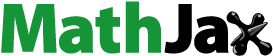
Abstract
A modeling of the transport processes in T-10 tokamak using Astra code for two sets of discharges having ECRH auxiliary heating is presented. Electric potential measurements using HIBP show that radial profiles are negative for ohmic heating but become positive for large heating powers. We first obtain and n profiles by adjusting transport coefficients and then try to obtain the radial
profiles by assuming three models for it. Results show good agreement for Ohmic and small ECRH power phases but not for large power where
indicating that other processes have to be included.
1. Introduction
Plasma profiles in toroidal plasmas are determined by various transport processes. Important information about the type of transport (neoclassical and anomalous) can be gained by transport simulations that aim to reproduce the experimental profiles. One of the quantities that characterize the plasma confinement is the radial electric field since it usually is related to some kind of confinement improvement. However, tokamaks and stellarators are normally subjected to different mechanisms of electric field generation due to their different symmetry properties. While tokamak neoclassical transport is, on a first approximation, intrinsically ambipolar, the non-axisymmetry of stellarators gives rise to unequal ion and electron transport, thus leading to the appearance of an ambipolar electric field. Experimentally, plasma electrostatic potential profiles can be measured, thus allowing to obtain radial electric field profiles . In the TJ-II stellarator, for instance, studies of ambipolar
profiles have been done based on neoclassical simulations of transport (Citation1). In these studies, good agreement with the shape of actual profiles was obtained although quantitative match was not achieved. On the other hand, the measurements of
obtained in tokamaks such as T-10 have shown properties similar to those in TJ-II discharges; namely negative potential in the entire column for Ohmic heating which gradually turns positive at large radii when auxiliary heating (ECRH) is increased.
Analyses of TJ-II discharges have shown that the main parameter determining profiles is plasma collsionality. In neoclassical simulations, electron diffusivity dominates at low collisionality thus leading to the ion root in the ambipolar condition, meaning
, but for higher collisionality ion diffusivity is dominant leading to the electron root (
) (Citation1). In T-10, the origin of
is expected to change since ambipolar contribution is very small. Here we try to understand the physical processes that determine the observed radial electric field profiles in T-10 in comparison with TJ-II. In particular, we focus on the effect of ECRH power increase in shaping these fields and changing their sign.
2. TJ-II and T-10 discharges
The TJ-II heliac at CIEMAT in Spain and the T-10 tokamak T-10 at the Kurchatov Institute in Moscow have very similar properties with dimensions and fields in the same range. T-10 has ,
, B = 1.5−2.5 T, operates with Ohmic heating and auxiliary ECRH. TJ-II has
,
, B = 1 T and uses ECRH to initiate and NBI heating. Both have Heavy Ion Beam Probe (HIBP) diagnostics to measure
, which is an important piece of equipment. It allows non-invasive measurements of inner plasma by injecting an energetic beam of Cs ions. Density and electron temperature can also be measured, which are in agreement with measurements of Langmuir probes in the edge region.
In TJ-II, measured electric potential radial profiles are simulated from the ambipolarity condition for particle flux using different models: Kovrizhnykh, Beidler, Shaing and kinetic simulations with DKES code (Citation1). The results for three different densities compared with experimental profiles (marked with symbols) are shown in Figure . There is qualitative agreement reflecting the negative
for high density becoming positive for lower densities.
On the other hand, T-10 has negative ϕ and values in ohmically heated discharges, but when ECRH is applied ϕ becomes less negative and may turn to positive (ion root). To study the situation for T-10, two well-diagnosed discharges were analyzed, shown in Figure . The transport mechanisms were explored to determine their relevance to
. Different regimes are extracted from them according to heating power (ECRH), which determines
, marked in the figures.
2.1. Transport simulations with Astra
Astra code was used for transport simulations. Transport coefficients are provided trying different models for diffusion D and heat conductivities . First, experimental density and temperature profiles are fit in the best possible way, combining collisional and anomalous transport coefficients, with Ohmic heating:
, one of them dominating near the center and the other near the edge.
Models that worked for OH also gave reasonable profiles for auxiliary heating. They had: For neoclassical (collisional) transport, an interpolation of banana, plateau and PS by Galeev–Sagdeev for species j = i, e For the anomalous coefficients, we used electromagnetic modes with trapped electrons for D, a semi-phenomenological model found in Asdex for
and Alacator scaling for
:
3. Models for radial electric field
Once these profiles are obtained we turn to the important issue of the origin of the radial electric field. In principle, ion momentum equation gives (Method 1)
(1)
(1) determined from simulated
profiles and unknown
profile. The latter can be proposed and is the main factor in determining
profile shape. We used a shifted Gaussian for
to match the observed
. But this lacks a physical basis. The sign reversal can only happen by an arbitrary inversion of poloidal rotation velocity.
As a second method to get is to assume non-ambipolar transport arising from the B-field ripple taken from (Citation2), just as in a stellarator.
is obtained through ambipolarity condition
solved with an Astra module. Values thus obtained are small but sign reversal may arise by trapped electrons transport when collisionality decreases.
A third model for getting is a combination of the previous two models. Taking the function
the velocity is determined from ambipolarity
and then used back in ion momentum equation (Equation1
(1)
(1) ) as
. This gives more appropriate results and provides better justification for simulation.
3.1. 
simulation results
For method 1, fit is good for Ohmic regime but not for ECRH unless is modified. For method 2 sign reversal for large ECR power is obtained but very small (small ripple). For method 3 ion root results near edge and
is larger. This is seen in Figure . Not a good match; there must be other effects not included here determining sign change.
4. Conclusion
Transport simulations of TJ-II and T-10 discharges are in good agreement when comparing with experimental profiles of and
, using a combination of neoclassical and anomalous transport models, chosen conveniently. In T-10, Ohmic and ECRH regimes have good fits. Radial electric field is well modeled in TJ-II and in T-10 for ohmic regimes with the three methods used, but is better for combined model. When
profiles become positive for large ECRH powers, the simulation fails to reproduce them, indicative of the presence of other effects not accounted for in the models. Possible candidates are contribution of suprathermal electrons created by EC waves. It has been showed that this effect is important in TJ-II case (Citation3).
Acknowledgments
We thank A.V. Melnikov for providing the data of T-10 shown here. Collaboration with D. Lopez-Bruna and C. Gutierrez-Tapia in these work is acknowledged.
Disclosure statement
No potential conflict of interest was reported by the author(s).
Additional information
Funding
Notes on contributors
J.J. Martinell
J.J. Martinell has a BS and MS from National Autonomous University of Mexico (UNAM) and a PhD from Massachusetts Institute of Technology, USA. He is a professor in Nuclear Science Institute at UNAM.
H.U. Lara-Martínez
H.U. Lara-Martinez has a BS from UNAM and is a MS student at University of York, UK.
References
- Gutierrez-Tapia, C.; Martinell, J.J.; López-Bruna, D.; Melnikov, A.V.; Eliseev, L.; Rodríguez, C.; Ochando, M.A.; Castejón, F.; García, J.; van Milligen, B.P.; Fontdecaba, J.M. Plasma Phys. Control. Fus. 2015, 57, 115004.
- Melnikov, A.V.; Eliseev, L.G.; Perfilov, S.V.; Andreev, V.F.; Grashin, S.A.; Dyabilin, K.S.; Chudnovskiy, A.N.; Isaev, M. Yu.; Lysenko, S.E.; Mavrin, V.A.; Mikhailov, M.I.; Ryzhakov, D.V.; Shurygin, R.V.; Zenin, V.N. Nuclear Fus. 2013, 53, 093019.
- Martinell, J.J. Radiat. Eff. Defects Solids 2016, 171, 103–108.