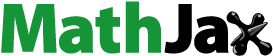
ABSTRACT
Purpose
This study intends to investigate the correlation between blood pressure variability (BPV) levels and the use of optical coherence tomography (OCT) and optical coherence tomography angiography (OCTA) to measure retinal microvasculature in hypertensive patients.
Methods
All individuals in the study had 24-hour ambulatory blood pressure monitoring and bilateral OCT and OCTA exams, and only data from the right eye were analyzed statistically.
Results
The study included 170 individuals, with 60 in the control group. The experimental group was separated into two groups based on the average real variability (ARV) median, with 55 in the low ARV group and 55 in the high ARV group. The mean thicknesses of the Retinal Nerve Fiber Layer (RNFL), internal limiting membrane-retinal pigment epithelial cell layer (ILM-RPE), vessel density (VD), and perfusion density (PD) in the high-ARV group were substantially lower in the low-ARV and control groups (p<0.05). Multiple linear regression analysis revealed that disease duration, age, and 24 h diastolic standard deviation all affected RNFL mean thickness (p<0.05). VD and PD were influenced by disease duration, systolic-ARV, daytime systolic blood pressure, intraocular pressure(IOP), and best-corrected visual acuity (BCVA) (p<0.05). And the change in VD was connected to best-corrected visual acuity.
Conclusion
Hypertensive retinopathy is related to BPV. In clinical practice, we can assess the degree of BPV and retinopathy in hypertensive patients to track the progression of hypertension-mediated organ damage (HMOD). Correction of BPV may help treat or postpone the progression of HOMD.
Introduction
With high morbidity and death rates worldwide, hypertension is one of the most prevalent chronic disorders and a significant risk factor for cardiovascular disease (Citation1). Elevated blood pressure can cause structural or functional abnormalities in the brain, heart, kidneys, central and peripheral arteries, eyes, and the organs it serves (Citation2).
According to the length of the observation period, there are two types of BPV: short-time BPV and long-time BPV. BPV measures how much blood pressure fluctuates over a specific period and has been linked to several conditions, including carotid intima-media thickness (Citation3), renal disease (Citation4), cardiovascular disease (Citation5), and cerebrovascular disease (Citation6).
Currently, short-term BPV is assessed for clinical investigations and the assessment of antihypertensive medication using 24-hour ambulatory blood pressure monitoring (24 h-ABPM). There are various ways to measure BPV, and the methods and sampling frequency determine the clinical and prognostic importance of BPV. Although the standard deviation (SD) and coefficient of variation (CV) are frequently employed to represent BPV, they both have drawbacks and are unable to accurately capture many of its properties. Additionally, they have a limited predictive value for target organ damage. To improve the predictive power of 24-hour BPV, Mena et al (Citation7) proposed the average true variability (ARV) index. This method believes the ARV index to have more predictive power than SD and is more appropriate for identifying treatment measures intended to control BPV because it focuses on changes occurring over shorter periods.
One of the frequent target organ damages in hypertension patients is hypertensive retinopathy (HTNR), and the severity of HTNR is linked to mortality (Citation8,Citation9). As a result, clinical purposes must evaluate retinal alterations in hypertension patients, however, HTNR is frequently disregarded in clinical applications. According to some theories (Citation10,Citation11), the microvascular system of the retina shares several physiological and morphological traits with the brain and coronary microcirculation. The retinal vascular system alters in several ways as a result of elevated blood pressure. Small arterial alterations, such as small artery stenosis, small artery wall clouding, and arteriovenous scratching, are separated from retinopathy, which manifests as retinal hemorrhage, microaneurysms, cotton patches, and hard exudates (Citation12). These variants serve as the foundation for the Keith-Wagener-Baker (Citation13) and Mitchell-Wong (Citation14) grading systems. However, there are certain subjective restrictions on the Keith-Wagener-Baker grading and the Mitchell-Wong grading, particularly in the early evaluation of HTNR. The introduction of OCT and OCTA techniques has transformed retinal imaging and introduced pertinent factors to accurately measure changes in the layers of the retina (Citation15), promising to eventually make up for HTNR grading.
The evaluation of patients’ HTNR is frequently overlooked in clinical practice, and it is still unknown whether blood pressure variability indicators in hypertensive patients affect their development of HTNR. It is also unknown whether hypertensive patients’ OCTA measurements correlate with blood pressure variability. In this work, we looked at how different blood pressure variability characteristics in hypertension individuals related to quantitative OCT and OCTA parameters to offer an additional theoretical framework for evaluating retinal damage in hypertension individuals.
Materials and methods
Study population
The study involved a total of 122 hypertensive subjects and 63 healthy volunteers who were recruited from the departments of general medicine and hypertension at the First Hospital of Shanxi Medical University between December 2021 and June 2022. 110 cases were included after low-quality pictures (12 eyes in the hypertension group and 3 eyes in the control group) were eliminated based on the nadir criteria. The control group consisted of healthy volunteers. The case group was split into two groups based on the median ARV, with 55 cases each in the low ARV group and the high ARV group. Patients were chosen if they met the following minimum requirements: (1) a primary hypertension diagnosis according to the 2020 International Society of Hypertension Global Hypertension Practice Guidelines (Citation2); blood pressure readings of≥140/90 mmHg obtained from three office measurements; (2) age between 18 and 80; (3) daily home blood pressure monitoring; (4) degree of lens clouding not affecting vision. Exclusion criteria include: (1) having glaucoma, retinal diseases, or other eye conditions that could affect the results of the analysis; (2) having diabetes (pre-diabetes), coronary heart disease, cerebrovascular disease, autoimmune diseases, hematological diseases, tumors, or other systemic conditions; (3) having a recent history of taking hormonal drugs, antineoplastic drugs, psychiatric medications, or other special medications.
All patients underwent 24 h-ABPM, slit-lamp biomicroscopy, BCVA, IOP, OCT, and OCTA.
The Declaration of Helsinki was followed in this observational, cross-sectional investigation. The First Hospital of Shanxi Medical University’s review board approved the study’s ethical components, and all qualified participants provided their written informed permission.
24 h-ABPM
All subjects underwent 24 hours of ABPM during each day of activity. The appropriate cuff was chosen based on the subject’s arm size and was placed on the non-dominant arm. During the measurement, subjects were instructed to keep their arm still. Ambulatory blood pressure was recorded automatically using an ambulatory blood pressure recorder (Welch Allyn, ABPM7100). Blood pressure was measured automatically every 30 minutes from 7:00 to 22:59 during the day, and every hour from 23:00 to 6:59 at night.
Daytime, nighttime, and over 24 hours systolic and diastolic blood pressure, the standard deviation of systolic blood pressure and diastolic blood pressure, the coefficient of variation of systolic blood pressure and diastolic blood pressure, and mean ARV of systolic blood pressure and diastolic blood pressure were used to calculate BPV. SD, CV, and ARV were common time-domain BPV indicators for short-term (24-hour) BPV analysis.
The SD were using the following formula:
where N is the number of valid BP measurements.
The CV were using the following formula:
ARV shows the average of the absolute differences between consecutive blood pressure measurements and were using the following formula:
where N represents the total number of valid BP measurements, and BP k + 1 and BP k represents 2 consecutive BP measurements.
Subjects had a good record of technical quality (at least 70% of valid readings over 24 hours, at least 20 valid readings while awake and at least two valid readings per hour; at least seven valid readings while asleep (at least one valid reading per hour), meeting the minimum requirements recommended by the European Society of Hypertension (Citation2).
Optical coherence tomography (OCT) and optical coherence tomography angiography (OCTA) measurement
OCT: All study participants underwent high-resolution blood flow OCT exams (Zeiss Meditec, Cirrus HD-OCT 5000) in a darkened room with their pupils uncontracted. They were also instructed to rest their jaws and foreheads on a jaw rest and forehead fixation device and to adjust the table height to their comfort. To obtain a clearer image, the subject was instructed to look at the internal fixation cursor and correct it based on the refractive state. The scanning mode was set to Macular 512 × 128, which determined the ILM-RPE mean volume thickness, volume, and central subregion thickness; the mode was set to Optic Disc Cube 200 × 200, which determined the superior, inferior, nasal, temporal, and circumferential RNFL thickness and cup-to-disc ratio; and images with proper position and high image quality (signal intensity≥6) were screened for better acquisition of the above parameters.
OCTA: All patients were examined in a dark room using OCTA (Zeiss Meditec, HD-OCT 5000 with Angioplex) with the subjects’ pupils in their natural state. The subject was told to rest his lower jaw and forehead on a mandibular rest and forehead fixation device and to adjust the table height to maintain comfort. The scanning procedure included: angio-OCTA 6 × 6 mm scan. To reduce motion artifacts, ZeissOCTA employs real-time tracking technology. Only image signal intensities≥6 are allowed. Due to examination system limitations, only superficial retinal vessel density (from the inner boundary membrane to the inner plexiform layer) can be quantified, but not deep retinal vessel density. In this study, the metrics included in the analysis were: vascular density (VD) and perfusion density (PD) at 6 × 6 mm (including mean VD and mean perfusion density, as well as VD and perfusion density in the superior, inferior, nasal, and temporal aspects of the inner ring and in the superior, inferior, nasal, and temporal aspects of the outer ring) (). All hypertensive patients and volunteers included in the study had both eyes examined, and all data from the right eye were chosen for statistical analysis, with data from the left eye chosen for statistical inclusion if the quality of the data from the right eye was poor.
Figure 1. Optical coherence tomography angiography 6×6mm scan image centered on the optic disc. The diameters of the three concentric circles are 1, 3, and 6mm. The measurement tool (AngioPlex software, version 10.0; Carl Zeiss Meditec) provided (a) vessel density and (b) perfusion density measurements in individual subfields. The bold box shows the automatic quantitative measurements for an average of the inner ring, outer ring, and full area.

Statistical analysis
Statistical analyses were performed using SPSS version 26.0 (IBM Corporation, USA). For statistical analyses, BCVA values were transformed to the logarithm of the minimum angle of resolution (log MAR) values. One-way analysis of variance (ANOVA), Bonferroni test, and chi-square test was used to compare the clinical characteristics, OCT and OCTA parameters of each group. Pearson correlation was used to analyze the relationship between the correlates of blood pressure variability and OCT and OCTA parameters. Spearman’s rank correlation was used to analyze the relationship between grade variables such as gender and K-W-B classification and OCT and OCTA parameters. p < .05 was considered statistically significant.
Result
Patient characteristics
We recruited 122 hypertensive patients and 63 healthy volunteers. After eliminating low quality images (12 eyes in the hypertensive group and 3 eyes in the control group), the hypertensive patients were divided into 55 each in the low ARV group (ARV 11.50) and high ARV group (ARV>11.5) by the median systolic ARV, and 60 in the healthy control group, for a total of 170 subjects included in this study. The case and control groups were described as stated in . The low ARV group’s mean age was 51.71 ± 11.76 years, the high ARV group’s mean age was 58.89 ± 10.84 years, and the control group’s mean age was 54.87 ± 13.91 years (p 0.05). The mean duration of hypertension in the low ARV group was 8.41 ± 7.44 years and 8.86 ± 7.86 years in the high ARV group (p = .001). BMI in the low-ARV group was 25.81 ± 4.31, 24.95 ± 4.13 in the high-ARV group, and 23.79 ± 3.00 in the control group (p<0.05). The K-W-B classification differed significantly between the three groups (p = .001). Gender, smoking history, IOP, and BCVA did not differ significantly between the three groups.
Table 1. Demographics and clinical features of the study subjects.
ABPM measurements
The ABPM data are shown as the mean standard deviation in for the case and control groups. The 24-h BPV measures (BP, SD, CV), systolic ARV(SBP-ARV), and diastolic ARV (DBP-ARV) were demonstrated to be statistically significant (p<0.05) in all three groups. There was no statistically significant difference in the three groups’ daytime systolic blood pressure standard deviation (Day-SBPSD), nighttime diastolic blood pressure standard deviation (Night-DBPSD), or night diastolic blood pressure CV (Night-DBPCV). By the Bonferroni test, we found that SBP-ARV and DBP-ARV were significantly higher in the high-ARV group than in the low-ARV group and the control group (P = .001), but DBP-ARV in the low-ARV group was not significantly different from the control group (P = 1.000). Systolic and diastolic blood pressure were not significantly different between the high-ARV and low-ARV groups, but both were significantly higher than the control group (P = .001).
Table 2. ABPM parameters of all patients and controls.
OCT and OCTA measurements
The OCT and OCTA data for the case and control groups are expressed as mean ± standard deviation and are shown in . RNFL thickness, ILM-RPE mean volume thickness, superficial retinal vascular density (VD), and superficial retinal perfusion density (PD) were statistically significant among the three groups (p < .05). By the Bonferroni test, we found that the mean ILM-RPE volume thickness, VD, and PD in the high-ARV group were significantly lower than those in the low-ARV and control groups, with statistically significant differences (P < .05). The difference in mean RNFL thickness was statistically significant in the high-ARV group compared with the control group, but not compared with the low-ARV group (P = 1.000). The mean RNFL thickness, VD and PD in the center of the inner ring were also not significantly different in the low-ARV group compared with the control group (P>0.05).
Table 3. Comparison of RNFL thickness, ILM-RPE mean volume thickness, VD and PD among groups.
Association of blood pressure variability parameters and OCT parameters with OCTA parameters in hypertensive patients
In hypertension patients, systolic ARV was adversely connected to mean RNFL thickness, although diastolic ARV was not related to mean RNFL thickness illness duration. In hypertensive patients, both systolic and diastolic ARV were unaffected by ILM-RPE mean volume thickness. The duration of hypertension was adversely associated with the mean RNFL thickness and the mean ILM-RPE volume thickness. BCVA was negatively related to the mean RNFL thickness and the mean ILM-RPE volume thickness. Age was negatively related to RNFL mean thickness and ILM-RPE mean volume thickness. K-W-B grading was negatively correlated with RNFL thickness but not with ILM-RPE mean volume thickness. In hypertensive patients, SBP-ARV, DBP-ARV, disease duration, age, BCVA, and K-W-B classification were all negatively related to VD and PD (Supplementary Table S1).
Multivariate regression analysis of OCT parameters and OCTA parameters with baseline indexes and blood pressure variability
The results of multiple linear regression analysis with RNFL, ILM-RPE, VD, and PD as dependent variables and each index of the above baseline data, and each index of BPV as independent variables are shown in . The duration of hypertensive disease, age, and 24hDBPSD were all factors that influenced RNFL thickness (p<0.05). The mean volume thickness of ILM-RPE was significantly influenced by the duration of the hypertensive condition, 24hDBPCV (p<0.05). SBP-ARV, Day-SBP, IOP, and BCVA were all significant predictors of VD (p<0.05). The duration of hypertension, SBP-ARV, day-SBP, and intraocular pressure were significant predictors of PD (p<0.05).
Table 4. Multivariate regression analysis of OCT parameters and OCTA parameters with baseline indexes and blood pressure variability.
Discussion
In this study, we used the OCT and OCTA systems to investigate the predictive efficacy of multiple indicators of blood pressure variability for retinal injury in 110 hypertension patients and 60 normotensive controls. In this population-based case-control study, ARV was found to be inversely linked with mean RNFL thickness, VD, and PD, and systolic ARV was an influential factor for VD and PD in multiple linear regression analysis.
This study focuses on retinal degeneration, which is frequently overlooked in clinical hypertension patients. This study discovered that RNFL thickness, ILM-RPE mean volume thickness, VD, and PD were lower in patients with higher ARV than in those with lower ARV and in the healthy population. A meta-analysis of the predictive efficacy of multiple BPV metrics for HOMD and cardiovascular outcomes (Citation16) found that ARV was a better predictor of 24-hour BPV than other dispersion measurements such as SD and CV. Several earlier investigations have demonstrated a strong connection between high ARV and the existence of target organ damage in the heart, brain, and kidney (Citation3,Citation17,Citation18) as well as the incidence of cardiovascular events (Citation19,Citation20), with ARV being an independent predictor of damage in each target organ. However, evidence on the influence of ARV on ocular damage is limited, and our work demonstrates a substantial relationship between high ARV and retinal damage, making a significant contribution to this field of study.
BPV is a very complex life phenomenon that is influenced by several factors (Citation21). In the hypertensive setting, the kinetics of these fluctuations correlate with the severity of hypertension, the use of antihypertensive medications, comorbidities, treatment adherence, and the presence of HMOD. Although the regulatory mechanisms of BPV are unknown, the main influencing factors are the nervous system, endocrine system, renin-angiotensin-aldosterone system, antidiuretic hormone and other neuroendocrine mechanisms, reflex mechanisms (cardiorespiratory reflex), blood viscosity, arterial elasticity, mental status, emotional factors, behavioral factors (e.g., activity, sleep, posture), environmental factors (e.g., chronic exposure to high industrial pollution), lifestyle (exercise, dietary habits), disease, smoking, and drugs (Citation22). Several studies have shown that BPV can cause microvascular dysfunction and thus target organ damage and that high blood pressure and rapid fluctuations in blood pressure can cause changes in the tension and pressure of blood pressure in the vessel wall, resulting in endothelial dysfunction and structural vascular damage (Citation23). On the other hand, sudden reductions in blood pressure reductions in blood pressure, on the other hand, might result in decreased microvascular perfusion, and the microvascular beds of low vascular impedance organs (e.g., the microvasculature of the brain, eyes, and kidneys) may be especially vulnerable to these blood pressure variations (Citation24). Eto et al (Citation25) suggested that an increase in BPV could impair endothelial function and enhance neointima formation, which may lead to atherosclerosis, while Kikuya et al (Citation26) suggested that atherosclerosis may in turn increase BPV.
In terms of embryonic development, anatomical structure, and physiological function, the retinal microvasculature is very similar to organs such as the heart, brain, and kidney; thus, changes in its morphology and function can be linked to diseases of other organs. The retinal microvasculature is the only directly observable microvasculature in the human body, and studies have shown that changes in the retinal microvasculature correspond to pathological changes in small vessels in other organs (Citation27). Several studies have previously found a link between HTNR and the beginning of coronary heart disease (Citation28), congestive heart failure (Citation29), coronary mortality (Citation30), and cardiovascular mortality (Citation8). Retinal small artery and vein sizes measured using fundus photographs reveal that narrower retinal small arteries and broader small veins are linked with long-term mortality, risk of ischemic stroke, and risk of coronary heart disease in women. However, there are still limits to diagnosing HTNR with fundus photographs.
According to Jacqueline Chua et al’s research (Citation31), OCTA can identify retinal microvascular anomalies, which may point to systemic heart microcirculatory dysfunction. With reduced VD and PD in hypertensive patients as well as reduced VD and PD in patients with long-term illness and poorer blood pressure control, our findings are consistent with those of a meta-analysis (Citation32). In the central recess and paracentral region, VD values were found to be higher in individuals without a history of hypertension, lower in those with a recent diagnosis of hypertension (untreated), and lowest in those with uncontrolled hypertension (Citation33), indicating that VD is correlated with the severity of the disease. Patients with good blood pressure control had higher levels of VD in the deep macular vascular plexus when they were stratified in earlier investigations based on BP values (Citation34,Citation35). However, several investigations did not identify any differences in VD and PD between hypertension patients and healthy controls in the superficial vascular layer (Citation33–35) or the deep vascular layer (Citation36). According to Donati et al (Citation33), the deep vascular layer differs significantly from the superficial vascular layer in terms of structure, perfusion, and flow resistance, and there is no discernible difference in VD between the superficial vascular layer and healthy people. In contrast, Hua et al (Citation36) concluded that the deep vascular layer is more autoregulated compared to the superficial vascular layer in chronic hypertension to demonstrate the lack of difference in VD in the deep vascular layer between healthy subjects and hypertensive patients. The mechanisms leading to HTNR are unclear, and some studies point to microvascular abnormalities as a cause or consequence of HTNR, which may occur in two phases: a functional first phase caused by excessive (albeit reversible) microvascular constriction resulting in no perfusion; and a structural/anatomical phase caused by irreversible depletion exists even under maximal vasodilation (Citation37,Citation38). Ozlem Bursali et al (Citation39) concluded that hypertension disrupts vascular self-regulation in patients, making tissues vulnerable to ischemia. Endothelin-1 (ET-1) has high plasma levels in hypertensive patients, despite its important role in autoregulation (Citation40). ET-1 increased the cup-to-disc ratio and decreased retinal blood circulation in animal experiments (Citation41). Retinal ischemia and hypoxia eventually result in tortuous and distorted intraretinal vessels. Similar to the previous theory, we hypothesize that larger BPV is more likely to damage the endothelial structure and function of retinal vessels, making them ischemic and hypoxic, eventually leading to a decrease in VD and PD.
Although the link between different types of BPV and target organ damage is well established, the link between abnormal BPV and fundus changes is not. According to a recent French survey, 55% of hypertensive patients had three or more target organ damages, while 70% of patients had target organ damages assessed by cardiologists (Citation42). Current guidelines, however, only recommend screening for hypertensive retinopathy in high-risk patients (Citation2). In patients with LVH and renal impairment, Brahim Harbaoui et al (Citation43) justified additional evaluation of hypertensive retinopathy. Previously, a large cohort study (Citation44) confirmed mild hypertensive retinopathy (Keith-Wagener-Barker grades 1 and 2; assessed by retinal photography) as an independent risk factor for cardiovascular mortality. ARV has also been shown to be an independent predictor of damage to various target organs, and the novelty of our study is the discovery of a close relationship between ARV and retinopathy; thus, clinically assessing BPV with HTNR is reasonable.
There are several limitations to this study. First off, we had a tiny sample size and our study was only conducted in one institution. Second, retinal microcirculation may be impacted by antihypertensive medications and renal function (low estimated glomerular filtration rate levels) (Citation45,Citation46). We were unable to thoroughly research these factors because of the cross-sectional study. Third, due to the equipment, we were only able to evaluate the superficial retinal veins, leaving out the deep retinal vessels and choroidal capillaries.
Conclusion
In conclusion, we discovered that greater ARV was closely connected with decreased RNFL thickness in hypertension individuals as well as decreased VD and PD in the retinal macula. Systolic ARV was also demonstrated to be an independent risk factor for HTNR. The clinical evaluation of HMOD now uses HTNR less frequently, and additional research is required to demonstrate the reliability of the OCTA parameter as a marker of early HMOD and its potential use as a useful predictor of cardiovascular morbidity and death. Additionally, BPV correction could be a therapeutic objective to stop the advancement of HMOD.
Acknowledgments
We thank the individuals for participating in this research. We would also like to thank Dr. Chaoran Lv, who is responsible for the collection and quality control of the ophthalmology data.”
Disclosure statement
No potential conflict of interest was reported by the authors.
Additional information
Funding
References
- Kim HC, Jeon YW, Heo ST. Global impact of the 2017 American College of Cardiology/American Heart Association Hypertension Guidelines. Circulation. 2018;138(21):2312–9. doi:10.1161/CIRCULATIONAHA.118.036312.
- Unger T, Borghi C, Charchar F, Khan NA, Poulter NR, Prabhakaran D, Ramirez A, Schlaich M, Stergiou GS, Tomaszewski M, et al. 2020 International Society of Hypertension global hypertension practice guidelines. Hypertension. 2020 Jun;38(6):982–1004. doi:10.1097/HJH.0000000000002453.
- Xiong H, Wu D, Tian X, Lin WH, Li C, Zhang H, Cai Y, Zhang Y-T. The relationship between the 24 h blood pressure variability and carotid intima-media thickness: a compared study. Comput Math Methods Med. 2014;2014:303159. doi:10.1155/2014/303159.
- Park CH, Kim HW, Joo YS, Park JT, Chang TI, Yoo TH, Park SK, Chae D-W, Chung W, Kim Y-S, et al. on the behalf of the KNOW‐CKD (Korean Cohort Study for Outcomes in Patients With Chronic Kidney Disease) Investigators. Association between systolic blood pressure variability and major adverse cardiovascular events in Korean Patients with Chronic Kidney Disease: findings from KNOW-CKD. J Am Heart Assoc. 2022; 11(11):e025513. doi:10.1161/JAHA.122.025513.
- Triantafyllidi H, Benas D, Schoinas A, Birmpa D, Trivilou P, Varytimiadi E, Voutsinos D, Ikonomidis I. Hypertension-mediated organ damage regression associates with blood pressure variability improvement three years after successful treatment initiation in essential hypertension. J Clin Hypertens (Greenwich). 2021;23(6):1150–58. doi:10.1111/jch.14209.
- Gutteridge DS, Tully PJ, Ghezzi ES, Jamadar S, Smith AE, Commerford T, Keage HAD. Blood pressure variability and structural brain changes: a systematic review. J Hypertens. 2022;40(6):1060–70. doi:10.1097/HJH.0000000000003133.
- Mena L, Pintos S, Queipo NV, Aizpúrua JA, Maestre G, Sulbarán T. A reliable index for the prognostic significance of blood pressure variability. J Hypertens. 2005;23(3):505–11. doi:10.1097/01.hjh.0000160205.81652.5a.
- Wong TY, Klein R, Nieto FJ, Klein BE, Sharrett AR, Meuer SM, Hubbard LD, Tielsch JM. Retinal microvascular abnormalities and 10-year cardiovascular mortality: a population-based case-control study. Ophthalmology. 2003;110(5):933–40. doi:10.1016/S0161-6420(03)00084-8.
- McGeechan K, Liew G, Macaskill P, Irwig L, Klein R, Klein BE, Wang JJ, Mitchell P, Vingerling JR, de Jong PTVM, et al. Prediction of incident stroke events based on retinal vessel caliber: a systematic review and individual-participant meta-analysis. Am J Epidemiol. 2009;170(11):1323–32. doi:10.1093/aje/kwp306.
- Arnould L, Binquet C, Guenancia C, Alassane S, Kawasaki R, Daien V, Tzourio C, Kawasaki Y, Bourredjem A, Bron A, et al. Association between the retinal vascular network with Singapore “I” Vessel Assessment (SIVA) software, cardiovascular history and risk factors in the elderly: the Montrachet study, population-based study. PLoS One. 2018;13(4):e0194694. doi:10.1371/journal.pone.0194694.
- Arnould L, Guenancia C, Azemar A, Alan G, Pitois S, Bichat F, Zeller M, Gabrielle P-H, Bron AM, Creuzot-Garcher C, et al. The EYE-MI pilot study: a prospective acute coronary syndrome cohort evaluated with retinal optical coherence tomography angiography. Invest Ophthalmol Visual Sci. 2018;59(10):4299–306. doi:10.1167/iovs.18-24090.
- Shin YI, Nam KY, Lee WH, Ryu CK, Lim HB, Jo YJ, Kim J-Y. Peripapillary microvascular changes in patients with systemic hypertension: an optical coherence tomography angiography study. Sci Rep. 2020;10(1):6541. doi:10.1038/s41598-020-63603-6.
- Keith NM, Wagener HP, Barker NW. Some different types of essential hypertension: their course and prognosis.Am. J Med Sci. 1974;6:336–45. Doi:10.1097/00000441-197412000-00004.
- Wong TY, Mitchell P. Hypertensive retinopathy. N Engl J Med. 2004;351(22):2310–17. doi:10.1056/NEJMra032865.
- Kashani AH, Chen CL, Gahm JK, Zheng F, Richter GM, Rosenfeld PJ, Shi Y, Wang RK. Optical coherence tomography angiography: a comprehensive review of current methods and clinical applications. Prog Retin Eye Res. 2017;60:66–100. doi:10.1016/j.preteyeres.2017.07.002.
- Mena LJ, Felix VG, Melgarejo JD, Maestre GE. 24-hour blood pressure variability assessed by average real variability: a systematic review and meta-analysis. J Am Heart Assoc. 2017;6(10):e006895. doi:10.1161/JAHA.117.006895.
- Yamaguchi Y, Wada M, Sato H, Nagasawa H, Koyama S, Takahashi Y, Kawanami T, Kato T. Impact of ambulatory blood pressure variability on cerebral small vessel disease progression and cognitive decline in community-based elderly Japanese. Am J Hypertens. 2014;27(10):1257–67. doi:10.1093/ajh/hpu045.
- Mulè G, Calcaterra I, Costanzo M, Morreale M, D’Ignoto F, Castiglia A, Geraci G, Rabbiolo G, Vaccaro F, Cottone S. Average real variability of 24-h systolic blood pressure is associated with microalbuminuria in patients with primary hypertension. J Hum Hypertens. 2016;30(3):164–70. doi:10.1038/jhh.2015.66.
- Hsu PF, Cheng HM, Wu CH, Sung SH, Chuang SY, Lakatta EG, Yin FCP, Chou P, Chen C-H. High short-term blood pressure variability predicts long-term cardiovascular mortality in untreated hypertensives but not in normotensives. Am J Hypertens. 2016;29(7):806–13. doi:10.1093/ajh/hpw002.
- Hansen TW, Thijs L, Li Y, Boggia J, Kikuya M, Björklund-Bodegård K, Richart T, Ohkubo T, Jeppesen J, Torp-Pedersen C, et al. International Database on Ambulatory Blood Pressure in Relation to Cardiovascular Outcomes Investigators. Prognostic value of reading-to-reading blood pressure variability over 24 hours in 8938 subjects from 11 populations. Hypertension. 2010 Apr;55(4):1049–57. doi:10.1161/HYPERTENSIONAHA.109.140798.
- Parati G, Stergiou GS, Dolan E, Bilo G. Blood pressure variability: clinical relevance and application. J Clin Hypertens (Greenwich). 2018;20(7):1133–37. doi:10.1111/jch.13304.
- Middeke M. [Blood pressure variability]. Dtsch Med Wochenschr. 2011;136(46):2361–66. doi:10.1055/s-0031-1292052.
- Rothwell PM. Limitations of the usual blood-pressure hypothesis and importance of variability, instability, and episodic hypertension. Lancet. 2010;375(9718):938–48. doi:10.1016/S0140-6736(10)60309-1.
- Mitchell GF. Effects of central arterial aging on the structure and function of the peripheral vasculature: implications for end-organ damage. J Appl Physiol (1985). 2008;105(5):1652–60. doi:10.1152/japplphysiol.90549.2008.
- Eto M, Toba K, Akishita M, Kozaki K, Watanabe T, Kim S, HASHIMOTO M, SUDOH N, YOSHIZUMI M, OUCHI Y. Reduced endothelial vasomotor function and enhanced neointimal formation after vascular injury in a rat model of blood pressure lability. Hypertens Res. 2003;26(12):991–98. doi:10.1291/hypres.26.991.
- Kikuya M, Hozawa A, Ohokubo T, Tsuji I, Michimata M, Matsubara M, Ota M, Nagai K, Araki T, Satoh H, et al. Prognostic significance of blood pressure and heart rate variabilities: the Ohasama study. Hypertension. 2000;36(5):901–06. doi:10.1161/01.HYP.36.5.901.
- Tang Q, Zhang Y, Yang Z, Li S, Wu M, Guo Y, Zhao W, Yin C. Study on the interaction between the characteristics of retinal microangiopathy and risk factors for cerebral small vessel disease. Contrast Media Mol Imaging. 2022;2022:9505945. doi:10.1155/2022/9505945.
- Wong TY, Klein R, Sharrett AR, Duncan BB, Couper DJ, Tielsch JM, Klein BE, Hubbard LD. Retinal arteriolar narrowing and risk of coronary heart disease in men and women. The Atherosclerosis Risk in Communities Study. JAMA. 2002;287(9):1153–59. doi:10.1001/jama.287.9.1153.
- Wong TY, Rosamond W, Chang PP, Couper DJ, Sharrett AR, Hubbard LD, Folsom AR, Klein R. Retinopathy and risk of congestive heart failure. JAMA. 2005;293(1):63–69. doi:10.1001/jama.293.1.63.
- Liew G, Wong TY, Mitchell P, Cheung N, Wang JJ. Retinopathy predicts coronary heart disease mortality. Heart. 2009;95(5):391–94. doi:10.1136/hrt.2008.146670.
- Chua J, Le TT, Sim YC, Chye HY, Tan B, Yao X, Wong D, Ang BWY, Toh D-F, Lim H, et al. Relationship of quantitative retinal capillary network and myocardial remodeling in systemic hypertension. J Am Heart Assoc. 2022;11(6):e024226. doi:10.1161/JAHA.121.024226.
- Anjos R, Ferreira A, Barkoudah E, Claggett B, Abegão Pinto L, Miguel A. Application of optical coherence tomography angiography macular analysis for systemic hypertension. a systematic review and meta-analysis. Am J Hypertens. 2022;35(4):356–64. doi:10.1093/ajh/hpab172.
- Donati S, Maresca AM, Cattaneo J, Grossi A, Mazzola M, Caprani SM, Premoli L, Docchio F, Rizzoni D, Guasti L, et al. Optical coherence tomography angiography and arterial hypertension: a role in identifying subclinical microvascular damage? Eur J Ophthalmol. 2021;31(1):158–65. doi:10.1177/1120672119880390.
- Chua J, Chin CWL, Hong J, Chee ML, Le TT, Wong TY, Schmetterer L, Schmetterer L. Impact of hypertension on retinal capillary microvasculature using optical coherence tomographic angiography. J Hypertens. 2019;37(3):572–80. doi:10.1097/HJH.0000000000001916.
- Chua J, Chin CWL, Tan B, Wong SH, Devarajan K, Le TT, Ang M, Wong TY, Schmetterer L. Impact of systemic vascular risk factors on the choriocapillaris using optical coherence tomography angiography in patients with systemic hypertension. Sci Rep. 2019;9(1):5819. doi:10.1038/s41598-019-41917-4.
- Hua D, Xu Y, Zeng X, Yang N, Jiang M, Zhang X, Yang J, He T, Xing Y. Use of optical coherence tomography angiography for assessment of microvascular changes in the macula and optic nerve head in hypertensive patients without hypertensive retinopathy. Microvasc Res. 2020;129:103969. doi:10.1016/j.mvr.2019.103969.
- Triantafyllou A, Anyfanti P, Pyrpasopoulou A, Triantafyllou G, Aslanidis S, Douma S. Capillary rarefaction as an index for the microvascular assessment of hypertensive patients. Curr Hypertens Rep. 2015;17(5):33. doi:10.1007/s11906-015-0543-3.
- Prewitt RL, Chen II, Dowell R. Development of microvascular rarefaction in the spontaneously hypertensive rat. Am J Physiol. 1982;243(2):H243–51. doi:10.1152/ajpheart.1982.243.2.H243.
- Bursali Ö, Altintaş Ö, Ağir A, Yüksel N, Özkan B. Optical coherence tomography measurements in patients with systemic hypertension. Scott Med J. 2021;66(3):115–21. doi:10.1177/00369330211011175.
- Aflyatumova GN, Nigmatullina RR, Sadykova DI, Chibireva MD, Fugetto F, Serra R. Endothelin-1, nitric oxide, serotonin and high blood pressure in male adolescents. Vasc Health Risk Manag. 2018;14:213–23. doi:10.2147/VHRM.S170317.
- Luo X, Shen YM, Jiang MN, Lou XF, Shen Y. Ocular blood flow autoregulation mechanisms and methods. J Ophthalmol. 2015;2015:864871. Doi:10.1155/2015/864871.
- Rossignol P, Hosseini K, Tropeano AI, Fay R, Tsatsaris A, Guillemin F, Mounier-Vehier C. Target organ damage assessment in French hypertensive patients without established cardiovascular or renal disease: results of the PREVENT-A study. J Hypertens. 2013;31(1):177–85. doi:10.1097/HJH.0b013e32835a34bb.
- Harbaoui B, Courand PY, Defforges A, Khettab F, Milon H, Girerd N, Lantelme P. cumulative effects of several target organ damages in risk assessment in hypertension. Am J Hypertens. 2016;29(2):234–44. doi:10.1093/ajh/hpv098.
- Sairenchi T, Iso H, Yamagishi K, Irie F, Okubo Y, Gunji J, Muto T, Ota H. Mild retinopathy is a risk factor for cardiovascular mortality in Japanese with and without hypertension: the Ibaraki Prefectural Health Study. Circulation. 2011;124(23):2502–11. doi:10.1161/CIRCULATIONAHA.111.049965.
- Hughes AD, Stanton AV, Jabbar AS, Chapman N, Martinez-Perez ME, McG Thom SA. Effect of antihypertensive treatment on retinal microvascular changes in hypertension. J Hypertens. 2008;26(8):1703–07. doi:10.1097/HJH.0b013e328304b072.
- Bosch A, Scheppach JB, Harazny JM, Raff U, Eckardt KU, Schmieder RE, Schneider MP. Retinal capillary and arteriolar changes in patients with chronic kidney disease. Microvasc Res. 2018;118:121–27. doi:10.1016/j.mvr.2018.03.008.