IL-6 response to acute stress and early-life adversity in healthy adults. Neuropsychopharmacology: Official Publication of the American College of Neuropsychopharmacology, 35(13), 2617–2623. https://doi.org/10.1038/npp.2010.159
- Caumo, W., Hidalgo, M. P., Souza, A., Torres, I. L. S., & Antunes, L. C. (2019). Melatonin is a biomarker of circadian dysregulation and is correlated with major depression and fibromyalgia symptom severity. Journal of Pain Research, 12, 545–556. https://doi.org/10.2147/JPR.S176857
- Center on the Developing Child at Harvard University. (2016). From best practices to breakthrough impacts: A science-based approach to building a more promising future for young children and families. Retrieved from www.developingchild.harvard.edu
- Chae, D. H., Wang, Y., Martz, C. D., Slopen, N., Yip, T., Adler, N. E., Fuller-Rowell, T. E., Lin, J., Matthews, K. A., Brody, G. H., Spears, E. C., Puterman, E., & Epel, E. S. (2020). Racial discrimination and telomere shortening among African Americans: The Coronary Artery Risk Development in Young Adults (CARDIA) Study. Health Psychology: Official Journal of the Division of Health Psychology, American Psychological Association, 39(3), 209–219. https://doi.org/10.1037/hea0000832
- Chellappa, S. L., & Aeschbach, D. (2022). Sleep and anxiety: From mechanisms to interventions. Sleep Medicine Reviews, 61, 101583. https://doi.org/10.1016/j.smrv.2021.101583
- Chen, E., Shalowitz, M. U., Story, R. E., Ehrlich, K. B., Manczak, E. M., Ham, P. J., Le, V., & Miller, G. E. (2017). Parents’ childhood socioeconomic circumstances are associated with their children’s asthma outcomes. The Journal of Allergy and Clinical Immunology, 140(3), 828–835.e2. https://doi.org/10.1016/j.jaci.2016.11.040
- Chen, E., Shalowitz, M. U., Story, R. E., Hayen, R., Leigh, A. K. K., Hoffer, L. C., Austin, M. K., Lam, P. H., Brody, G. H., & Miller, G. E. (2019). The costs of high self-control in black and Latino youth with asthma: Divergence of mental health and inflammatory profiles. Brain, Behavior, and Immunity, 80, 120–128. https://doi.org/10.1016/j.bbi.2019.02.031
- Cheshire, W. P., Freeman, R., Gibbons, C. H., Cortelli, P., Wenning, G. K., Hilz, M. J., Spies, J. M., Lipp, A., Sandroni, P., Wada, N., Mano, A., Ah Kim, H., Kimpinski, K., Iodice, V., Idiáquez, J., Thaisetthawatkul, P., Coon, E. A., Low, P. A., & Singer, W. (2021). Electrodiagnostic assessment of the autonomic nervous system: A consensus statement endorsed by the American Autonomic Society, American Academy of Neurology, and the International Federation of Clinical Neurophysiology. Clinical Neurophysiology: Official Journal of the International Federation of Clinical Neurophysiology, 132(2), 666–682. https://doi.org/10.1016/j.clinph.2020.11.024
- Chiang, J. J., Chen, E., & Miller, G. E. (2018). Midlife self-reported social support as a buffer against premature mortality risks associated with childhood abuse. Nature Human Behaviour, 2(4), 261–268. https://doi.org/10.1038/s41562-018-0316-5
- Chida, Y., Sudo, N., Sonoda, J., Hiramoto, T., & Kubo, C. (2007). Early-life psychological stress exacerbates adult mouse asthma via the hypothalamus-pituitary-adrenal axis. American Journal of Respiratory and Critical Care Medicine, 175(4), 316–322. https://doi.org/10.1164/rccm.200607-898OC
- Chung, F., Abdullah, H. R., & Liao, P. (2016). STOP-bang questionnaire: A practical approach to screen for obstructive sleep apnea. Chest, 149(3), 631–638. https://doi.org/10.1378/chest.15-0903
- Conway, S. M., Mikati, M. O., & Al-Hasani, R. (2022). Challenges and new opportunities for detecting endogenous opioid peptides in reward. Addiction Neuroscience, 2, 100016. https://doi.org/10.1016/j.addicn.2022.100016
- Cooke, J. E., Kochendorfer, L. B., Stuart-Parrigon, K. L., Koehn, A. J., & Kerns, K. A. (2019). Parent-child attachment and children’s experience and regulation of emotion: A meta-analytic review. Emotion (Washington, D.C.), 19(6), 1103–1126. https://doi.org/10.1037/emo0000504
- Craske, M. G., Meuret, A. E., Echiverri-Cohen, A., Rosenfield, D., & Ritz, T. (2023). Positive affect treatment targets reward sensitivity: A randomized controlled trial. Journal of Consulting and Clinical Psychology, 91(6), 350–366. https://doi.org/10.1037/ccp0000805
- Danese, A., & J Lewis, S. (2017). Psychoneuroimmunology of early-life stress: The hidden wounds of childhood trauma? Neuropsychopharmacology: Official Publication of the American College of Neuropsychopharmacology, 42(1), 99–114. https://doi.org/10.1038/npp.2016.198
- Danese, A., & McEwen, B. S. (2012). Adverse childhood experiences, allostasis, allostatic load, and age-related disease. Physiology & Behavior, 106(1), 29–39. https://doi.org/10.1016/j.physbeh.2011.08.019
- Danese, A., Pariante, C. M., Caspi, A., Taylor, A., & Poulton, R. (2007). Childhood maltreatment predicts adult inflammation in a life-course study. Proceedings of the National Academy of Sciences of the United States of America, 104(4), 1319–1324. https://doi.org/10.1073/pnas.0610362104
- Davies, P. L., & Gavin, W. J. (2007). Validating the diagnosis of sensory processing disorders using EEG technology. The American Journal of Occupational Therapy: Official Publication of the American Occupational Therapy Association, 61(2), 176–189. https://doi.org/10.5014/ajot.61.2.176
- de Arellano, M. A. R., Lyman, D. R., Jobe-Shields, L., George, P., Dougherty, R. H., Daniels, A. S., Ghose, S. S., Huang, L., & Delphin-Rittmon, M. E. (2014). Trauma-focused cognitive behavioral therapy: Assessing the evidence. Psychiatric Services (Washington, D.C.), 65(5), 591–602. https://doi.org/10.1176/appi.ps.201300255
- De Berardis, D., Marini, S., Serroni, N., Iasevoli, F., Tomasetti, C., de Bartolomeis, A., Mazza, M., Tempesta, D., Valchera, A., Fornaro, M., Pompili, M., Sepede, G., Vellante, F., Orsolini, L., Martinotti, G., & Di Giannantonio, M. (2015). Targeting the noradrenergic system in posttraumatic stress disorder: A systematic review and meta-analysis of prazosin trials. Current Drug Targets, 16(10), 1094–1106. https://doi.org/10.2174/1389450116666150506114108
- Dean, E., Dunn, W., & Little, L. (2016). Validity of the sensory profile 2: A confirmatory factor analysis. The American Journal of Occupational Therapy, 70(4_Supplement_1), 7011500075p1–7011500075p1. https://doi.org/10.5014/ajot.2016.70S1-PO7054
- Deighton, S., Neville, A., Pusch, D., & Dobson, K. (2018). Biomarkers of adverse childhood experiences: A scoping review. Psychiatry Research, 269, 719–732. https://doi.org/10.1016/j.psychres.2018.08.097
- D’Esposito, M., & Postle, B. R. (2015). The cognitive neuroscience of working memory. Annual Review of Psychology, 66(1), 115–142. https://doi.org/10.1146/annurev-psych-010814-015031
- Dias, B. G., & Ressler, K. J. (2014). Parental olfactory experience influences behavior and neural structure in subsequent generations. Nature Neuroscience, 17(1), 89–96. https://doi.org/10.1038/nn.3594
- Djuric, Z., Bird, C. E., Furumoto-Dawson, A., Rauscher, G. H., Ruffin, M. T., Stowe, R. P., Tucker, K. L., & Masi, C. M. (2008). Biomarkers of psychological stress in health disparities research. The Open Biomarkers Journal, 1(1), 7–19. https://doi.org/10.2174/1875318300801010007
- Dolder, P. C., Müller, F., Schmid, Y., Borgwardt, S. J., & Liechti, M. E. (2018). Direct comparison of the acute subjective, emotional, autonomic, and endocrine effects of MDMA, methylphenidate, and modafinil in healthy subjects. Psychopharmacology, 235(2), 467–479. https://doi.org/10.1007/s00213-017-4650-5
- Doss, B. D., Roddy, M. K., Wiebe, S. A., & Johnson, S. M. (2022). A review of the research during 2010–2019 on evidence-based treatments for couple relationship distress. Journal of Marital and Family Therapy, 48(1), 283–306. https://doi.org/10.1111/jmft.12552
- Dragioti, E., Li, H., Tsitsas, G., Lee, K. H., Choi, J., Kim, J., Choi, Y. J., Tsamakis, K., Estradé, A., Agorastos, A., Vancampfort, D., Tsiptsios, D., Thompson, T., Mosina, A., Vakadaris, G., Fusar-Poli, P., Carvalho, A. F., Correll, C. U., Han, Y. J., … Solmi, M. (2022). A large-scale meta-analytic atlas of mental health problems prevalence during the COVID-19 early pandemic. Journal of Medical Virology, 94(5), 1935–1949. https://doi.org/10.1002/jmv.27549
- Drozdz, S. J., Goel, A., McGarr, M. W., Katz, J., Ritvo, P., Mattina, G. F., Bhat, V., Diep, C., & Ladha, K. S. (2022). Ketamine assisted psychotherapy: A systematic narrative review of the literature. Journal of Pain Research, 15, 1691–1706. https://doi.org/10.2147/JPR.S360733
- Duffy, K. A., McLaughlin, K. A., & Green, P. A. (2018). Early life adversity and health-risk behaviors: Proposed psychological and neural mechanisms. Annals of the New York Academy of Sciences, 1428(1), 151–169. https://doi.org/10.1111/nyas.13928
- Dunn, W. (1999). Sensory profile, PsycTESTS Dataset [Preprint].
- Dutcher, J. M. (2023). Brain reward circuits promote stress resilience and health: Implications for reward-based interventions. Current Directions in Psychological Science, 32(1), 65–72. https://doi.org/10.1177/09637214221121770
- Ebesutani, C., Drescher, C. F., Reise, S. P., Heiden, L., Hight, T. L., Damon, J. D., & Young, J. (2012). The loneliness questionnaire–short version: An evaluation of reverse-worded and non-reverse-worded items via item response theory. Journal of Personality Assessment, 94(4), 427–437. https://doi.org/10.1080/00223891.2012.662188
- Eisenberger, N. I. (2012). The pain of social disconnection: Examining the shared neural underpinnings of physical and social pain. Nature Reviews. Neuroscience, 13(6), 421–434. https://doi.org/10.1038/nrn3231
- Eisenberger, N. I., & Cole, S. W. (2012). Social neuroscience and health: Neurophysiological mechanisms linking social ties with physical health. Nature Neuroscience, 15(5), 669–674. https://doi.org/10.1038/nn.3086
- Elbers, J., Jaradeh, S., Yeh, A. M., & Golianu, B. (2018). Wired for threat: Clinical features of nervous system dysregulation in 80 children. Pediatric Neurology, 89, 39–48. https://doi.org/10.1016/j.pediatrneurol.2018.07.007
- Elwenspoek, M. M. C., Kuehn, A., Muller, C. P., & Turner, J. D. (2017). The effects of early life adversity on the immune system. Psychoneuroendocrinology, 82, 140–154. https://doi.org/10.1016/j.psyneuen.2017.05.012
- Entringer, S., Buss, C., & Wadhwa, P. D. (2012). Prenatal stress, telomere biology, and fetal programming of health and disease risk. Science Signaling, 5(248), pt12. https://doi.org/10.1126/scisignal.2003580
- Entringer, S., Buss, C., & Wadhwa, P. D. (2015). Prenatal stress, development, health and disease risk: A psychobiological perspective-2015 Curt Richter Award Paper. Psychoneuroendocrinology, 62, 366–375. https://doi.org/10.1016/j.psyneuen.2015.08.019
- Epel, E. S., Crosswell, A. D., Mayer, S. E., Prather, A. A., Slavich, G. M., Puterman, E., & Mendes, W. B. (2018). More than a feeling: A unified view of stress measurement for population science. Frontiers in Neuroendocrinology, 49, 146–169. https://doi.org/10.1016/j.yfrne.2018.03.001
- Erickson, K. I., Voss, M. W., Prakash, R. S., Basak, C., Szabo, A., Chaddock, L., Kim, J. S., Heo, S., Alves, H., White, S. M., Wojcicki, T. R., Mailey, E., Vieira, V. J., Martin, S. A., Pence, B. D., Woods, J. A., McAuley, E., & Kramer, A. F. (2011). Exercise training increases size of hippocampus and improves memory. Proceedings of the National Academy of Sciences of the United States of America, 108(7), 3017–3022. https://doi.org/10.1073/pnas.1015950108
- Fabbri, M., Beracci, A., Martoni, M., Meneo, D., Tonetti, L., & Natale, V. (2021). Measuring subjective sleep quality: A review. International Journal of Environmental Research and Public Health, 18(3), 1082. https://doi.org/10.3390/ijerph18031082
- Feldman, R. (2015). The adaptive human parental brain: Implications for children’s social development. Trends in Neurosciences, 38(6), 387–399. https://doi.org/10.1016/j.tins.2015.04.004
- Fenster, R. J., Lebois, L. A. M., Ressler, K. J., & Suh, J. (2018). Brain circuit dysfunction in post-traumatic stress disorder: From mouse to man. Nature Reviews. Neuroscience, 19(9), 535–551. https://doi.org/10.1038/s41583-018-0039-7
- Firth, J., Stubbs, B., Vancampfort, D., Schuch, F., Lagopoulos, J., Rosenbaum, S., & Ward, P. B. (2018). Effect of aerobic exercise on hippocampal volume in humans: A systematic review and meta-analysis. NeuroImage, 166, 230–238. https://doi.org/10.1016/j.neuroimage.2017.11.007
- Fisher, J. (2019). Sensorimotor psychotherapy in the treatment of trauma. Practice Innovations, 4(3), 156–165. https://doi.org/10.1037/pri0000096
- Fisher, P. A., & Berkman, E. T. (2015). Designing interventions informed by scientific knowledge about effects of early adversity: A translational neuroscience agenda for next-generation addictions research. Current Addiction Reports, 2(4), 347–353. https://doi.org/10.1007/s40429-015-0071-x
- Fournié, C., Chouchou, F., Dalleau, G., Caderby, T., Cabrera, Q., & Verkindt, C. (2021). Heart rate variability biofeedback in chronic disease management: A systematic review. Complementary Therapies in Medicine, 60, 102750. https://doi.org/10.1016/j.ctim.2021.102750
- Fuligni, A. J., Chiang, J. J., & Tottenham, N. (2021). Sleep disturbance and the long-term impact of early adversity. Neuroscience and Biobehavioral Reviews, 126, 304–313. https://doi.org/10.1016/j.neubiorev.2021.03.021
- Fuller, H. R., Ajrouch, K. J., & Antonucci, T. C. (2020). The convoy model and later-life family relationships. Journal of Family Theory & Review, 12(2), 126–146. https://doi.org/10.1111/jftr.12376
- Furman, D., Campisi, J., Verdin, E., Carrera-Bastos, P., Targ, S., Franceschi, C., Ferrucci, L., Gilroy, D. W., Fasano, A., Miller, G. W., Miller, A. H., Mantovani, A., Weyand, C. M., Barzilai, N., Goronzy, J. J., Rando, T. A., Effros, R. B., Lucia, A., Kleinstreuer, N., & Slavich, G. M. (2019). Chronic inflammation in the etiology of disease across the life span. Nature Medicine, 25(12), 1822–1832. https://doi.org/10.1038/s41591-019-0675-0
- Garland, E. L. (2020). Psychosocial intervention and the reward system in pain and opioid misuse: New opportunities and directions. Pain, 161(12), 2659–2666. https://doi.org/10.1097/j.pain.0000000000001988
- Garner, A., & Yogman, M. (2021). Preventing childhood toxic stress: Partnering with families and communities to promote relational health. Pediatrics, 148(2), e2021052582. https://doi.org/10.1542/peds.2021-052582
- George, K. C., Kebejian, L., Ruth, L. J., Miller, C. W. T., & Himelhoch, S. (2016). Meta-analysis of the efficacy and safety of prazosin versus placebo for the treatment of nightmares and sleep disturbances in adults with posttraumatic stress disorder. Journal of Trauma & Dissociation: The Official Journal of the International Society for the Study of Dissociation (ISSD), 17(4), 494–510. https://doi.org/10.1080/15299732.2016.1141150
- Gilgoff, R., Schwartz, T., Owen, M., Bhushan, D., & Burke Harris, N. (2022). Opportunities to treat toxic stress. Pediatrics, 151(1), e2021055591. https://doi.org/10.1542/peds.2021-055591
- Gilgoff, R., Singh, L., Koita, K., Gentile, B., & Marques, S. S. (2020). Adverse childhood experiences, outcomes, and interventions. Pediatric Clinics of North America, 67(2), 259–273. https://doi.org/10.1016/j.pcl.2019.12.001
- Glaser, R., & Kiecolt-Glaser, J. K. (2005). Stress-induced immune dysfunction: Implications for health. Nature Reviews. Immunology, 5(3), 243–251. https://doi.org/10.1038/nri1571
- Gleeson, M., Bishop, N. C., Stensel, D. J., Lindley, M. R., Mastana, S. S., & Nimmo, M. A. (2011). The anti-inflammatory effects of exercise: Mechanisms and implications for the prevention and treatment of disease. Nature Reviews. Immunology, 11(9), 607–615. https://doi.org/10.1038/nri3041
- Greene, R., & Winkler, J. (2019). Collaborative & proactive solutions (CPS): A review of research findings in families, schools, and treatment facilities. Clinical Child and Family Psychology Review, 22(4), 549–561. https://doi.org/10.1007/s10567-019-00295-z
- Greenfield, E. A., Lee, C., Friedman, E. L., & Springer, K. W. (2011). Childhood abuse as a risk factor for sleep problems in adulthood: Evidence from a US national study. Annals of Behavioral Medicine: A Publication of the Society of Behavioral Medicine, 42(2), 245–256. https://doi.org/10.1007/s12160-011-9285-x
- Greer, S. M., Trujillo, A. J., Glover, G. H., & Knutson, B. (2014). Control of nucleus accumbens activity with neurofeedback. NeuroImage, 96, 237–244. https://doi.org/10.1016/j.neuroimage.2014.03.073
- Grégoire, J.-M., Gilon, C., Carlier, S., & Bersini, H. (2023). Autonomic nervous system assessment using heart rate variability. Acta Cardiologica, 78(6), 648–662. https://doi.org/10.1080/00015385.2023.2177371
- Grewen, K. M., Girdler, S. S., Amico, J., & Light, K. C. (2005). Effects of partner support on resting oxytocin, cortisol, norepinephrine, and blood pressure before and after warm partner contact. Psychosomatic Medicine, 67(4), 531–538. https://doi.org/10.1097/01.psy.0000170341.88395.47
- Gualtieri, C. T., & Johnson, L. G. (2006). Reliability and validity of a computerized neurocognitive test battery, CNS Vital Signs. Archives of Clinical Neuropsychology: The Official Journal of the National Academy of Neuropsychologists, 21(7), 623–643. https://doi.org/10.1016/j.acn.2006.05.007
- Hanson, J. L., Williams, A. V., Bangasser, D. A., & Peña, C. J. (2021). Impact of early life stress on reward circuit function and regulation. Frontiers in Psychiatry, 12, 744690. https://doi.org/10.3389/fpsyt.2021.744690
- Harb, H., & Renz, H. (2015). Update on epigenetics in allergic disease. The Journal of Allergy and Clinical Immunology, 135(1), 15–24. https://doi.org/10.1016/j.jaci.2014.11.009
- Hazan, C., & Shaver, P. (1987). Romantic love conceptualized as an attachment process. Journal of Personality and Social Psychology, 52(3), 511–524. https://doi.org/10.1037/0022-3514.52.3.511
- Health (UK), N.C.C. for M. (2015). Identification and assessment of attachment difficulties. In Children’s attachment: Attachment in children and young people who are adopted from care, in care or at high risk of going into care. National Institute for Health and Care Excellence (NICE). https://www.ncbi.nlm.nih.gov/books/NBK356191/ (Accessed: October 15, 2023).
- Heim, C., Wagner, D., Maloney, E., Papanicolaou, D. A., Solomon, L., Jones, J. F., Unger, E. R., & Reeves, W. C. (2006). Early adverse experience and risk for chronic fatigue syndrome: Results from a population-based study. Archives of General Psychiatry, 63(11), 1258–1266. https://doi.org/10.1001/archpsyc.63.11.1258
- Heim, C. M., Entringer, S., & Buss, C. (2019). Translating basic research knowledge on the biological embedding of early-life stress into novel approaches for the developmental programming of lifelong health. Psychoneuroendocrinology, 105, 123–137. https://doi.org/10.1016/j.psyneuen.2018.12.011
- Heinrichs, M., von Dawans, B., & Domes, G. (2009). Oxytocin, vasopressin, and human social behavior. Frontiers in Neuroendocrinology, 30(4), 548–557. https://doi.org/10.1016/j.yfrne.2009.05.005
- Hendrikse, C. J., Du Plessis, S., Luckhoff, H. K., Vink, M., van den Heuvel, L. L., Scheffler, F., Phahladira, L., Smit, R., Asmal, L., Seedat, S., & Emsley, R. (2022). Childhood trauma exposure and reward processing in healthy adults: A functional neuroimaging study. Journal of Neuroscience Research, 100(7), 1452–1462. https://doi.org/10.1002/jnr.25051
- Herman, J. P. (2013). Neural control of chronic stress adaptation. Frontiers in Behavioral Neuroscience, 7, 61. https://doi.org/10.3389/fnbeh.2013.00061
- Herman, J. P., et al. (2016). Regulation of the hypothalamic-pituitary-adrenocortical stress response. Comprehensive Physiology, 6(2), 603–621. https://doi.org/10.1002/cphy.c150015
- Hermans, E. J., Henckens, M. J. A. G., Joëls, M., & Fernández, G. (2014). Dynamic adaptation of large-scale brain networks in response to acute stressors. Trends in Neurosciences, 37(6), 304–314. https://doi.org/10.1016/j.tins.2014.03.006
- Herzberg, M. P., & Gunnar, M. R. (2020). Early life stress and brain function: Activity and connectivity associated with processing emotion and reward. NeuroImage, 209, 116493. https://doi.org/10.1016/j.neuroimage.2019.116493
- Hewson-Bower, B., & Drummond, P. D. (2001). Psychological treatment for recurrent symptoms of colds and flu in children. Journal of Psychosomatic Research, 51(1), 369–377. https://doi.org/10.1016/s0022-3999(01)00212-4.
- Hill, P. L., Turiano, N. A., & Burrow, A. L. (2018). Early life adversity as a predictor of sense of purpose during adulthood. International Journal of Behavioral Development, 42(1), 143–147. https://doi.org/10.1177/0165025416681537
- Hillard, C. J. (2018). Circulating endocannabinoids: From whence do they come and where are they going? Neuropsychopharmacology: Official Publication of the American College of Neuropsychopharmacology, 43(1), 155–172. https://doi.org/10.1038/npp.2017.130
- Hodkinson, H. M. (1972). Evaluation of a mental test score for assessment of mental impairment in the elderly. Age and Ageing, 1(4), 233–238. https://doi.org/10.1093/ageing/1.4.233
- Hofmann, S. G., & Hayes, S. C. (2019). The future of intervention science: Process-based therapy. Clinical Psychological Science: A Journal of the Association for Psychological Science, 7(1), 37–50. https://doi.org/10.1177/2167702618772296
- Holt-Lunstad, J., Smith, T. B., & Layton, J. B. (2010). Social relationships and mortality risk: A meta-analytic review. PLoS Medicine, 7(7), e1000316. https://doi.org/10.1371/journal.pmed.1000316
- Horn, S. R., Leve, L. D., Levitt, P., & Fisher, P. A. (2019). Childhood adversity, mental health, and oxidative stress: A pilot study. PloS One, 14(4), e0215085. https://doi.org/10.1371/journal.pone.0215085
- Horne, J. A., & Ostberg, O. (1976). A self-assessment questionnaire to determine morningness-eveningness in human circadian rhythms. International Journal of Chronobiology, 4(2), 97–110.
- Hughes, K., Bellis, M. A., Hardcastle, K. A., Sethi, D., Butchart, A., Mikton, C., Jones, L., & Dunne, M. P. (2017). The effect of multiple adverse childhood experiences on health: A systematic review and meta-analysis. The Lancet Public Health, 2(8), e356–e366. https://doi.org/10.1016/S2468-2667(17)30118-4
- Irwin, M. R., Olmstead, R., Carrillo, C., Sadeghi, N., Breen, E. C., Witarama, T., Yokomizo, M., Lavretsky, H., Carroll, J. E., Motivala, S. J., Bootzin, R., & Nicassio, P. (2014). Cognitive behavioral therapy vs. Tai Chi for late life insomnia and inflammatory risk: A randomized controlled comparative efficacy trial. Sleep, 37(9), 1543–1552. https://doi.org/10.5665/sleep.4008
- Irwin, M. R., Olmstead, R., Carrillo, C., Sadeghi, N., Nicassio, P., Ganz, P. A., & Bower, J. E. (2017). Tai Chi Chih compared with cognitive behavioral therapy for the treatment of insomnia in survivors of breast cancer: A randomized, partially blinded, noninferiority trial. Journal of Clinical Oncology: Official Journal of the American Society of Clinical Oncology, 35(23), 2656–2665. https://doi.org/10.1200/JCO.2016.71.0285
- Jaffee, S. R., Bowes, L., Ouellet-Morin, I., Fisher, H. L., Moffitt, T. E., Merrick, M. T., & Arseneault, L. (2013). Safe, stable, nurturing relationships break the intergenerational cycle of abuse: A prospective nationally representative cohort of children in the United Kingdom. The Journal of Adolescent Health: Official Publication of the Society for Adolescent Medicine, 53(4 Suppl), S4–S10. https://doi.org/10.1016/j.jadohealth.2013.04.007
- Jalali, M., Mahmoodi, M., Moosavian, S. P., Jalali, R., Ferns, G., Mosallanezhad, A., Imanieh, M. H., & Mosallanezhad, Z. (2020). The effects of ginger supplementation on markers of inflammatory and oxidative stress: A systematic review and meta-analysis of clinical trials. Phytotherapy Research: PTR, 34(8), 1723–1733. https://doi.org/10.1002/ptr.6638
- Jarcho, M. R., Slavich, G. M., Tylova-Stein, H., Wolkowitz, O. M., & Burke, H. M. (2013). Dysregulated diurnal cortisol pattern is associated with glucocorticoid resistance in women with major depressive disorder. Biological Psychology, 93(1), 150–158. https://doi.org/10.1016/j.biopsycho.2013.01.018
- Jasienska, G., Bribiescas, R. G., Furberg, A.-S., Helle, S., & Núñez-de la Mora, A. (2017). Human reproduction and health: An evolutionary perspective. Lancet, 390(10093), 510–520. https://doi.org/10.1016/S0140-6736(17)30573-1
- Jerath, R., Beveridge, C., & Barnes, V. A. (2018). Self-regulation of breathing as an adjunctive treatment of insomnia. Frontiers in Psychiatry, 9, 780. https://doi.org/10.3389/fpsyt.2018.00780
- Jokić-Begić, N., & Begić, D. (2003). Quantitative electroencephalogram (qEEG) in combat veterans with post-traumatic stress disorder (PTSD). Nordic Journal of Psychiatry, 57(5), 351–355. https://doi.org/10.1080/08039480310002688
- Jones, R., Tarter, R., & Ross, A. M. (2021). Greenspace interventions, stress and cortisol: A scoping review. International Journal of Environmental Research and Public Health, 18(6), 2802. https://doi.org/10.3390/ijerph18062802
- Joung, K. E., Park, K.-H., Zaichenko, L., Sahin-Efe, A., Thakkar, B., Brinkoetter, M., Usher, N., Warner, D., Davis, C. R., Crowell, J. A., & Mantzoros, C. S. (2014). Early life adversity is associated with elevated levels of circulating leptin, irisin, and decreased levels of adiponectin in midlife adults. The Journal of Clinical Endocrinology and Metabolism, 99(6), E1055–E1060. https://doi.org/10.1210/jc.2013-3669
- Kajeepeta, S., Gelaye, B., Jackson, C. L., & Williams, M. A. (2015). Adverse childhood experiences are associated with adult sleep disorders: A systematic review. Sleep Medicine, 16(3), 320–330. https://doi.org/10.1016/j.sleep.2014.12.013
- Kandola, A., Hendrikse, J., Lucassen, P. J., & Yücel, M. (2016). Aerobic exercise as a tool to improve hippocampal plasticity and function in humans: Practical implications for mental health treatment. Frontiers in Human Neuroscience, 10, 373. https://doi.org/10.3389/fnhum.2016.00373
- Kar, N. (2011). Cognitive behavioral therapy for the treatment of post-traumatic stress disorder: A review. Neuropsychiatric Disease and Treatment, 7, 167–181. https://doi.org/10.2147/NDT.S10389
- Kaski, D., & Seemungal, B. M. (2010). The bedside assessment of vertigo. Clinical Medicine, 10(4), 402–405. https://doi.org/10.7861/clinmedicine.10-4-402
- Kearney, B. E., Corrigan, F. M., Frewen, P. A., Nevill, S., Harricharan, S., Andrews, K., Jetly, R., McKinnon, M. C., & Lanius, R. A. (2023). A randomized controlled trial of Deep Brain Reorienting: A neuroscientifically guided treatment for post-traumatic stress disorder. European Journal of Psychotraumatology, 14(2), 2240691. https://doi.org/10.1080/20008066.2023.2240691
- Kearney, B. E., & Lanius, R. A. (2022). The brain-body disconnect: A somatic sensory basis for trauma-related disorders. Frontiers in Neuroscience, 16, 1015749. https://doi.org/10.3389/fnins.2022.1015749
- Kemeny, M. E. (2003). The psychobiology of stress. Current Directions in Psychological Science, 12(4), 124–129. https://doi.org/10.1111/1467-8721.01246
- Kiecolt-Glaser, J. K. (2010). Stress, food, and inflammation: Psychoneuroimmunology and nutrition at the cutting edge. Psychosomatic Medicine, 72(4), 365–369. https://doi.org/10.1097/PSY.0b013e3181dbf489
- Kiecolt-Glaser, J. K., Glaser, R., & Christian, L. M. (2014). Omega-3 fatty acids and stress-induced immune dysregulation: Implications for wound healing. Military Medicine, 179(11 Suppl), 129–133. https://doi.org/10.7205/MILMED-D-14-00167
- Kim, H.-G., Cheon, E.-J., Bai, D.-S., Lee, Y. H., & Koo, B.-H. (2018). Stress and heart rate variability: A meta-analysis and review of the literature. Psychiatry Investigation, 15(3), 235–245. https://doi.org/10.30773/pi.2017.08.17
- Kim, J. J., & Diamond, D. M. (2002). The stressed hippocampus, synaptic plasticity and lost memories. Nature Reviews. Neuroscience, 3(6), 453–462. https://doi.org/10.1038/nrn849
- Kim, E.-J., & Dimsdale, J. E. (2007). The effect of psychosocial stress on sleep: A review of polysomnographic evidence. Behavioral Sleep Medicine, 5(4), 256–278. https://doi.org/10.1080/15402000701557383
- Kipps, C. M., & Hodges, J. R. (2005). Cognitive assessment for clinicians. Journal of Neurology, Neurosurgery, and Psychiatry, 76 (suppl 1), i22–i30. https://doi.org/10.1136/jnnp.2004.059758
- Kjaer, T. W., Bertelsen, C., Piccini, P., Brooks, D., Alving, J., & Lou, H. C. (2002). Increased dopamine tone during meditation-induced change of consciousness. Brain Research. Cognitive Brain Research, 13(2), 255–259. https://doi.org/10.1016/s0926-6410(01)00106-9.
- Koch, C. E., Leinweber, B., Drengberg, B. C., Blaum, C., & Oster, H. (2017). Interaction between circadian rhythms and stress. Neurobiology of Stress, 6, 57–67. https://doi.org/10.1016/j.ynstr.2016.09.001
- Komori, T. (2018). The relaxation effect of prolonged expiratory breathing. Mental Illness, 10(1), 7669–7667. https://doi.org/10.4081/mi.2018.7669
- Koncz, A., Demetrovics, Z., & Takacs, Z. K. (2021). Meditation interventions efficiently reduce cortisol levels of at-risk samples: A meta-analysis. Health Psychology Review, 15(1), 56–84. https://doi.org/10.1080/17437199.2020.1760727
- Korgaonkar, M. S., Williamson, T., & Bryant, R. A. (2021). Neural activity during response inhibition in mild traumatic brain injury and posttraumatic stress disorder. Neurobiology of Stress, 14, 100308. https://doi.org/10.1016/j.ynstr.2021.100308
- Kowalczyk, P., Sulejczak, D., Kleczkowska, P., Bukowska-Ośko, I., Kucia, M., Popiel, M., Wietrak, E., Kramkowski, K., Wrzosek, K., & Kaczyńska, K. (2021). Mitochondrial oxidative stress—A causative factor and therapeutic target in many diseases. International Journal of Molecular Sciences, 22(24), 13384. https://doi.org/10.3390/ijms222413384
- Kozlowska, K., Walker, P., McLean, L., & Carrive, P. (2015). Fear and the defense cascade: Clinical implications and management. Harvard Review of Psychiatry, 23(4), 263–287. https://doi.org/10.1097/HRP.0000000000000065
- Kreuz, L. E., Rose, R. M., & Jennings, J. R. (1972). Suppression of plasma testosterone levels and psychological stress. A longitudinal study of young men in Officer Candidate School. Archives of General Psychiatry, 26(5), 479–482. https://doi.org/10.1001/archpsyc.1972.01750230089017
- Kuhfuß, M., Maldei, T., Hetmanek, A., & Baumann, N. (2021). Somatic experiencing–effectiveness and key factors of a body-oriented trauma therapy: A scoping literature review. European Journal of Psychotraumatology, 12(1), 1929023. https://doi.org/10.1080/20008198.2021.1929023
- Kuhlman, K. R., Chiang, J. J., Horn, S., & Bower, J. E. (2017). Developmental psychoneuroendocrine and psychoneuroimmune pathways from childhood adversity to disease. Neuroscience and Biobehavioral Reviews, 80, 166–184. https://doi.org/10.1016/j.neubiorev.2017.05.020
- Lacagnina, A. F., Brockway, E. T., Crovetti, C. R., Shue, F., McCarty, M. J., Sattler, K. P., Lim, S. C., Santos, S. L., Denny, C. A., & Drew, M. R. (2019). Distinct hippocampal engrams control extinction and relapse of fear memory. Nature Neuroscience, 22(5), 753–761. https://doi.org/10.1038/s41593-019-0361-z
- Lanius, R. A. (2015). Trauma-related dissociation and altered states of consciousness: A call for clinical, treatment, and neuroscience research. European Journal of Psychotraumatology, 6(1). https://doi.org/10.3402/ejpt.v6.27905
- Lanius, R. A., Boyd, J. E., McKinnon, M. C., Nicholson, A. A., Frewen, P., Vermetten, E., Jetly, R., & Spiegel, D. (2018). A review of the neurobiological basis of trauma-related dissociation and its relation to cannabinoid- and opioid-mediated stress response: A transdiagnostic, translational approach. Current Psychiatry Reports, 20(12), 118. https://doi.org/10.1007/s11920-018-0983-y
- Lanius, R. A., Frewen, P. A., Tursich, M., Jetly, R., & McKinnon, M. C. (2015). Restoring large-scale brain networks in PTSD and related disorders: A proposal for neuroscientifically-informed treatment interventions. European Journal of Psychotraumatology, 6(1). https://doi.org/10.3402/ejpt.v6.27313
- Le, O., et al. (2023). Childhood adversity and impaired reward processing: A meta-analysis. Child Abuse & Neglect, 142(Pt 1), 105596. https://doi.org/10.1016/j.chiabu.2022.105596
- Lehrer, P., Kaur, K., Sharma, A., Shah, K., Huseby, R., Bhavsar, J., Sgobba, P., & Zhang, Y. (2020). Heart rate variability biofeedback improves emotional and physical health and performance: A systematic review and meta analysis. Applied Psychophysiology and Biofeedback, 45(3), 109–129. https://doi.org/10.1007/s10484-020-09466-z
- Lempert, K. M., Porcelli, A. J., Delgado, M. R., & Tricomi, E. (2012). Individual differences in delay discounting under acute stress: The role of trait perceived stress. Frontiers in Psychology, 3, 251. https://doi.org/10.3389/fpsyg.2012.00251
- Lendor, C., Johnson, A., Perzanowski, M., Chew, G. L., Goldstein, I. F., Kelvin, E., Perera, F., & Miller, R. L. (2008). Effects of winter birth season and prenatal cockroach and mouse allergen exposure on indoor allergen-specific cord blood mononuclear cell proliferation and cytokine production. Annals of Allergy, Asthma & Immunology: Official Publication of the American College of Allergy, Asthma, & Immunology, 101(2), 193–199. https://doi.org/10.1016/S1081-1206(10)60209-8
- Levine, P. A. (2015). Trauma and memory: Brain and body in a search for the living past: A practical guide for understanding and working with traumatic memory. North Atlantic Books.
- Leza, L., Siria, S., López-Goñi, J. J., & Fernández-Montalvo, J. (2021). Adverse childhood experiences (ACEs) and substance use disorder (SUD): A scoping review. Drug and Alcohol Dependence, 221, 108563. https://doi.org/10.1016/j.drugalcdep.2021.108563
- Li, M.-Y., Huang, M.-M., Li, S.-Z., Tao, J., Zheng, G.-H., & Chen, L.-D. (2017). The effects of aerobic exercise on the structure and function of DMN-related brain regions: A systematic review. The International Journal of Neuroscience, 127(7), 634–649. https://doi.org/10.1080/00207454.2016.1212855
- Liddell, B. J., Brown, K. J., Kemp, A. H., Barton, M. J., Das, P., Peduto, A., Gordon, E., & Williams, L. M. (2005). A direct brainstem–amygdala–cortical “alarm” system for subliminal signals of fear. NeuroImage, 24(1), 235–243. https://doi.org/10.1016/j.neuroimage.2004.08.016
- Lieberman, A. F., Ghosh Ippen, C., & P, V. A. N. H. (2006). Child-parent psychotherapy: 6-month follow-up of a randomized controlled trial. Journal of the American Academy of Child and Adolescent Psychiatry, 45(8), 913–918. https://doi.org/10.1097/01.chi.0000222784.03735.92
- Lieberman, A. F. (2004). Child-parent psychotherapy: A relationship-based approach to the treatment of mental health disorders in infancy and early childhood. In Treating parent-infant relationship problems: Strategies for intervention (pp. 97–122). The Guilford Press.
- Lieberman, A. F., Van Horn, P., & Ippen, C. G. (2005). Toward evidence-based treatment: Child-parent psychotherapy with preschoolers exposed to marital violence. Journal of the American Academy of Child and Adolescent Psychiatry, 44(12), 1241–1248. https://doi.org/10.1097/01.chi.0000181047.59702.58
- Linnstaedt, S. D., Zannas, A. S., McLean, S. A., Koenen, K. C., & Ressler, K. J. (2019). Literature review and methodological considerations for understanding circulating risk biomarkers following trauma exposure. Molecular Psychiatry, 25 (9), 1986–1999. https://doi.org/10.1038/s41380-019-0636-5
- Lokshina, Y., Nickelsen, T., & Liberzon, I. (2021). Reward processing and circuit dysregulation in posttraumatic stress disorder. Frontiers in Psychiatry, 12, 559401. https://doi.org/10.3389/fpsyt.2021.559401
- Lorenc, T., Lester, S., Sutcliffe, K., Stansfield, C., & Thomas, J. (2020). Interventions to support people exposed to adverse childhood experiences: Systematic review of systematic reviews. BMC Public Health, 20(1), 657. https://doi.org/10.1186/s12889-020-08789-0
- Luby, J. L., Gilbert, K., Whalen, D., Tillman, R., & Barch, D. M. (2020). The differential contribution of the components of parent-child interaction therapy emotion development for treatment of preschool depression. Journal of the American Academy of Child and Adolescent Psychiatry, 59(7), 868–879. https://doi.org/10.1016/j.jaac.2019.07.937
- Lucero, R., Jones, A. C., & Hunsaker, J. C. (2018). Using internal family systems theory in the treatment of combat veterans with post-traumatic stress disorder and their families. Contemporary Family Therapy, 40(3), 266–275. https://doi.org/10.1007/s10591-017-9424-z
- Lund, J. I., Toombs, E., Radford, A., Boles, K., & Mushquash, C. (2020). Adverse childhood experiences and executive function difficulties in children: A systematic review. Child Abuse & Neglect, 106, 104485. https://doi.org/10.1016/j.chiabu.2020.104485
- MacKinnon, D. F. (2008). Bipolar disorder as maladaptive arousal: A behavioral model and evidence. Annals of the New York Academy of Sciences, 1129(1), 185–189. https://doi.org/10.1196/annals.1417.000
- Malejko, K., Abler, B., Plener, P. L., & Straub, J. (2017). Neural correlates of psychotherapeutic treatment of post-traumatic stress disorder: A systematic literature review. Frontiers in Psychiatry, 8, 85. https://doi.org/10.3389/fpsyt.2017.00085
- Manka, L. A., & Wechsler, M. E. (2018). Selecting the right biologic for your patients with severe asthma. Annals of Allergy, Asthma & Immunology: official Publication of the American College of Allergy, Asthma, & Immunology, 121(4), 406–413. https://doi.org/10.1016/j.anai.2018.07.033
- Manthey, A., Sierk, A., Brakemeier, E.-L., Walter, H., & Daniels, J. K. (2021). Does trauma-focused psychotherapy change the brain? A systematic review of neural correlates of therapeutic gains in PTSD. European Journal of Psychotraumatology, 12(1), 1929025. https://doi.org/10.1080/20008198.2021.1929025
- Maren, S. (2001). Neurobiology of pavlovian fear conditioning. Annual Review of Neuroscience, 24(1), 897–931. https://doi.org/10.1146/annurev.neuro.24.1.897
- Marie-Mitchell, A., & Kostolansky, R. (2019). A systematic review of trials to improve child outcomes associated with adverse childhood experiences. American Journal of Preventive Medicine, 56(5), 756–764. https://doi.org/10.1016/j.amepre.2018.11.030
- Marques, A. H., O’Connor, T. G., Roth, C., Susser, E., & Bjørke-Monsen, A.-L. (2013). The influence of maternal prenatal and early childhood nutrition and maternal prenatal stress on offspring immune system development and neurodevelopmental disorders. Frontiers in Neuroscience, 7, 120. https://doi.org/10.3389/fnins.2013.00120
- Martin, E. C., Leue, C., Delespaul, P., Peeters, F., Janssen, A. M. L., Lousberg, R., Erdkamp, A., van de Weijer, S., Widdershoven, J., Blom, H., Bruintjes, T., Zwergal, A., Grill, E., Guinand, N., Perez-Fornos, A., van de Berg, M. R., Stultiens, J. J. A., Kingma, H., & van de Berg, R. (2020). Introducing the DizzyQuest: An app-based diary for vestibular disorders. Journal of Neurology, 267(Suppl 1), 3–14. https://doi.org/10.1007/s00415-020-10092-2
- Maté, G. (2008). In the realm of hungry ghosts: Close encounters with addiction. Random House Digital, Inc.
- Mayer, S. E., Guan, J., Lin, J., Hamlat, E., Parker, J. E., Brownell, K., Price, C., Mujahid, M., Tomiyama, A. J., Slavich, G. M., Laraia, B. A., & Epel, E. S. (2023). Intergenerational effects of maternal lifetime stressor exposure on offspring telomere length in Black and White women. Psychological Medicine, 53(13), 6171–6182. https://doi.org/10.1017/S0033291722003397
- McEwen, B. S. (2000a). Allostasis and allostatic load: Implications for neuropsychopharmacology. Neuropsychopharmacology: Official Publication of the American College of Neuropsychopharmacology, 22(2), 108–124. https://doi.org/10.1016/S0893-133X(99)00129-3
- McEwen, B. S. (2000b). The neurobiology of stress: From serendipity to clinical relevance. Brain Research, 886(1–2), 172–189. https://doi.org/10.1016/s0006-8993(00)02950-4
- McEwen, B. S. (2006). Protective and damaging effects of stress mediators: Central role of the brain. Dialogues in Clinical Neuroscience, 8(4), 367–381. https://doi.org/10.31887/DCNS.2006.8.4/bmcewen
- McEwen, B. S. (2007). Physiology and neurobiology of stress and adaptation: Central role of the brain. Physiological Reviews, 87(3), 873–904. https://doi.org/10.1152/physrev.00041.2006
- McEwen, B. S. (2015). Biomarkers for assessing population and individual health and disease related to stress and adaptation. Metabolism, 64(3), S2–S10. https://doi.org/10.1016/j.metabol.2014.10.029
- Melzack, R., & Raja, S. N. (2005). The McGill pain questionnaire: From description to measurement. Anesthesiology, 103(1), 199–202. https://doi.org/10.1097/00000542-200507000-00028
- Mengelkoch, S., Miryam Schüssler-Fiorenza Rose, S., Lautman, Z., Alley, J. C., Roos, L. G., Ehlert, B., Moriarity, D. P., Lancaster, S., Snyder, M. P., & Slavich, G. M. (2023). Multi-omics approaches in psychoneuroimmunology and health research: Conceptual considerations and methodological recommendations. Brain, Behavior, and Immunity, 114, 475–487. https://doi.org/10.1016/j.bbi.2023.07.022
- Menon, V. (2011). Large-scale brain networks and psychopathology: A unifying triple network model. Trends in Cognitive Sciences, 15(10), 483–506. https://doi.org/10.1016/j.tics.2011.08.003
- Merzenich, M. M., Van Vleet, T. M., & Nahum, M. (2014). Brain plasticity-based therapeutics. Frontiers in Human Neuroscience, 8, 385. https://doi.org/10.3389/fnhum.2014.00385
- Meyer-Lindenberg, A., Domes, G., Kirsch, P., & Heinrichs, M. (2011). Oxytocin and vasopressin in the human brain: Social neuropeptides for translational medicine. Nature Reviews. Neuroscience, 12(9), 524–538. https://doi.org/10.1038/nrn3044
- Miller, G. E., & Chen, E. (2006). Life stress and diminished expression of genes encoding glucocorticoid receptor and beta2-adrenergic receptor in children with asthma. Proceedings of the National Academy of Sciences of the United States of America, 103(14), 5496–5501. https://doi.org/10.1073/pnas.0506312103
- Miller, G. E., & Chen, E. (2010). Harsh family climate in early life presages the emergence of a proinflammatory phenotype in adolescence. Psychological Science, 21(6), 848–856. https://doi.org/10.1177/0956797610370161
- Miller, G. E., Chen, E., Fok, A. K., Walker, H., Lim, A., Nicholls, E. F., Cole, S., & Kobor, M. S. (2009). Low early-life social class leaves a biological residue manifested by decreased glucocorticoid and increased proinflammatory signaling. Proceedings of the National Academy of Sciences of the United States of America, 106(34), 14716–14721. https://doi.org/10.1073/pnas.0902971106
- Miller, G. E., Chen, E., & Zhou, E. S. (2007). If it goes up, must it come down? Chronic stress and the hypothalamic-pituitary-adrenocortical axis in humans. Psychological Bulletin, 133(1), 25–45. https://doi.org/10.1037/0033-2909.133.1.25
- Miller, G. E., & Cohen, S. (2001). Psychological interventions and the immune system: A meta-analytic review and critique. Health Psychology: Official Journal of the Division of Health Psychology, American Psychological Association, 20(1), 47–63. https://doi.org/10.1037/0278-6133.20.1.47
- Miller, G. E., Cohen, S., & Ritchey, A. K. (2002). Chronic psychological stress and the regulation of pro-inflammatory cytokines: A glucocorticoid-resistance model. Health Psychology: Official Journal of the Division of Health Psychology, American Psychological Association, 21(6), 531–541. https://doi.org/10.1037//0278-6133.21.6.531
- Mioshi, E., Dawson, K., Mitchell, J., Arnold, R., & Hodges, J. R. (2006). The Addenbrooke’s Cognitive Examination Revised (ACE-R): A brief cognitive test battery for dementia screening. International Journal of Geriatric Psychiatry, 21(11), 1078–1085. https://doi.org/10.1002/gps.1610
- Molina-Torres, G., Rodriguez-Arrastia, M., Roman, P., Sanchez-Labraca, N., & Cardona, D. (2019). Stress and the gut microbiota-brain axis. Behavioural Pharmacology, 30(2 and 3-Spec Issue), 187–200. https://doi.org/10.1097/FBP.0000000000000478
- Monroe, S. M., & Slavich, G. M. (2016). Chapter 13 - psychological stressors: Overview. In G. Fink (Ed.), Stress: Concepts, cognition, emotion, and behavior (pp. 109–115). Academic Press. https://doi.org/10.1016/B978-0-12-800951-2.00013-3
- Moore, R. C., Swendsen, J., & Depp, C. A. (2017). Applications for self-administered mobile cognitive assessments in clinical research: A systematic review. International Journal of Methods in Psychiatric Research, 26(4), e1562. https://doi.org/10.1002/mpr.1562
- Morena, M., Patel, S., Bains, J. S., & Hill, M. N. (2016). Neurobiological interactions between stress and the endocannabinoid system. Neuropsychopharmacology: Official Publication of the American College of Neuropsychopharmacology, 41(1), 80–102. https://doi.org/10.1038/npp.2015.166
- Morton, K. R., Lee, J. W., & Spencer-Hwang, R. (2021). Plant-based dietary intake moderates adverse childhood experiences association with early mortality in an older Adventist cohort. Journal of Psychosomatic Research, 151, 110633. https://doi.org/10.1016/j.jpsychores.2021.110633
- Muench, A., Vargas, I., Grandner, M. A., Ellis, J. G., Posner, D., Bastien, C. H., Drummond, S. P. A., & Perlis, M. L. (2022). We know CBT-I works, now what? Faculty Reviews, 11, 4. https://doi.org/10.12703/r/11-4
- Mulraney, M., Sciberras, E., Payne, J. M., De Luca, C., Mills, J., Tennant, M., & Coghill, D. (2022). Collaborative and proactive solutions compared with usual care to treat irritability in children and adolescents: A pilot randomized controlled trial. Clinical Psychologist, 26(2), 231–239. https://doi.org/10.1080/13284207.2022.2041983
- Murawski, B., Wade, L., Plotnikoff, R. C., Lubans, D. R., & Duncan, M. J. (2018). A systematic review and meta-analysis of cognitive and behavioral interventions to improve sleep health in adults without sleep disorders. Sleep Medicine Reviews, 40, 160–169. https://doi.org/10.1016/j.smrv.2017.12.003
- National Academies of Sciences, E., and Medicine. (2019). Vibrant and healthy kids: Aligning science, practice, and policy to advance health equity. The National Academies Press. https://doi.org/10.17226/25466
- Nelson, C. A., & Gabard-Durnam, L. J. (2020). Early adversity and critical periods: Neurodevelopmental consequences of violating the expectable environment. Trends in Neurosciences, 43(3), 133–143. https://doi.org/10.1016/j.tins.2020.01.002
- Nelson, C. A., Scott, R. D., Bhutta, Z. A., Harris, N. B., Danese, A., & Samara, M. (2020). Adversity in childhood is linked to mental and physical health throughout life. BMJ (Clinical Research ed.), 371, m3048. https://doi.org/10.1136/bmj.m3048
- Neuchat, E. E., Bocklud, B. E., Kingsley, K., Barham, W. T., Luther, P. M., Ahmadzadeh, S., Shekoohi, S., Cornett, E. M., & Kaye, A. D. (2023). The role of alpha-2 agonists for attention deficit hyperactivity disorder in children: A review. Neurology International, 15(2), 697–707. https://doi.org/10.3390/neurolint15020043
- Neuhaus, E. C., & Slavich, G. M. (2022). Behavioral psychedelics: Integrating mind and behavior to improve health and resilience. Frontiers in Psychiatry, 13, 821208. https://doi.org/10.3389/fpsyt.2022.821208
- NIH Toolbox. (2023). https://www.healthmeasures.net/explore-measurement-systems/nih-toolbox
- NIH. (2022). Emotional wellness toolkit. National Institutes of Health (NIH). https://www.nih.gov/health-information/emotional-wellness-toolkit
- Nouchi, R., Taki, Y., Takeuchi, H., Hashizume, H., Nozawa, T., Kambara, T., Sekiguchi, A., Miyauchi, C. M., Kotozaki, Y., Nouchi, H., & Kawashima, R. (2013). Brain training game boosts executive functions, working memory and processing speed in the young adults: A randomized controlled trial. PloS One, 8(2), e55518. https://doi.org/10.1371/journal.pone.0055518
- Novick, A. M., Levandowski, M. L., Laumann, L. E., Philip, N. S., Price, L. H., & Tyrka, A. R. (2018). The effects of early life stress on reward processing. Journal of Psychiatric Research, 101, 80–103. https://doi.org/10.1016/j.jpsychires.2018.02.002
- Ntamati, N. R., Creed, M., Achargui, R., & Lüscher, C. (2018). Periaqueductal efferents to dopamine and GABA neurons of the VTA. PloS One, 13(1), e0190297. https://doi.org/10.1371/journal.pone.0190297
- O’Brien, S. M., Fitzgerald, P., Scully, P., Landers, A., Scott, L. V., & Dinan, T. G. (2007). Impact of gender and menstrual cycle phase on plasma cytokine concentrations. Neuroimmunomodulation, 14(2), 84–90. https://doi.org/10.1159/000107423
- O’Mahony, S. M., Clarke, G., Borre, Y. E., Dinan, T. G., & Cryan, J. F. (2015). Serotonin, tryptophan metabolism and the brain-gut-microbiome axis. Behavioural Brain Research, 277, 32–48. https://doi.org/10.1016/j.bbr.2014.07.027
- O’Connor, D. B., Thayer, J. F., & Vedhara, K. (2021). Stress and health: A review of psychobiological processes. Annual Review of Psychology, 72(1), 663–688. https://doi.org/10.1146/annurev-psych-062520-122331
- Ogden, P., & Minton, K. (2000). Sensorimotor psychotherapy: One method for processing traumatic memory. Traumatology, 6(3), 149–173. https://doi.org/10.1177/153476560000600302
- Ohashi, K., Anderson, C. M., Bolger, E. A., Khan, A., McGreenery, C. E., & Teicher, M. H. (2019). Susceptibility or resilience to maltreatment can be explained by specific differences in brain network architecture. Biological Psychiatry, 85(8), 690–702. https://doi.org/10.1016/j.biopsych.2018.10.016
- Ortiz, R., Gilgoff, R., & Burke Harris, N. (2022). Adverse childhood experiences, toxic stress, and trauma-informed neurology. JAMA Neurology, 79(6), 539–540. https://doi.org/10.1001/jamaneurol.2022.0769
- Palm-Fischbacher, S., & Ehlert, U. (2014). Dispositional resilience as a moderator of the relationship between chronic stress and irregular menstrual cycle. Journal of Psychosomatic Obstetrics and Gynaecology, 35(2), 42–50. https://doi.org/10.3109/0167482X.2014.912209
- Pascoe, M. C., Thompson, D. R., Jenkins, Z. M., & Ski, C. F. (2017). Mindfulness mediates the physiological markers of stress: Systematic review and meta-analysis. Journal of Psychiatric Research, 95, 156–178. https://doi.org/10.1016/j.jpsychires.2017.08.004
- Patterson, J. A. (2014). Validation of measures from the smartphone sway balance application: A pilot study. International Journal of Sports Physical Therapy, 9(2), 135–139.
- Peake, J. M., Kerr, G., & Sullivan, J. P. (2018). A critical review of consumer wearables, mobile applications, and equipment for providing biofeedback, monitoring stress, and sleep in physically active populations. Frontiers in Physiology, 9, 743. https://doi.org/10.3389/fphys.2018.00743
- Perry. (2001). The neuroarcheology of childhood maltreatment the neurodevelopmental costs of adverse childhood events. In K. Franey, R. Falconer, & R. Geffner (Eds.), The cost of maltreatment: Who pays? We all do (pp. 15–37). Family Violence and Sexual Assault Institute.
- Perry, B. (2006). Applying principles of neurodevelopment to clinical work with malt0reated and traumatized children: The neurosequential model of therapeutics. In N. B. Webb (Ed.), Working with traumatized youth in child welfare (pp. 27–52). The Guilford Press (Social Work Practice with Children and Families).
- Perry, B. (2009). Examining child maltreatment through a neurodevelopmental lens: Clinical applications of the neurosequential model of therapeutics. Journal of Loss and Trauma, 14(4), 240–255. https://doi.org/10.1080/15325020903004350
- Perry, B. (2013). The neurosequential model of therapeutics in young children. In K. Brandt, B. D. Perry, S. Seligman, & E. Tronick (Eds.), Infant and early childhood mental health (pp. 21–47). American Psychiatric Press.
- Perry, B. (2020). The neurosequential model A developmentally-sensitive, neuroscience-informed approach to clinical problem solving. In J. Tucci, J. Mitchell, & E. C. Tronick (Eds.), The handbook of therapeutic child care: evidence-informed approaches to working with traumatized children in foster, relative and adoptive care. Jessica Kingsley.
- Perry, B., & Dobson, C. L. (2013). Application of the neurosequential model (NMT) in maltreated children. In J. D. Ford, & C. A. Courtois (Eds.), Treating complex traumatic stress disorders in children and adolescents: Scientific foundations and therapeutic models (pp. 249–260). The Guilford Press.
- Perry, B., & Hambrick, E. P. (2008). The neurosequential model of therapeutics. Reclaiming Children and Youth, 17(3), 38–43.
- Perry, B., & Pollard, R. (1998). Homeostasis, stress, trauma, and adaptation. A neurodevelopmental view of childhood trauma. Child and Adolescent Psychiatric Clinics of North America, 7(1), 33–51. https://doi.org/10.1016/S1056-4993(18)30258-X
- Perry, B. D., Pollard, R. A., Blakley, T. L., Baker, W. L., & Vigilante, D. (1995). Childhood trauma, the neurobiology of adaptation, and “use-dependent” development of the brain: How “states” become “traits. Infant Mental Health Journal, 16(4), 271–291. https://doi.org/10.1002/1097-0355(199524)16:4<271::AID-IMHJ2280160404>3.0.CO;2-B
- Perry, B., & Szalavitz, M. (2017). The boy who was raised as a dog: And other stories from a child psychiatrist’s notebook–What traumatized children can teach us about loss, love, and healing. Hachette.
- Perry, B., & Winfrey, O. (2021). What happened to you? Conversations on trauma, resilience, and healing. Flatiron Books.
- Peters, A. T., Burkhouse, K. L., Kinney, K. L., & Phan, K. L. (2019). The roles of early-life adversity and rumination in neural response to emotional faces amongst anxious and depressed adults. Psychological Medicine, 49(13), 2267–2278. https://doi.org/10.1017/S0033291718003203
- Piazza, J. R., Almeida, D. M., Dmitrieva, N. O., & Klein, L. C. (2010). Frontiers in the use of biomarkers of health in research on stress and aging. The Journals of Gerontology. Series B, Psychological Sciences and Social Sciences, 65(5), 513–525. https://doi.org/10.1093/geronb/gbq049
- Picard, M., & McEwen, B. S. (2018). Psychological stress and mitochondria: A systematic review. Psychosomatic Medicine, 80(2), 141–153. https://doi.org/10.1097/PSY.0000000000000545
- Pierce, Z. P., & Black, J. M. (2023). The neurophysiology behind trauma-focused therapy modalities used to treat post-traumatic stress disorder across the life course: A systematic review. Trauma, Violence & Abuse, 24(2), 1106–1123. https://doi.org/10.1177/15248380211048446
- Plassmann, H., O’Doherty, J., & Rangel, A. (2007). Orbitofrontal cortex encodes willingness to pay in everyday economic transactions. The Journal of Neuroscience: The Official Journal of the Society for Neuroscience, 27(37), 9984–9988. https://doi.org/10.1523/JNEUROSCI.2131-07.2007
- Portnoy, J., Raine, A., Liu, J., & Hibbeln, J. R. (2018). Reductions of intimate partner violence resulting from supplementing children with omega-3 fatty acids: A randomized, double-blind, placebo-controlled, stratified, parallel-group trial. Aggressive Behavior, 44 (5), 491–500. https://doi.org/10.1002/ab.21769
- Powers, M. B., Halpern, J. M., Ferenschak, M. P., Gillihan, S. J., & Foa, E. B. (2010). A meta-analytic review of prolonged exposure for posttraumatic stress disorder. Clinical Psychology Review, 30(6), 635–641. https://doi.org/10.1016/j.cpr.2010.04.007
- Proffitt Leyva, R. P., Mengelkoch, S., Gassen, J., Ellis, B. J., Russell, E. M., & Hill, S. E. (2020). Low socioeconomic status and eating in the absence of hunger in children aged 3-14. Appetite, 154, 104755. https://doi.org/10.1016/j.appet.2020.104755
- Purewal Boparai, S. K., Au, V., Koita, K., Oh, D. L., Briner, S., Burke Harris, N., & Bucci, M. (2018). Ameliorating the biological impacts of childhood adversity: A review of intervention programs. Child Abuse & Neglect, 81, 82–105. https://doi.org/10.1016/j.chiabu.2018.04.014
- Radley, J. J., & Herman, J. P. (2022). Preclinical models of chronic stress: Adaptation or pathology? Biological Psychiatry, 94(3), 194–202. https://doi.org/10.1016/j.biopsych.2022.11.004
- Radwan, B., Yanez Touzet, A., Hammami, S., & Chaudhury, D. (2021). Prolonged exposure to social stress impairs homeostatic sleep regulation. Frontiers in Neuroscience, 15, 633955. https://doi.org/10.3389/fnins.2021.633955
- Raj, S. R., Black, B. K., Biaggioni, I., Paranjape, S. Y., Ramirez, M., Dupont, W. D., & Robertson, D. (2009). Propranolol decreases tachycardia and improves symptoms in the Postural Tachycardia Syndrome (POTS): Less is More. Circulation, 120(9), 725–734. https://doi.org/10.1161/CIRCULATIONAHA.108.846501
- Raman, G. (2013). Tai Chi improves sleep quality in healthy adults and patients with chronic conditions: A systematic review and meta-analysis. Journal of Sleep Disorders & Therapy, 2(6), 141. https://doi.org/10.4172/2167-0277.1000141
- Raspopow, K., Abizaid, A., Matheson, K., & Anisman, H. (2010). Psychosocial stressor effects on cortisol and ghrelin in emotional and non-emotional eaters: Influence of anger and shame. Hormones and Behavior, 58(4), 677–684. https://doi.org/10.1016/j.yhbeh.2010.06.003
- Raspopow, K., Abizaid, A., Matheson, K., & Anisman, H. (2014). Anticipation of a psychosocial stressor differentially influences ghrelin, cortisol and food intake among emotional and non-emotional eaters. Appetite, 74, 35–43. https://doi.org/10.1016/j.appet.2013.11.018
- Reiff, C. M., Richman, E. E., Nemeroff, C. B., Carpenter, L. L., Widge, A. S., Rodriguez, C. I., Kalin, N. H., & McDonald, W. M, the Work Group on Biomarkers and Novel Treatments, a Division of the American Psychiatric Association Council of Research. (2020). Psychedelics and psychedelic-assisted psychotherapy. The American Journal of Psychiatry, 177(5), 391–410. https://doi.org/10.1176/appi.ajp.2019.19010035
- Reinertsen, E., & Clifford, G. D. (2018). A review of physiological and behavioral monitoring with digital sensors for neuropsychiatric illnesses. Physiological Measurement, 39(5), 05TR01. https://doi.org/10.1088/1361-6579/aabf64
- Retana-Márquez, S., Bonilla-Jaime, H., Vázquez-Palacios, G., Martínez-García, R., & Velázquez-Moctezuma, J. (2003). Changes in masculine sexual behavior, corticosterone and testosterone in response to acute and chronic stress in male rats. Hormones and Behavior, 44(4), 327–337. https://doi.org/10.1016/j.yhbeh.2003.04.001
- Roelofs, K., & Dayan, P. (2022). Freezing revisited: Coordinated autonomic and central optimization of threat coping. Nature Reviews. Neuroscience, 23(9), 568–580. https://doi.org/10.1038/s41583-022-00608-2
- Rooks, C., Veledar, E., Goldberg, J., Bremner, J. D., & Vaccarino, V. (2012). Early trauma and inflammation: Role of familial factors in a study of twins. Psychosomatic Medicine, 74(2), 146–152. https://doi.org/10.1097/PSY.0b013e318240a7d8
- Roos, L. G., & Slavich, G. M. (2023). Wearable technologies for health research: Opportunities, limitations, and practical and conceptual considerations. Brain, Behavior, and Immunity, 113, 444–452. https://doi.org/10.1016/j.bbi.2023.08.008
- Ross, J. A., & Van Bockstaele, E. J. (2020). The locus coeruleus- norepinephrine system in stress and arousal: Unraveling historical, current, and future perspectives. Frontiers in Psychiatry, 11, 601519. https://doi.org/10.3389/fpsyt.2020.601519
- Rowe, J., Kusel, M., Holt, B. J., Suriyaarachchi, D., Serralha, M., Hollams, E., Yerkovich, S. T., Subrata, L. S., Ladyman, C., Sadowska, A., Gillett, J., Fisher, E., Loh, R., Soderstrom, L., Ahlstedt, S., Sly, P. D., & Holt, P. G. (2007). Prenatal versus postnatal sensitization to environmental allergens in a high-risk birth cohort. Journal of Allergy and Clinical Immunology, 119(5), 1164–1173. https://doi.org/10.1016/j.jaci.2007.02.016
- Rusch, H. L., Rosario, M., Levison, L. M., Olivera, A., Livingston, W. S., Wu, T., & Gill, J. M. (2019). The effect of mindfulness meditation on sleep quality: A systematic review and meta-analysis of randomized controlled trials. Annals of the New York Academy of Sciences, 1445(1), 5–16. https://doi.org/10.1111/nyas.13996
- Ryan, J., Pouliot, J. J., Hajcak, G., & Nee, D. E. (2022). Manipulating reward sensitivity using reward circuit–targeted transcranial magnetic stimulation. Biological Psychiatry. Cognitive Neuroscience and Neuroimaging, 7(8), 833–840. https://doi.org/10.1016/j.bpsc.2022.02.011
- Sadeh, A. (1996). Stress, trauma, and sleep in children. Child and Adolescent Psychiatric Clinics of North America, 5(3), 685–700. https://doi.org/10.1016/S1056-4993(18)30356-0
- Saman, Y. (2020). Chapter Nine - Stress and the vestibular system. In A. Clow and N. Smyth (Eds.), International review of neurobiology (pp. 221–236). Academic Press (Stress and Brain Health: In Clinical Conditions). https://doi.org/10.1016/bs.irn.2020.03.013
- Saman, Y., Bamiou, D. E., Gleeson, M., & Dutia, M. B. (2012). Interactions between stress and vestibular compensation – A review. Frontiers in Neurology, 3, 116. https://doi.org/10.3389/fneur.2012.00116
- Sapolsky, R. M., Romero, L. M., & Munck, A. U. (2000). How do glucocorticoids influence stress responses? Integrating permissive, suppressive, stimulatory, and preparative actions. Endocrine Reviews, 21(1), 55–89. https://doi.org/10.1210/edrv.21.1.0389
- Schaal, N. K., Hepp, P., Schweda, A., Wolf, O. T., & Krampe, C. (2019). A functional near-infrared spectroscopy study on the cortical haemodynamic responses during the Maastricht acute stress test. Scientific Reports, 9(1), 13459. https://doi.org/10.1038/s41598-019-49826-2
- Schimmenti, A., Billieux, J., Santoro, G., Casale, S., & Starcevic, V. (2022). A trauma model of substance use: Elaboration and preliminary validation. Addictive Behaviors, 134, 107431. https://doi.org/10.1016/j.addbeh.2022.107431
- Schliep, K. C., Mumford, S. L., Vladutiu, C. J., Ahrens, K. A., Perkins, N. J., Sjaarda, L. A., Kissell, K. A., Prasad, A., Wactawski-Wende, J., & Schisterman, E. F. (2015). Perceived stress, reproductive hormones, and ovulatory function: A prospective cohort study. Epidemiology (Cambridge, Mass.), 26(2), 177–184. https://doi.org/10.1097/EDE.0000000000000238
- Schoen, S. A., Miller, L. J., & Sullivan, J. (2017). The development and psychometric properties of the Sensory Processing Scale Inventory: A report measure of sensory modulation. Journal of Intellectual & Developmental Disability, 42(1), 12–21. https://doi.org/10.3109/13668250.2016.1195490
- Schofield, T. J., Lee, R. D., & Merrick, M. T. (2013). Safe, stable, nurturing relationships as a moderator of intergenerational continuity of child maltreatment: A meta-analysis. The Journal of Adolescent Health: official Publication of the Society for Adolescent Medicine, 53(4 Suppl), S32–S38. https://doi.org/10.1016/j.jadohealth.2013.05.004
- Scionti, N., Cavallero, M., Zogmaister, C., & Marzocchi, G. M. (2019). Is cognitive training effective for improving executive functions in preschoolers? A systematic review and meta-analysis. Frontiers in Psychology, 10, 2812. https://doi.org/10.3389/fpsyg.2019.02812
- Scullin, M. K., Krueger, M. L., Ballard, H. K., Pruett, N., & Bliwise, D. L. (2018). The effects of bedtime writing on difficulty falling asleep: A polysomnographic study comparing to-do lists and completed activity lists. Journal of Experimental Psychology. General, 147(1), 139–146. https://doi.org/10.1037/xge0000374
- Shapiro, F. (2014). The role of eye movement desensitization and reprocessing (EMDR) therapy in medicine: Addressing the psychological and physical symptoms stemming from adverse life experiences. The Permanente Journal, 18(1), 71–77. https://doi.org/10.7812/TPP/13-098
- Sherbourne, C. D., & Stewart, A. L. (1991). The MOS social support survey. Social Science & Medicine (1982), 32(6), 705–714. https://doi.org/10.1016/0277-9536(91)90150-B
- Shields, G. S., Doty, D., Shields, R. H., Gower, G., Slavich, G. M., & Yonelinas, A. P. (2017). Recent life stress exposure is associated with poorer long-term memory, working memory, and self-reported memory. Stress, 20(6), 598–607. https://doi.org/10.1080/10253890.2017.1380620
- Shields, G. S., & Slavich, G. M. (2017). Lifetime stress exposure and health: A review of contemporary assessment methods and biological mechanisms. Social and Personality Psychology Compass, 11(8), e12335. https://doi.org/10.1111/spc3.12335
- Shields, G. S., Spahr, C. M., & Slavich, G. M. (2020). Psychosocial interventions and immune system function: A systematic review and meta-analysis of randomized clinical trials. JAMA Psychiatry, 77(10), 1031–1043. https://doi.org/10.1001/jamapsychiatry.2020.0431
- Shonkoff, J. P., Boyce, W. T., Bush, N. R., Gunnar, M. R., Hensch, T. K., Levitt, P., Meaney, M. J., Nelson, C. A., Slopen, N., Williams, D. R., & Silveira, P. P. (2022). Translating the biology of adversity and resilience into new measures for pediatric practice. Pediatrics, 149(6), e2021054493. https://doi.org/10.1542/peds.2021-054493
- Shonkoff, J. P., & Garner, A. S, Section on Developmental and Behavioral Pediatrics. (2012). The lifelong effects of early childhood adversity and toxic stress. Pediatrics, 129(1), e232–e246. https://doi.org/10.1542/peds.2011-2663
- Shonkoff, J. P., Slopen, N., & Williams, D. R. (2021). Early childhood adversity, toxic stress, and the impacts of racism on the foundations of health. Annual Review of Public Health, 42(1), 115–134. https://doi.org/10.1146/annurev-publhealth-090419-101940
- Slade, A., & Holmes, J. (2019). Attachment and psychotherapy. Current Opinion in Psychology, 25, 152–156. https://doi.org/10.1016/j.copsyc.2018.06.008
- Slavich, G. M. (2015). Understanding inflammation, its regulation, and relevance for health: A top scientific and public priority. Brain, Behavior, and Immunity, 45, 13–14. https://doi.org/10.1016/j.bbi.2014.10.012
- Slavich, G. M. (2016). Life stress and health: A review of conceptual issues and recent findings. Teaching of Psychology (Columbia, Mo.), 43(4), 346–355. https://doi.org/10.1177/0098628316662768
- Slavich, G. M. (2019). Stressnology: The primitive (and problematic) study of life stress exposure and pressing need for better measurement. Brain, Behavior, and Immunity, 75, 3–5. https://doi.org/10.1016/j.bbi.2018.08.011
- Slavich, G. M. (2020). Social safety theory: A biologically based evolutionary perspective on life stress, health, and behavior. Annual Review of Clinical Psychology, 16(1), 265–295. https://doi.org/10.1146/annurev-clinpsy-032816-045159
- Slavich, G. M. (2022). Social Safety Theory: Understanding social stress, disease risk, resilience, and behavior during the COVID-19 pandemic and beyond. Current Opinion in Psychology, 45, 101299. https://doi.org/10.1016/j.copsyc.2022.101299
- Slavich, G. M., & Auerbach, R. P. (2018). Stress and its sequelae: Depression, suicide, inflammation, and physical illness. In APA handbook of psychopathology: Psychopathology: Understanding, assessing, and treating adult mental disorders (Vol. 1, pp. 375–402). American Psychological Association (APA Handbooks in Psychology®). https://doi.org/10.1037/0000064-016
- Slavich, G. M., & Cole, S. W. (2013). The emerging field of human social genomics. Clinical Psychological Science: A Journal of the Association for Psychological Science, 1(3), 331–348. https://doi.org/10.1177/2167702613478594
- Slavich, G. M., & Irwin, M. R. (2014). From stress to inflammation and major depressive disorder: A social signal transduction theory of depression. Psychological Bulletin, 140(3), 774–815. https://doi.org/10.1037/a0035302
- Slavich, G. M., Mengelkoch, S., & Cole, S. W. (2023). Human social genomics: Concepts, mechanisms, and implications for health. Lifestyle Medicine, 4(2), e75. https://doi.org/10.1002/lim2.75
- Slavich, G. M., O’Donovan, A., Epel, E. S., & Kemeny, M. E. (2010b). Black sheep get the blues: A psychobiological model of social rejection and depression. Neuroscience and Biobehavioral Reviews, 35(1), 39–45. https://doi.org/10.1016/j.neubiorev.2010.01.003
- Slavich, G. M., Roos, L. G., Mengelkoch, S., Webb, C. A., Shattuck, E. C., Moriarity, D. P., & Alley, J. C. (2023). Social Safety Theory: Conceptual foundation, underlying mechanisms, and future directions. Health Psychology Review, 17(1), 5–59. https://doi.org/10.1080/17437199.2023.2171900
- Slavich, G. M., Roos, L. G., & Zaki, J. (2022). Social belonging, compassion, and kindness: Key ingredients for fostering resilience, recovery, and growth from the COVID-19 pandemic. Anxiety, Stress, and Coping, 35(1), 1–8. https://doi.org/10.1080/10615806.2021.1950695
- Slavich, G. M., & Sacher, J. (2019). Stress, sex hormones, inflammation, and major depressive disorder: Extending social signal transduction theory of depression to account for sex differences in mood disorders. Psychopharmacology, 236(10), 3063–3079. https://doi.org/10.1007/s00213-019-05326-9
- Slavich, G. M., Stewart, J. G., Esposito, E. C., Shields, G. S., & Auerbach, R. P. (2019). The stress and adversity inventory for adolescents (Adolescent STRAIN): Associations with mental and physical health, risky behaviors, and psychiatric diagnoses in youth seeking treatment. Journal of Child Psychology and Psychiatry, 60(9), 998–1009. https://doi.org/10.1111/jcpp.13038
- Slavich, G. M., Way, B. M., Eisenberger, N. I., & Taylor, S. E. (2010a). Neural sensitivity to social rejection is associated with inflammatory responses to social stress. Proceedings of the National Academy of Sciences of the United States of America, 107(33), 14817–14822. https://doi.org/10.1073/pnas.1009164107
- Slopen, N., McLaughlin, K. A., & Shonkoff, J. P. (2014). Interventions to improve cortisol regulation in children: A systematic review. Pediatrics, 133(2), 312–326. https://doi.org/10.1542/peds.2013-1632
- Spreng, R. N. (2009). The Toronto empathy questionnaire. Journal of Personality Assessment, 91(1), 62–71. https://doi.org/10.1080/00223890802484381
- Steptoe, A., Hamer, M., & Chida, Y. (2007). The effects of acute psychological stress on circulating inflammatory factors in humans: A review and meta-analysis. Brain, Behavior, and Immunity, 21(7), 901–912. https://doi.org/10.1016/j.bbi.2007.03.011
- Suh, D. I., Chang, H. Y., Lee, E., Yang, S. I., & Hong, S. J. (2017). Prenatal maternal distress and allergic diseases in offspring: Review of evidence and possible pathways. Allergy, Asthma & Immunology Research, 9(3), 200–211. https://doi.org/10.4168/aair.2017.9.3.200
- Sullivan, M. J., Bishop, S. R., & Pivik, J. (1995). The pain catastrophizing scale: Development and validation. Psychological Assessment, 7(4), 524–532. https://doi.org/10.1037/1040-3590.7.4.524
- Tanner, B. A. (2012). Validity of global physical and emotional SUDS. Applied Psychophysiology and Biofeedback, 37(1), 31–34. https://doi.org/10.1007/s10484-011-9174-x
- Teicher, M. H., Gordon, J. B., & Nemeroff, C. B. (2022). Recognizing the importance of childhood maltreatment as a critical factor in psychiatric diagnoses, treatment, research, prevention, and education. Molecular Psychiatry, 27(3), 1331–1338. https://doi.org/10.1038/s41380-021-01367-9
- Teicher, M. H., & Samson, J. A. (2016). Annual research review: Enduring neurobiological effects of childhood abuse and neglect. Journal of Child Psychology and Psychiatry, 57(3), 241–266. https://doi.org/10.1111/jcpp.12507
- Teicher, M. H., Samson, J. A., Anderson, C. M., & Ohashi, K. (2016). The effects of childhood maltreatment on brain structure, function and connectivity. Nature Reviews Neuroscience, 17(10), 652–666. https://doi.org/10.1038/nrn.2016.111
- Thayer, R. E. (1986). Activation-deactivation adjective check list: Current overview and structural analysis. Psychological Reports, 58(2), 607–614. https://doi.org/10.2466/pr0.1986.58.2.607
- Thayer, J. F., Hansen, A. L., Saus-Rose, E., & Johnsen, B. H. (2009). Heart rate variability, prefrontal neural function, and cognitive performance: The neurovisceral integration perspective on self-regulation, adaptation, and health. Annals of Behavioral Medicine, 37(2), 141–153. https://doi.org/10.1007/s12160-009-9101-z
- The Lancet. (2020). The Lancet: Latest global disease estimates reveal perfect storm of rising chronic diseases and public health failures fuelling COVID-19 pandemic. The Institute for Health Metrics and Evaluation. https://www.healthdata.org/news-events/newsroom/news-releases/lancet-latest-global-disease-estimates-reveal-perfect-storm
- Thijs, R. D., & van Dijk, J. G. (2006). Stress induced hypotension in pure autonomic failure. Journal of Neurology, Neurosurgery, and Psychiatry, 77(4), 552–553. https://doi.org/10.1136/jnnp.2005.074369
- Thome, J., Densmore, M., Koppe, G., Terpou, B., Théberge, J., McKinnon, M. C., & Lanius, R. A. (2019). Back to the basics: Resting state functional connectivity of the reticular activation system in PTSD and its dissociative subtype. Chronic Stress, 3, 2470547019873663. https://doi.org/10.1177/2470547019873663
- Toepfer, P., Heim, C., Entringer, S., Binder, E., Wadhwa, P., & Buss, C. (2017). Oxytocin pathways in the intergenerational transmission of maternal early life stress. Neuroscience and Biobehavioral Reviews, 73, 293–308. https://doi.org/10.1016/j.neubiorev.2016.12.026
- Toljan, K., & Vrooman, B. (2018). Low-dose naltrexone (LDN)—review of therapeutic utilization. Medical Sciences, 6(4), 82. https://doi.org/10.3390/medsci6040082
- Tombaugh, T. N., & McIntyre, N. J. (1992). The mini-mental state examination: A comprehensive review. Journal of the American Geriatrics Society, 40(9), 922–935. https://doi.org/10.1111/j.1532-5415.1992.tb01992.x
- Tomiyama, A. J. (2019). Stress and obesity. Annual Review of Psychology, 70(1), 703–718. https://doi.org/10.1146/annurev-psych-010418-102936
- Tomiyama, A. J., Schamarek, I., Lustig, R. H., Kirschbaum, C., Puterman, E., Havel, P. J., & Epel, E. S. (2012). Leptin concentrations in response to acute stress predict subsequent intake of comfort foods. Physiology & Behavior, 107(1), 34–39. https://doi.org/10.1016/j.physbeh.2012.04.021
- Tosato, S., Bonetto, C., Tomassi, S., Zanardini, R., Faravelli, C., Bruschi, C., D’Agostino, A., Minelli, A., Scocco, P., Lasalvia, A., Furlato, K., Imbesi, M., Preti, A., Ruggeri, M., Gennarelli, M., & Bocchio-Chiavetto, L, GET UP GROUP. (2020). Childhood trauma and glucose metabolism in patients with first-episode psychosis. Psychoneuroendocrinology, 113, 104536. https://doi.org/10.1016/j.psyneuen.2019.104536
- Tottenham, N., & Sheridan, M. A. (2009). A review of adversity, the amygdala and the hippocampus: A consideration of developmental timing. Frontiers in Human Neuroscience, 3, 68. https://doi.org/10.3389/neuro.09.068.2009.
- Treadway, M. T., Buckholtz, J. W., Schwartzman, A. N., Lambert, W. E., & Zald, D. H. (2009). Worth the “EEfRT”? The effort expenditure for rewards task as an objective measure of motivation and anhedonia. PloS One, 4(8), e6598. https://doi.org/10.1371/journal.pone.0006598
- Tsuji, H., Venditti, F. J., Manders, E. S., Evans, J. C., Larson, M. G., Feldman, C. L., & Levy, D. (1994). Reduced heart rate variability and mortality risk in an elderly cohort. The Framingham Heart Study. Circulation, 90(2), 878–883. https://doi.org/10.1161/01.CIR.90.2.878
- Turunen, T., Haravuori, H., Punamäki, R.-L., Suomalainen, L., & Marttunen, M. (2014). The role of attachment in recovery after a school-shooting trauma. European Journal of Psychotraumatology, 5(1). https://doi.org/10.3402/ejpt.v5.22728
- Uchino, B. N., Bowen, K., Carlisle, M., & Birmingham, W. (2012). Psychological pathways linking social support to health outcomes: A visit with the “ghosts” of research past, present, and future. Social Science & Medicine (1982), 74(7), 949–957. https://doi.org/10.1016/j.socscimed.2011.11.023
- van Bodegom M., Homberg J. R., & Henckens M. J. A. G. (2017). Modulation of the hypothalamic-pituitary-adrenal axis by early life stress exposure. Frontiers in Cellular Neuroscience, 11, 87. https://doi.org/10.3389/fncel.2017.00087.
- Van der Kolk, B. A. (2015). The body keeps the score: brain, mind, and body in the healing of trauma. Penguin Books.
- Vinkers, C. H., Kalafateli, A. L., Rutten, B. P. F., Kas, M. J., Kaminsky, Z., Turner, J. D., & Boks, M. P. M. (2015). Traumatic stress and human DNA methylation: A critical review. Epigenomics, 7(4), 593–608. https://doi.org/10.2217/epi.15.11
- Wang, Z., & Young, M. R. I. (2016). PTSD, a disorder with an immunological component. Frontiers in Immunology, 7, 219. (Accessed: July 6, 2023). https://doi.org/10.3389/fimmu.2016.00219
- Warner, E., Spinazzola, J., Westcott, A., Gunn, C., & Hodgdon, H. (2014). The body can change the score: Empirical support for somatic regulation in the treatment of traumatized adolescents. Journal of Child & Adolescent Trauma, 7(4), 237–246. https://doi.org/10.1007/s40653-014-0030-z
- Watson, E. (2023). PhD A theory of change for primary prevention in the us, prevent child abuse America. https://preventchildabuse.org/theory-of-change/ (Accessed: December 17, 2023).
- Weems, C. F., Russell, J. D., Neill, E. L., & McCurdy, B. H. (2019). Annual Research Review: Pediatric posttraumatic stress disorder from a neurodevelopmental network perspective. Journal of Child Psychology and Psychiatry, and Allied Disciplines, 60(4), 395–408. https://doi.org/10.1111/jcpp.12996
- Wei, L., Simen, A., Mane, S., & Kaffman, A. (2012). Early life stress inhibits expression of a novel innate immune pathway in the developing hippocampus. Neuropsychopharmacology, 37(2), 567–580. https://doi.org/10.1038/npp.2011.239
- Wiley, J. F., Gruenewald, T. L., Karlamangla, A. S., & Seeman, T. E. (2016). Modeling multisystem physiological dysregulation. Psychosomatic Medicine, 78(3), 290–301. https://doi.org/10.1097/PSY.0000000000000288
- Wilhelmsen, K., Strand, L. I., Nordahl, S. H. G., Eide, G. E., & Ljunggren, A. E. (2008). Psychometric properties of the Vertigo symptom scale – short form. BMC Ear, Nose, and Throat Disorders, 8(1), 2. https://doi.org/10.1186/1472-6815-8-2
- Williams, L. M. (2016). Precision psychiatry: A neural circuit taxonomy for depression and anxiety. The Lancet Psychiatry, 3(5), 472–480. https://doi.org/10.1016/S2215-0366(15)00579-9.
- Wilson, G., Farrell, D., Barron, I., Hutchins, J., Whybrow, D., & Kiernan, M. D. (2018). The use of eye-movement desensitization reprocessing (EMDR) therapy in treating post-traumatic stress disorder-a systematic narrative review. Frontiers in Psychology, 9, 923. https://doi.org/10.3389/fpsyg.2018.00923
- Wiss, D. A., Avena, N., & Gold, M. (2020). Food addiction and psychosocial adversity: Biological embedding, contextual factors, and public health implications. Nutrients, 12(11), 3521. https://doi.org/10.3390/nu12113521
- Wright, R. J. (2011). Epidemiology of stress and asthma: From constricting communities and fragile families to epigenetics. Immunology and Allergy Clinics of North America, 31(1), 19–39. https://doi.org/10.1016/j.iac.2010.09.011
- Yam, K.-Y., Naninck, E. F. G., Schmidt, M. V., Lucassen, P. J., & Korosi, A. (2015). Early-life adversity programs emotional functions and the neuroendocrine stress system: The contribution of nutrition, metabolic hormones and epigenetic mechanisms. Stress, 18(3), 328–342. https://doi.org/10.3109/10253890.2015.1064890
- Younger, J., Parkitny, L., & McLain, D. (2014). The use of low-dose naltrexone (LDN) as a novel anti-inflammatory treatment for chronic pain. Clinical Rheumatology, 33(4), 451–459. https://doi.org/10.1007/s10067-014-2517-2
- Young, E. S., Griskevicius, V., Simpson, J. A., Waters, T. E. A., & Mittal, C. (2018). Can an unpredictable childhood environment enhance working memory? Testing the sensitized-specialization hypothesis. Journal of Personality and Social Psychology, 114(6), 891–908. https://doi.org/10.1037/pspi0000124
- Young, S. G., Jones, I. F., & Claypool, H. M. (2016). Stimulus threat and exposure context modulate the effect of mere exposure on approach behaviors. Frontiers in Psychology, 7, 1881. https://doi.org/10.3389/fpsyg.2016.01881
- Young-Southward, G., Svelnys, C., Gajwani, R., Bosquet Enlow, M., & Minnis, H. (2020). Child maltreatment, autonomic nervous system responsivity, and psychopathology: Current state of the literature and future directions. Child Maltreatment, 25(1), 3–19. https://doi.org/10.1177/1077559519848497
- Yousufzai, M. I. U. A., Harmatz, E. S., Shah, M., Malik, M. O., & Goosens, K. A. (2018). Ghrelin is a persistent biomarker for chronic stress exposure in adolescent rats and humans. Translational Psychiatry, 8(1), 74. https://doi.org/10.1038/s41398-018-0135-5
- Zamyslowska-Szmytke, E., Politanski, P., & Jozefowicz-Korczynska, M. (2021). Dizziness handicap inventory in clinical evaluation of dizzy patients. International Journal of Environmental Research and Public Health, 18(5), 2210. https://doi.org/10.3390/ijerph18052210
- Zelazo, P. D. (2020). Executive function and psychopathology: A neurodevelopmental perspective. Annual Review of Clinical Psychology, 16(1), 431–454. https://doi.org/10.1146/annurev-clinpsy-072319-024242
- Zhang, W., Kaldewaij, R., Hashemi, M. M., Koch, S. B. J., Smit, A., van Ast, V. A., Beckmann, C. F., Klumpers, F., & Roelofs, K. (2022). Acute-stress-induced change in salience network coupling prospectively predicts post-trauma symptom development. Translational Psychiatry, 12(1), 63. https://doi.org/10.1038/s41398-022-01798-0
- Zhang, Y., Ren, R., Sanford, L. D., Yang, L., Zhou, J., Zhang, J., Wing, Y.-K., Shi, J., Lu, L., & Tang, X. (2019). Sleep in posttraumatic stress disorder: A systematic review and meta-analysis of polysomnographic findings. Sleep Medicine Reviews, 48, 101210. https://doi.org/10.1016/j.smrv.2019.08.004
- Zhou, H.-Y., Chen, S.-R., & Pan, H.-L. (2011). Targeting N-methyl-D-aspartate receptors for treatment of neuropathic pain. Expert Review of Clinical Pharmacology, 4(3), 379–388. https://doi.org/10.1586/ecp.11.17
- Zitkovsky, E. K., Daniels, T. E., & Tyrka, A. R. (2021). Mitochondria and early-life adversity. Mitochondrion, 57, 213–221. https://doi.org/10.1016/j.mito.2021.01.005
- Zygmunt, A., & Stanczyk, J. (2010). Methods of evaluation of autonomic nervous system function. Archives of Medical Science, 6(1), 11–18. https://doi.org/10.5114/aoms.2010.13500