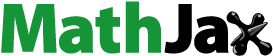
Abstract
Controlled tungsten (W) dust injection experiments have been performed in the STOR-M tokamak. Tokamak discharges were initiated after a known delay time with respect to the injection of W micro-spheres. The plasma parameters were detrimentally affected, proportional to an increased amount of dust within the plasma volume. High speed camera imaging of the injected dust revealed that the dust particles experience a strong force in the counter-plasma current direction. This force decreased for high dust number density due to the associated decline of plasma parameters.
1. Introduction
Tungsten (W) as a wall material has been extensively studied in many tokamaks, including JET, DIII-D, ASDEX Upgrade and WEST. It is chosen as a plasma-facing materials because of its many interesting properties, including: low sputter yield, high thermal conductivity, low thermal expansion coefficient, high melting point and low hydrogen retention. However, since W has a high atomic number Z, Bremsstrahlung radiation loss due to W impurity entering the plasma may be increased. The resulting cooling in the core region may result in hollow temperature profiles or even disruptions [Citation1, Citation2]. Additionally, tritium retention in W dust and the risk of explosion are potential operational and safety issues. In this article, preliminary experimental results of plasma-dust interactions in the STOR-M plasma are presented. For that purpose, a dust dispenser was used to inject well-calibrated spherical tungsten particles.
2. Experiment setup
STOR-M is a tokamak with an iron core and the following main parameters: R = 45 cm (major radius), a = 12 cm (minor radius), 30 kA (plasma current),
3 V (loop voltage), and
1 T (toroidal magnetic field). STOR-M discharges in this study are gas puff terminated after 40 ms. Figure (a) shows the cross-section of STOR-M, including the limiter and the vacuum vessel. A dust dispenser [Citation3] is mounted on the top of the vacuum vessel (z = 0) and the center of STOR-M is located at z = 37 cm below the dispenser. The dispenser injects
g of W micro-particles. The injected particle sizes range from
to
m with a most probable diameter of
m [Citation3]. Based on the dispenser calibration studies, the center of mass of the dust plume reaches the center of the vacuum chamber
280 ms after the dispenser's trigger. Note that the dispenser is a modified fast gas valve activated by a current pulse (
0.5 ms in duration) through an enclosed coil and injects hydrogen gas at the same time. The injected gas diffuses into the STOR-M vacuum vessel in
10 ms. In this experiment, the dust dispenser is triggered at time t = 0. After a chosen time delay
, the STOR-M discharge is initiated. The delay time
controls the number of tungsten particles present in the volume of the STOR-M chamber when the discharge starts. If the discharge starts before the dust dispenser, the delay time is defined as negative. In this case, no dust is present in the tokamak plasma, but the gas injected can affect the discharge. A Chronos 1.4 fast video camera working at 2156 frames per second (fps) is used to image the tungsten particle from a horizontal port and an Ocean Optics spectrometer looks at the plasma glow from a vertical port directly under the dust dispenser.
Figure 1. (a) Poloidal cross-section of the experimental dust injection setup in STOR-M. An example of a dispersed dust plumb with its center of mass reaching after a particular delay time with respect to dust injection is illustrated. (b) Characteristic emission spectrum. The observed emission lines include: Oxygen and Carbon impurities, Balmer series, and Tungsten. (c) STOR-M plasma current for various delay times.

3. Results
Figure (b) shows a typical emission spectrum of a STOR-M discharge containing W dust. The theoretical wavelengths of the impurity (C, O, W) line emissions and the hydrogen Balmer Series are marked in the spectrum (red for W, black for O and C, purple for H). Note the W emission peak at 400.9 nm. This characteristic W line emission has been observed in many other tokamaks.
Plasma parameters were recorded for various delay times corresponding to different dust number densities within the tokamak volume. Figure (c) shows plasma current () plotted for five different delay times and when no dust is injected. Without dust, a maximum current of approximately 20 kA is reached and the discharge is stable overall. For a long delay time
645 ms, the dust plume has already fallen through the tokamak but microparticles are still present due to W particles bouncing off the bottom of the vacuum vessel back into the plasma. These dusts lower the maximum plasma current and shorten its duration. The plasma duration for a discharge triggered at
645 ms after dust injection is roughly the same as without dust injection, though it takes longer to terminate. For
205 ms and
415 ms, the plasma behaves similarly as roughly the same amount of dust is found within the plasma volume, the difference being that the center-of-mass (CoM) of the dust plume is above the tokamak mid-plane for
205 ms and below the mid-plane for
415 ms. In both cases, dust induced a disruption after
25 ms. Lastly, the results for
285 ms and
305 ms are very similar, as in both cases, the dust plume CoM is located near the center of the plasma column. This results in the greatest reduction of plasma parameters and plasma disruption after
20 ms. Generally, plasma parameters are found to worsen as W dust number density within the plasma volume increases.
Many experiments have been conducted for different delay times. Figure shows measurements of the dust plume fraction within the plasma and some chosen plasma parameters for different delay times. Each point is the average of 10 shots with the error bar corresponding to the standard deviation. For delay times ranging from 200 ms to
450 ms, Figure (a) shows an increase in the W
line emission intensity. This result correlates perfectly with an increase of the calculated dust plume fraction within the plasma volume (
), shown in Figure (b). Indeed, calculation predicts that the falling dust plume starts entering the top of the STOR-M vacuum chamber after
200 ms (green line) and reaches the bottom of the vacuum chamber, where dust may bounce after
450 ms (orange line). Furthermore, as
increases, the plasma parameters appear to be negatively affected. Indeed, Figure (c) shows the plasma duration (
) decreases to a local minimum, corresponding to the highest
at
300 ms, and then increases again as
decreases. Figure (d) shows that the peak
follows the same trend as plasma duration, though less pronounced. However, plasma parameters are also affected by delay times when no dust is supposed to be present within the plasma volume, thus pointing to other possible mechanisms.
Figure 2. Dust plume fraction in the plasma volume and STOR-M discharge parameters as a function of the delay time: (a) W line emission counts, (b) ratio of dust within STOR-M to total dust injected, (c) plasma duration, (d) peak (maximum) plasma current. Each point is an average over 10 shots with the error bar corresponding to the standard deviation. Four significant delay times are denoted:
delay time at which gas puffing starts to affect the discharge,
delay time at which gas puffing has the maximum effect,
delay time at which the dust plume enters the top of the plasma volume, and
) delay time at which the dust plume tail reaches the bottom of the vacuum vessel.

Dust injection inevitably results in a puff of hydrogen gas soon after triggering. It can have a strong effect on the plasma depending on . For delay times ranging from
ms (purple line) to
0 ms (blue line), the dust injector is triggered during the lifetime of the plasma. The puffed hydrogen gas is localized below the dispenser and causes early termination of the plasma. For delay times ranging from
to at least
, the dust dispenser is triggered before the plasma column is formed. As the time delay increases, the injected gas has more time to disperse and be pumped away, thus explaining the improvement of the plasma parameters in the interval
to
. Note that plasma parameters are affected by the excess hydrogen gas even when dust particles are present (i.e.
). For delay times
, a second reduction of
and
is observed, corresponding to W microparticles bouncing off the bottom of the vacuum vessel and re-entering the plasma volume. For delay times
850 ms, dust particles have settled down and the discharge is no longer affected.
Tungsten dust particles interacting with the STOR-M plasma reached very high temperatures and emitted visible light, allowing their direct visualization with the high speed camera. Regardless of the chosen delay time, the dust particles glowed and could be tracked even after the plasma had terminated (for up to 10 ms after plasma disruption). Dust particle trajectories for different delay times are shown in Figure ( 235 ms, 315 ms and 645 ms). For the shortest delay time
235 ms (Figure (a)), the dust plume CoM is above the tokamak midplane, and only
20% of the injected dust is present in the vacuum chamber. A strong acceleration on dust particles is observed, opposing the plasma current direction (in the same direction as the toroidal magnetic field). For the delay time
315 ms (Figure (b)), the dust plume CoM center is close to the center of the plasma column, and about 80% of the injected dust is present within the vacuum chamber. Many dust particle trajectories are seen, and the trajectories are not significantly affected by the plasma. For the longest delay time
645 ms (Figure (c)), the dust plume has passed through the vacuum chamber, and only particles which have bounced off the bottom of the vacuum vessel are present within the plasma. These particles experience a strong acceleration in the direction opposing the plasma current, as was the case for
235 ms. As the dust number density increases, the dust particles' toroidal acceleration is observed to decrease, consistent with the worsening of plasma parameters, leading to a reduction of the plasma-induced force acting on dust (likely ion-drag).
4. Conclusion
Controlled W dust injection experiments have been performed in STOR-M. Plasma parameters and fast camera images of dust particles within the plasma were measured for different delay times between dust injection and discharge trigger. A significant impact on plasma parameters was observed when the injected dust plume was within the vacuum chamber. Moreover, plasma parameters decline when increasing the dust number denisty in the plasma. However, hydrogen gas puffed by the dust injector is also observed to affect the discharge for short delay times. Dust trajectories were also found to be significantly impacted and depended on the dust number density and the dust position. In particular, a strong plasma-induced force acts on dust particles in the toroidal direction. The reduction of this force with increased dust number density in the discharge is consistent with the measured decline in plasma parameters.
Disclosure statement
No potential conflict of interest was reported by the author(s).
Additional information
Funding
Notes on contributors
N. Nelson
Mr. N. Nelson is a PhD student at the University of Saskatchewan since January 2021. He obtained his BSc in Engineering in 2015 and MSc in 2020 from the University of Saskatchewan. He has received a Mitacs Globalink Research Award and will work at Aix-Marseille and CEA Cadarache in 2022 for six months. Mr. Nathan Nelson is an experimental plasma physicist. His current research interests are tokamak dust studies, plasma wall interactions and plasma facing component analysis.
R. Davies
Mr. R. Davies is a BSc student at McGill University, Montreal since September 2021. He received an NSERC USRA to work at the University of Saskatchewan for four months in the summer of 2023. His research interests are in experimental plasma physics, particularly in tokamak plasmas and dust transport.
L. Couëdel
Dr. L. Couëdel is a professor at the University of Saskatchewan (USask), Saskatoon since March 2018. He obtained his PhD from the University of Sydney, Australia in 2008. He was a postdoctoral fellow at the Max-Planck Institute for extraterrestrial Physics in the group of Professor Gregor Morfill (2009-2011). Prior to joining the USask, Dr. Couedel was a CNRS researcher in the PIIM laboratory at Aix-Marseille University (2011-2018). Dr. Couedel is an experimental plasma physicist. His current research interests are complex (dusty) plasmas (especially nanoparticle growth in low temperature plasmas (magnetized and unmagnetized), dust in tokamaks and instabilities in complex plasma crystals) and low temperature plasma diagnostics (especially sheath diagnostics).
C. Xiao
Dr. C. Xiao received his BSc (1982) and MSc (1984) degrees from the University of Science and Technology of China, Hefei, China and his PhD (Dr.rer.nat., 1990) degree from the Ruhr-University Bochum, Germany. He is a professor in the Department of Physics and Engineering Physics, University of Saskatchewan, Canada. His current research interests include the physics, engineering, and application aspects related to plasma diagnostics and plasma devices such as tokamak, reversed field pinch, compact torus injector, and dense plasma focus. He is responsible for operation of and research on the STOR-M tokamak and the University of Saskatchewan Compact Torus Injector.
References
- Lazzaro, E; Causa, F; Gervasini, G; Ghezzi, F; Borodin, D; Borodkina, I; Douai, D; Huber, A; Pawelec, E; Solano, E et al. Simulated Effects of W Dust Ablation and Deposition on the Pedestal Edge in Jet D and Dt Experiments. Nucl. Fus. 2022, 62 (12), 126037.
- Ostuni, V; Morales, J; Artaud, J-F; Bourdelle, C; Manas, P; Fedorczak, N; Dumont, R; Goniche, M; Maget, P; Peysson, Y et al. Core Radiative Collapse Characterisation and Integrated Modelling in West Plasmas. Nuclear Fusion 2022, 62 (10), 106034.
- Nelson, N; Couëdel, L; Xiao, C Design and Characterization of a Dust Dispenser for Tungsten Dust Injection Experiments in the STOR-M Tokamak. Radiat. Eff. Defects Solids 2022, 177 (1-2), 181–197.