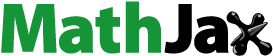
Abstract
The contamination by W impurity originating from dust released from Plasma Facing Components (PFCs) is a crucial issue of ITER and future reactors, expected to be fitted with an all-W first wall. In all existing experiments, information on dust pollution is obtained from post-mortem analysis and the data provided are accumulated over many discharges, and generally no shot-by-shot analysis is available. Real-time detection of W dust ‘footprints’ in the form of electrostatic waves can be useful to monitor edge and
pedestal evolution in high performance tokamaks.
1. Introduction
During operation of tokamaks with tungsten wall a sudden inflow of micrometric (m) W dust released randomly by the divertor tiles and nearby Plasma Facing Components (PFC) often occurs (Citation1). An amount of
is considered a lethal pollution for the discharge (Citation2), and it is therefore important to conceive a number of methods of detection of dust penetration in the scrape off layer (SOL) and of its effects on the profiles pedestals, in H mode regimes. Here the attention is focussed on the outer layers of the SOL, where density and temperature are significantly lower than in the bulk plasma, to investigate possible, detectable, dust induced perturbations. The results of the calculations presented in Figure 1 of ref. (Citation2) show that individual trajectories of the dust released from a certain tile of the divertor louvre are essentially ballistic with a dispersion within a cone defined by angular width
and height
, and axis parallel to the injection velocity
. The SOL volume spanned by these incoming projectiles, eventually ablated, is the ‘interaction volume’ much smaller than the full torus volume, and therefore the local dust density is relatively high. The information gathered on dust propagation in the peripheral tokamak divertor plasma, suggests the possible transient destabilisation of electrostatic waves as a kind of ‘pebble in a pond’ effect perturbing the charge balance in a limited plasma volume.
Dust ablation in-flight along trajectories that are essentially transverse to the magnetic surfaces, releases W atoms that become partially and eventually totally ionised by interaction with hot ambient plasma electrons. On time scales of radius reduction by thermodynamic processes (Citation2), dust particles deposition perturbs the background electrons profile, and also contaminates the plasma with a fraction of W ions. In such conditions, the condition of quasineutrality can be written as:
(1)
(1) where account is taken of ablation subtracting
atoms from the charged dust density
, leading to a modification of the Havnes parameter,
. As a side effect of altered quasi-neutrality, electrostatic waves can be excited (Citation3, Citation4), propagating obliquely with respect to the magnetic field. Such perturbations could contribute to the dust diagnostics in the outer plasma edge, monitored by reflectometric techniques or edge probes.
1.1. Formulation of the problem
We consider a very simplified scenario of a ‘collimated beam’ of W dust, interacting linearly with outer layers of tenuous SOL plasma, where (reciprocating) probes can be used. The density of highly-charged dust grains in the SOL ‘interaction volume’ can be locally significant. We assume a local Cartesian geometry and a magnetised thermal fluid plasma: . Electrons and ions are magnetised and the dust ballistic component is unmagnetised. The plasma species zero order fluid velocities are:
In the basic case where electrons and ions are magnetised, and dust unmagnetised, the frequency ordering is:
(2)
(2) where
and
are the ion and electron cyclotron frequencies and (local) thermal velocities, respectively. As a realistic benchmark scenario we consider, the values of the SOL density at the separatrix Σ, reported in (Citation2) for JET D and T discharges:
for a Deuterium pulse. The far SOL at
has a collisionality in the range
. Therefore the region of interest for the present problem is collisional. The two-fluid momentum equations, the continuity and Poisson equations, are written for harmonic perturbations
. Under the ordering conditions (Equation2
(2)
(2) ) lead to the expressions of the susceptibilities and the electrostatic Dispersion relation:
(3)
(3) The linear analysis predicts onset of Ion–dust streaming instability for realistic conditions in JET. The modelling results (see Table), show that the instability growth time is shorter than the average flight time
, over a wide range of propagation angle. Therefore the destabilised waves could be observed by edge probes or identified in spectrograms of plasma edge reflectometry (Citation5) (Figures and ).
Figure 1. Normalized electrostatic (acoustic) wave frequency (left) vs.
and growth rate (right)
, for various propagation angles. The plasma SOL data considered are:
,
,
;
eV,
,
m;
eV,
,
,
m;
,
.

Figure 2. Real (solid) and imaginary
(dashed) parts of the dispersion equation solutions vs.
, for propagation angle
.(left)- Scan of Havnes parameter: P
; (right)- Scan of
m/s.

Table
2. Nonlinear dynamics
The linearly unstable ion dust waves, may evolve into a nonlinear regime. An instructive analysis is performed with method of the Sagdeev pseudo-potential, adapted to the specific context described in the previous sections. Poisson's equation is expressed through the charged species densities obtained from the nonlinear fluid equations of motion cast in dimensionless form, and after an affine transformation of coordinates: ,
,
,
,
.
(4)
(4) The r.h.s of Equations (Equation1
(1)
(1) ) and (Equation4
(4)
(4) ) represents effectively a four species plasma (Citation4).
Multiplication of Poisson equation by and integration with the appropriate boundary conditions, yields the elegant form of the Sagdeev pseudo-potential statement:
(5)
(5)
(6)
(6) The nonlinear waves compatible with the topology of such Sagdeev potential (
) are acoustic solitons (Figure ). The phase space plots (
vs χ) show the existence of periodic nonlinear soliton solutions, for the physical parameters considered (Figure ). It can be expected that ‘solitonic’ bursts of waves, in coincidence with beam-like dust inflow, can be detected by suitable edge probes or reflectometry.
Additional information
Funding
Notes on contributors
E. Lazzaro
E. Lazzaro is a theorist, former Director of ISTP CNR.
F. Causa
F. Causa is a plasma modelling researcher of ISTP CNR.
G. Gervasini
G. Gervasini is a plasma modelling senior researcher at ISTP CNR.
F. Ghezzi
F. Ghezzi is a plasma experimentalist at ISTP CNR.
References
- Flanagan, J.C.Y.; Sertoli, M.; Bacharis, M.; Matthews, G.F.; de Vries, P.C.; Widdowson, A; Coffey, I.H.; Arnoux, G.; Sieglin, B.; Brezinsek, S. et al. Plasma Phys. Control. Fusion 2015, 57, 014037.
- Lazzaro, E.; Causa, F.; Gervasini, G.; Ghezzi, F.; Borodin, D.; Borodkina, I.; Douai, D.; Huber, A.; Pawelec, E.; Solano, E. et al. Nucl. Fusion 2022, 62 126037.
- Quest, K.; Rosenberg, M; Levine, A. J. Plasma Phys. 2020, 86, 905860605.
- Kiana, R.B.; Mahdieha, M.H. Plasma Phys. Rep. 2022, 48, 211.
- Goncalves, B.; Varela, P.; Silva, A.; Silva, F.; Santos, J.; Ricardo, E.; Vale, A.; Luis, R.; Nietiadi, Y.; Malaquias, A. et al. Sensors 2023, 23, 3926.