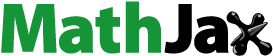
ABSTRACT
The usage of copper nanoparticles (CuNPs) is now acknowledged as a promising therapeutic agent in the detection and treatment of cancer. This study aimed to develop a sustainable method for synthesizing CuNPs using Pedalium murex L. and characterization by SEM, FTIR, XRD & UV-Vis methods. The produced CuNPs were primarily spherical and varied in shape, and the average dimension ranged between 20 and 50 nm. The aqueous fruit juice of Pedalium murex L. contained biomolecules that serve as capping and reducing agents for the production of CuNPs, according to the findings of FTIR analysis. Various assays were used to determine the fruit extract’s in vitro antioxidant activity. CuNPs exhibited dose-dependent cytotoxicity and apoptosis promotion in A549 cells. The findings demonstrated that biosynthesized CuNPs using Pedalium murex L. fruit juice have anti-cancer properties and can be used in lung cancer treatment, particularly for early-stage intervention.
Introduction
Nanotechnology is one the most lively field in the recent advancement of materials science research [Citation1,Citation2]. It is a promising extent of research with numerous applications in the fields of biology and medicine. Based on specific potentials including size, dissemination, and morphology, nanoparticles have entirely novel and enhanced possessions. The expansion of sustainable, eco-friendly methods for the synthesis of nanoparticles with environmental benign is a crucial aspects of nanotechnology, which is also known as green nanotechnology [Citation3]. Metallic nanoparticles have several uses because of their versatility, including targeted drug delivery, cancer therapy, wastewater treatment, DNA analysis, optoelectronics, solar power production, antibacterial agents and biosensors and catalysis [Citation4–10].
Metallic nanoparticles are often synthesized using various physical and chemical practices. The physical methods include laser ablation [Citation11], spark discharge [Citation12], and pyrolysis [Citation13]. The chemical methods are electrochemical reaction [Citation14], Reduction of chemicals [Citation15], solution irradiation [Citation16] and others. The foremost process involved in chemical blend is the reduction of Cu+ ions to Cu NPs. While the physical methods are time-consuming and labour-intensive, the chemical processes could use dangerous and harmful compounds. Additionally, the byproducts of chemical processes cannot be safe for the environment [Citation17]. Due to its ease, speed, safety, non-toxicity, affordability, and environmental friendliness, green production of nanoparticles has recently emerged as an alternative to conventional methods, taking into account the drawbacks of the physical and chemical ones [Citation18–20]. Plant extracts enhance redox reactions, which reduce metallic ions into nanoparticles [Citation21]. For metallic particles to be reduced to nanoparticles, metabolites such as sugar, terpenoids, flavonoids, alkaloids, steroids, and proteins are crucial [Citation22]. These metabolites also help to maintain the stability of nanoparticles.
Lung cancer is the primary source of years of cancer-related life loss and has the biggest economic burden compared to other tumour types [Citation23]. Modern cancer therapies include serious side effects such anaemia, hair loss, and nausea and can damage or kill healthy cells that proliferate fast. Compared to naturally occurring compounds acquired from medicinal plants, chemotherapy drugs are more costly. Therefore, employing natural remedies may be an alternate plan of action for preventing and treating cancer [Citation24]. Natural sources including plants, microorganisms, and marine animals are potential bioresources for anticancer compounds [Citation25]. The improvement of lung cancer outcomes will always depend heavily on research. Numerous phytochemicals originating from plants have been reported to possess antioxidant activities [Citation26]. Metallic nanoparticles have been used in cancer therapy for a very long time, and this poses a risk free for the public health of both developed and developing nations [Citation27,Citation28]. Anticancer characteristics, including antioxidant capabilities, have been studied for a variety of natural and synthesized substances [Citation29]. Copper nanoparticles (Cu NPs), one of the many types of metal nanoparticles, have found use in everything from food and medicine to consumer goods and washing machines [Citation30]. Significantly, the utilization of CuNPs have increased up in cancer diagnosis and therapy, not only as desirable tools for delivering targeted medications or as cancer detection probes, but also as a potential therapeutic molecule on its own.
Additionally, CuNPs have gained attention recently as a possible anticancer drug and have demonstrated potential cytotoxicity against cell lines including MCF-7, Hep2, HCT-116 colon, HepG2, HT29, Caco2, HELA and A549 [Citation31,Citation32]. The main effects of Cu NPs in cancer treatment also imply that CuNPs cause cell death through the formation of reactive oxygen species (ROS), cellular apoptosis, and DNA fragmentation [Citation33]. In general, the discharge of copper ions seems to be what causes CuNPs to be poisonous. Because Cu NPs dissolve more slowly than other materials, copper ions are continuously released [Citation34]. Cu NPs were found to have considerable cytotoxicity against the (SW480) Human Colon Cancer Cell Line, but not against healthy, normal peripheral lymphocytes (PLs) [Citation35]. However, the harmful outcomes and underlying molecular processes within human cells remain poorly investigated. The cellular events were deciphered in healthy (IMR-90) human lung fibroblast cells and (U251) human glioblastoma cells, and they indicated mitochondrial dysfunction and the induction of reactive oxygen species (ROS), which consequently triggered DNA damage and chromosomal aberrations, as the potential mechanisms [Citation36]. To track the molecular process behind the bioaccumulation and toxicity of copper ions or CuNPs, additional research on nano-toxicology is needed [Citation37,Citation38]. Even though the green synthesis of AgNPs has been testified using a certain plant species, like Anisomeles malabarica [Citation39], Jatropha curcas [Citation40], Mentha pulegium [Citation41], etc., the area has not yet been thoroughly investigated. This has motivated us to research different types of plants for the environmentally friendly green production of Cu NPs. Here, in a unique effort, copper nanoparticles were synthesized using an extract from Pedalium murex L. fruit [Citation42].
Pedalium murex L., commonly known as Large Caltrops, a flowering plant and goes to the family, Pedaliaceae and capable of serving as an herbal medication [Citation43]. As a species of Indian subcontinent, Sri Lanka, and Tropical Africa. It is a cooling tonic that cleanses the blood, acts as a diuretic, and eliminates bladder stones. Phytochemicals found in the fruit extract include carbohydrates, flavonoids, phenols, glycosides, alkaloids, steroids, saponins, fixed oils, tannins and fats. These bioactive components exhibited a variety of pharmacological activities, including antioxidant, anti-diabetic, antibacterial, aphrodisiac, anti-inflammatory, and nephroprotective actions [Citation44–46]. The Pedalium murex L. species can be investigated for the biological production of nanomaterials due to its prevalence and abundance [Citation47,Citation48].
In this study, an aqueous extract of the fruit of the Pedalium murex L. was used to investigate a green production of copper nanoparticles (CuNPs). UV-Visible Spectroscopy, energy-dispersive X-ray spectroscopy (EDX), Fourier transform infrared spectroscopy (FTIR), and scanning electron microscopy (SEM) were used to evaluate the biogenic-produced copper nanoparticles. The antioxidant and antibacterial properties of the biosynthesized Cu NPs were next evaluated to determine the biological property of the synthesized Cu NPs.
Materials and methods
Materials
Fresh dried Pedalium murexes L. fruits were properly identified and purchased from the medicinal market in the Coimbatore district, Tamil Nadu, India. All reagents (CuSO4, NaOH, H2SO4, FeCl3, phosphate buffer and ethidium bromide), ethyl alcohol, ninhydrin solution, Benedict’s reagent, trichloroacetic acid (TCA), potassium ferric cyanide, Dulbecco’s Modified Eagle’s Medium (DMEM), potato dextrose agar (PDA), Dimethyl sulfoxide (DMSO), and 3-(4,5-dimethylthiazol-2-yl)-2,5-diphenyl tetrazolium bromide (MTT) was procured from the India. The pathogenic organisms used in this study, namely Staphylococcus aureus, Streptococcus Pyogenes, E. coli, and Klebsiella pneumoniae were kindly obtained from Department of Microbiology, Coimbatore medical college and hospital (CMHC), Coimbatore. The National Center for Cell Science (NCCS), Pune, India, provided the human lung cancer cell lines (A549).
Preparation of plant extract
To get rid of dust and other associated particles, Pedalium murexes L. fruits were carefully cleaned with tap water before being rinsed with distilled water. After being washed, the fruits were dried for 15 days at room temperature in the shade. The dried fruits were then broken down into tiny bits and processed in a motorized mortar to produce powder. 10 g of the material was weighed out to make the extract, which was then combined with 100 mL of DD water and incubated for 48 hours on an orbital shaker. In order to get the pure fruit extract, the fruit solutions were filtered through Whatman No. 1 filter paper. Additionally, the filtrate was centrifuged at approximately 4000 rpm for 10 minutes to remove the debris, and the supernatant was kept at 4°C for additional research [Citation49].
Phytochemical screening
Pedalium murexes L.‘s aqueous fruit extract underwent a qualitative phytochemical screening in order to determine the phytoconstituents using the common colour test. flavonoids, Proteins, alkaloids, carbohydrates, steroids, saponins and tannins, among other substances, are present in Pedalium murexes L. fruit’s aqueous extract and actively contribute to the green production of copper nanoparticles [Citation50].
Green synthesis of copper nanoparticles
In a nutshell, copper sulphate in a 1 mM water solution was used to create copper nanoparticles. For the purpose of lowering copper particles, 20 ml of Pedalium murex L. extract of fruit was combined with 1 g of finely ground copper sulphate (CuSO4) in 80 ml of a water-based solution of 1 mM CuSO4. On a magnetic plate, the mixture was swirled while being kept at 27°C for 24 hours. Copper nanoparticles (Cu NPs) are produced when a yellow-brown colour changes to green. Solid substance with a green hue appeared and stayed suspended in the mixture. After allowing it to settle, it was separated by centrifugation for 10 minutes at 4500 rpm. A paste-like product was produced from the synthesis. After rinsing using DD water and alcohol, it was left to dry in an oven with hot air at 80°C overnight. After being scraped, the dry result was put in a silica crucible. As a result, a powder with a green hue was created and used for characterization and additional research [Citation51].
Characterization of copper nanoparticles
Various techniques were used to characterize the green produced Cu NPs. They were diluted before being first identified using UV-Visible (UV-Vis) spectroscopy. On a (UV-1700 Pharma) spectrophotometer with a resolution of 1 nm, the UV-Vis spectra of the substances have been determined between 200 and 800 nm. The probable functional groups found in the biomolecules that were in charge of the decrease and stability of the produced Cu NPs was determined using FTIR measurements. The functional group that is responsible for the reduction and capping of Cu NPs was examined using an FTIR (RX1-Perkin Elmer) spectrometer with a wavelength spectrum of 4000–600 cm−1. In order to prepare the specimen for SEM investigation, a biologically produced Cu NPs solution was drop-coated onto a copper grid that had been coated with carbon. The extra solution has been removed using blotting paper, and the layer of film on the SEM grid has been allowed to dry for five minutes before being examined for the form and dimension of the copper nanoparticles. EDAX was used to quantitatively analyse the Cu NPs’ elemental composition at a 1.3 KeV accelerating voltage [Citation52]
In vitro antioxidant activity
Hydroxyl radical scavenging activity
The hydroxyl radical scavenging capacity and total reducing power test were used to assess the antioxidant properties of the greenly produced Cu NPs. The ascorbic acid-iron EDTA technique was used to gauge the sample extract’s ability to scavenge hydroxyl radicals. The addition of 1.0 ml of iron-EDTA in the solution, 0.5 ml of EDTA solution, and 1.0 ml of DMSO in 0.1 M phosphate buffer at pH 7.4) was done in succession with different amounts (20–100 g) of extract. 0.5 ml of ascorbic acid (0.22%) was added to start the reaction, which was then incubated in a water bath at 80–90°C for 15 minutes. When the reaction had been incubated, 1.0 ml of ice-cold TCA (17.5% w/v) was added to stop it. Nash reagent was added, and 3 ml was added after 15 minutes at room temperature. As a control, the reaction solution without a specimen was employed. At 412 nm, the absorption of the colour that resulted was determined spectrophotometrically in comparison to a blank for the reagent [Citation52].
Total reducing power assay
A ferric-ferrous complex with a maximum absorbance of 700 nm is created when substances with reduction potential interact with potassium ferricyanide (Fe3+) to form potassium ferrocyanide (Fe2+), as well as with ferric. The following ingredients were combined: 1.0 ml of extract solution (20–100 l) at various concentrations; 2.5 ml of 0.2 mol/L phosphate buffer (with a pH 6.5); and 2.5 ml of 1% potassium ferric cyanide solution. For 20 minutes, the entire mixture was left to incubate at 50°C. 2.5 ml of 10% trichloroacetic acid was added to the mixture after the incubation period and centrifuged for 10 minutes at 3000 rpm. 2.5 ml of distilled water and 0.5 ml of a 0.1% ferric chloride solution were combined with the upper layer of the solution after it had been collected. At 700 nm, the absorbance was calculated in comparison to a blank.
Antibacterial activity
By using the well diffusion method, it was determined that the green produced copper nanoparticles have antibacterial activity against both gram positive (Staphylococcus aureus, Streptococcus pyogenes) and gram-negative (Escherichia coli, Klebsiella pneumoniae) bacteria. On sterile nutrient agar plates, 10 L of the suspension with 10 CFU per L of the culture of bacteria were evenly distributed [Citation53]. With the use of a sterilized gel borer, disc wells (5 mm in diameter) were created. 20 L of Cu NPs were added to the nutritional agar plates in separate wells at three levels (10, 20, and 30 mg mL-1). Fruit extract from the Pedalium murex L. plant was used to inoculate control discs. The widths of the inhibitory zones on the plates were determined in mm after 24 hours of incubation at 37°C [Citation54].
Evaluation of in vitro anticancer activity
Cell culture, cytotoxic and MTT assays
The National Center for Cell Sciences (NCCS), located in Pune, India, provided the Human Lung Cancer cells (A549). The selected cancer cells have maintained in Dulbecco’s modified eagles’ medium (DMEM) (GIBCO, U.S.A.). Streptomycin and penicillin (100 IU/100 g) have been adjusted to 1 mL/L. The cells have been kept at 37°C in a humidified CO2 environment with 5% CO2. An MTT [3-(4,5-dimethylthiazol-2-yl)-2,5-diphenyltetrazolium bromide] test has been used to calculate the inhibitory concentration (IC50) value. In a 96-well plate, the cells were cultivated for 48 hours to 80% confluence (1 × 104 cells/well). The cells were then cultured for a further 48 hours with fresh media that included a sample that had been serially diluted. The culture medium has been withdrawn, and each well has received 100 mL of the MTT solution (3-(4,5-dimethylthiazol-2-yl)-3,5-diphenyl tetrazolium bromide; Hi-Media), which has been incubated at 37°C for 4 hours. The formazan crystals were solubilized by adding 50 L of DMSO to each well after the supernatant had been removed. This process took 10 minutes. In the case of an ELISA multi-well plate analyser (Thermo Multiskan EX, U.S.A.), the optical density was measured at 620 nm. The following formula should be used to get the OD value’s viability %.
Morphological assessment of apoptotic cells
Apoptotic cells were identified using classic morphological criteria such as cell shrinking, nuclear condensation, and the generation of apoptotic bodies. A549 cells were loaded onto cover slips using the cytospin technique, treated with ethanol/acetic acid buffer (v/v, 3:1), and incubated at room temperature with varied concentrations (1 × 105 cells/cover slip). For morphometric examination, the cover slips were gently placed on glass slides. Three monolayers were micrographed for each experimental group. Nikon (Japan) bright field inversion microscopy with light at 10 magnifications was used to examine the morphological alterations of the cells.
Fluorescence microscopic analysis of apoptotic cell death
On clean microscope cover slips, 1 L of a dye solution (100 mg/mL acridine orange (AO) and 100 mg/mL ethidium bromide (EtBr) in distilled water) was combined with 0.9 mL of cell suspension (1105 cells/mL). Cancerous cells were collected, rinsed in phosphate-buffered saline (pH 7.2), and stained with 10 µL of AO/EtBr. After 2 minutes, the cells were washed twice with PBS (5 minutes each) and examined using a fluorescent microscope (Nikon Eclipse, Inc, Japan) at 400 magnifications with a 580 nm excitation filter. Similarly, the cells have been set on a 6-well plate on a glass cover slip and treated with complexes for 24 hours. The cells that were fixed were permeated with 0.2% triton X-100 (50 μl) for 10 minutes at room temperature before being incubated for 3 minutes with 10 μl of DAPI using a cover slip to ensure uniform stain distribution. The cells were examined using a fluorescent microscope (Nikon Eclipse, Inc, Japan) [Citation55].
Statistical analysis
Analytical measurements were conducted in triplicate, and the resulting experimental data are presented as mean ± standard deviation. Statistical analyses were performed using Origin software (version 7.0383; OriginLab Corporation, Northampton, MA 01060, U.S.A.). The statistical significance of the data was assessed through two-sample independent t-tests and one-way ANOVA using GraphPad InStat 3 (San Diego, CA, U.S.A.), with a significance limit set at a 5% probability level.
Results and discussion
Qualitative phytochemical screening
The shade-dried fruits of the Pedalium murex L. under study were phytochemically screened to identify its various components. The phytochemical study results are tabulated in . The aqueous fruit extract of Pedalium murex L. was discovered to be abundant in bioactive elements such carbohydrates, amino acids, saponins, phenols, tannins, alkaloids, flavonoids, glycosides, and steroids as suggested. Flavonoids are significant secondary metabolites found in P. murex that are known for their biological and pharmacological roles as well as their health benefits. The presence of these phytochemicals makes the plant a promising source for green production of nanoparticles because they are renowned for their reducing functional groups. The results coincided with Garima Mishra. Pedalium murex L. fruits contain a variety of amino acids, including threonine, aspartic acid, glutamic acid, and histidine. Although P. murex includes numerous phyto-constituents, their pharmacological actions have not been well investigated. As a result, more research is needed to connect its pharmacological effect with phytochemical proofs by increasing the combination of research being undertaken by various groups all through the world, in order that it can be developed as a potential drug candidate.
Table 1. Qualitative phytochemical screening from Pedalium murex L. aqueous extract.
Synthesis of copper nanoparticles
When the extracts of Pedalium murex L. fruit was blended with 1 mM of copper sulphate solution, copper nanoparticles were produced instantaneously and can be observed with opacity and colour change. The copper nanoparticles were effectively synthesized utilizing Pedalium murex L. fruit extract. The nanoparticles were subsequently collected, purified, and lyophilized later being centrifuged three times. The visual evidences confirm the synthesis of copper nanoparticles and are showed in . The fruit extract was initially brown, but following the addition of copper sulphate, it turned green. It suggests that the reaction mixture is producing copper sulphate. A 24-hour incubation period led to the appearance of colour changes [Citation51].
UV-Vis characterization
UV-Vis spectroscopy was used to confirm the production of green Pedalium murex L. Cu NPs. To investigate the optical properties of biosynthesized copper nanoparticles, samples were subjected to UV-Vis spectroscopic examinations (Shimadzu UV-1800) at room temperature with a resolution of 1 nm between 200 and 600 nm. Unbound electrons in metal nanoparticles form a surface plasmon resonance (SPR) absorption zone as an outcome of the mutual oscillations of metal nanoparticle electrons into resonance with light waves. The rise of the peaks demonstrates the surface plasmon resonance properties of copper nanoparticles. The copper SPR seems to occur at 350 nm and are depicted in . This band corresponds to colloidal copper nanoparticle absorption in the area (300–350) nm) caused by surface plasmon vibration excitation. As a result, the high-concentration sample was used for further investigation. The amount of biocompounds required to decrease Cu+ to Cu0 rises with the content of Pedalium murex L. fruit extract. The absorption peak at 350 nm with an increase in the time specified the synthesis of copper nanoparticles [Citation56].
Fourier transform infrared spectroscopy
Fourier transform infrared (FTIR) analysis was used to depict Pedalium murex L. extract and to identify the molecules and functional groups present in the synthesized copper nanoparticles. The FTIR spectrum of fruit extract of Pedalium murex L. Cu nanoparticles are showed in . The aqueous extract nanoparticle shows an absorption peak at 3407 cm−1 indicates the presence of NH & OH bond and 2361 cm−1 indicates the presence of CuNPs with β-CD and 1623 cm−1 C=C bond and 1440 cm−1 indicates the presence of C-N and 823 cm−1 designates the presence of CO bond and 611 cm−1 indicates the presence of CH bond. The results are tabulated in the . They confirm the existence and interaction of proteins with copper nanoparticles, which might lead to protein stabilization. These peaks represent stretching vibrational bands responsible for substances such as flavonoids and terpenoids, and they may be responsible for the effective capping and stabilization of produced copper nanoparticles [Citation57]. According to FTIR research, Pedalium murex extract’s carboxyl (-C=O), amine (N-H), hydroxyl (−OH) units are principally responsible for the reduction of Cu+ to Cu nanoparticles. These results validated the contribution of phytochemicals to the stability of the right away Cu NPs and the decrease of copper salt.
Table 2. FTIR analyses of CuNPs synthesized from aqueous extract Pedalium murex L.
Scanning electron microscopy (SEM)
Cu NP washed off with distilled water, thin films of the sample were made on a silicon plate, the film was allowed to air out on a hot plate at 100°C for two hours, and then it was gold-coated. A scanning electron microscope was employed to look at the exterior morphology and characteristics of the surface of CuNPs. Visual representations of the size and morphology of synthesized Pedalium murex L. CuNPs revealed by SEM micrographs are shown in . The SEM images also illustrates that the particles were not homogeneous in terms of shape and size. All potential spherical and irregular CuNPs geometries, including truncated hexagonal shapes, were visible under the micrographs. The common CuNPs grain size of CuNPs was determined to be between 10 and 50 nm. Due to the large surface area, particles appear to cluster slightly, resulting in the production of medium-sized particles [Citation58].
Energy dispersive absorption spectroscopy (EDAX) analysis
EDAX were performed to characterize the elemental composition of the synthesized CuNPs. Pedalium murex synthesized copper nanoparticles using energy dispersive absorption spectroscopy (EDAX) is given in . Cu was found in the final products, confirming the existence of the required element. The current research indicated that the nano-structures for Pedalium murex L. produced a peak at 1.3 keV entirely of copper nanoparticles. EDAX analysis validated the chemical element of the produced Cu NPs made from Pedalium murex L. fruit extract, which is listed in . The concentration of O was too low for XRD analysis to identify. Thus, X-ray examination appears to imply that the copper nanoparticles produced are highly pure. Furthermore, EDAX examination of the remaining samples yielded similar results [Citation59]. The EDAX spectra provide confirmation of the carbon and oxygen components of the copper elements. The secondary metabolites in the fruit extract from Pedalium murex L. may be the cause of this aggregation.
Table 3. Elemental analysis of EDAX in Pedalium murex L. CuNPs.
In vitro antioxidant activity
depicts the results of a test for total reducing power and hydroxyl radical scavenging activity used to assess the antioxidant capacity of Pedalium murex L. fruit extract and Cu NPs. The scavenging of hydroxyl radicals is a significant antioxidant activity due to the OH radical’s high reactivity, which allows it to react with a diverse spectrum of compounds found in live cells, including sugars, amino acids, lipids, and nucleotides. The ability of CuNPs and Pedalium murex L. fruit extract to scavenge hydroxyl radicals at various concentrations (25, 50, 70, 100, and 125 μg mL−1) are depicted. As can be shown, in the range of the specified concentration, the Pedalium murex L. aqueous fruit extract and the copper nanoparticles both demonstrated notable scavenging efficiencies in a dose-dependent manner [Citation60]. As a result, eliminating OH is essential for the safety of living things. With the ability to reduce the oxidized precursors of lipid peroxidation processes, compounds with reducing power can act as both primary and secondary antioxidants. Polyphenols and flavonoids have a high reducing capacity due to their electron transport properties [Citation61]. Because of their potential to scavenge free radicals, reduces lipid peroxidation, and have a lowering impact, phenolic compounds are referred to as antioxidants. Meanwhile, the dietary antioxidant like ascorbic acid was chosen for comparison in total reducing power assay. It was determined that Pedalium murex L.‘s aqueous fruit extract had the ability to reduce Fe+3 to Fe+2 (reducing effect). The scavenging ability for the minimal concentration (25 μg mL−1) of the synthesized CuNPs was 17%; this scavenging ability was increased to 63.8%, when the concentration was increased to 125 μg mL−1 with average IC50 of 102 μg mL−1. Due to the presence of the antioxidant polyphenolic chemicals on their surfaces, the scavenging activity values of Cu NPs were considered to be higher than those of ascorbic acid for all concentrations examined.
Antibacterial activity
Two strains of Gram-negative and Gram-positive bacteria were used as test subjects for the antibacterial activity of the green CuNPs. The architecture of the bacterial cell and nuclear membrane changed as a result of the high reactivity and efficiency of protein binding. Copper ions have the ability to inhibit bacterial reproduction by attaching to and denaturing bacterial DNA, which includes the interaction of copper ions with the thiol group of proteins, followed by DNA condensation and death. CuNPs (10, 20 and 30 mg mL−1) at various concentrations were prepared and utilized to the infected plates. The experiment was conducted in triplicates (n = 3) and the diameter of the inhibition zones are tabulated in . High concentrations (30 mg mL−1) of CuNPs significantly inhibited the growth of all tested bacteria, like the bacteria Escherichia coli (inhibition zone diameter, 14 mm), Klebsiella pneumoniae (inhibition zone diameter, 16 mm), Staphylococcus aureus (inhibition zone diameter, 18 mm), along with Streptococcus pyogenes (inhibition zone diameter, 14 mm).This might be explained by the fact that as the dosage of Cu NPs increases, membrane permeability rises, causing damage to the cell wall [Citation62]. Contrarily, the lowest tested Cu NPs dosage (10 mg mL−1) only mildly suppressed bacterial growth; this was likely the result of insufficient interaction between the CuNPs and the bacterial cell membrane. All of the examined microorganisms were unaffected by the Pedalium murex L. leaf extract. The inhibitory action of CuNPs was therefore inferred to be directly proportional to their concentration as shown in . The release of diffusible inhibitory chemicals from copper nanoparticles causes these bacterial group incubations around the wall. Biosynthesized nanoparticles are frequently employed in cancer therapy, wound healing, antibacterial activities, water paints, cotton fabrics and textiles, and other applications. The green synthesis of CuNPs has also cleared the way for a more effective scientific approach in the medical area. Therefore, it can be said that both Gram-positive and Gram-negative pathogens are susceptible to the green synthesized nanoparticles [Citation63].
Figure 7. Well diffusion assay of Cu nanoparticles synthesized from fruit extract of Pedalium murex L. against (a) Staphylococcus aureus, (b) Streptococcus pyogenes, (c) Klebsiella pneumoniae and (d) E. coli.

Table 4. Zone of inhibition (ZOI) of Cu nanoparticles on Staphylococcus aureus, Streptococcus pyogenes, Klebsiella pneumoniae, and E. coli.
It has been hypothesized that copper nanoparticles obtained from Pedalium murex bind to the outer membrane of bacteria and increase its permeability, resulting in cell death [Citation64,Citation65]. It is commonly accepted that the interaction of the copper ions with negatively charged species present in the cytoplasm layer is the mechanism by which copper exerts its antibacterial effect [Citation66]. Copper ions are released in the aqueous solution as the Cu nanostructure in contact with the bacteria and preferentially attaches to the cell wall. The oxygen and hydroxyl groups in aqueous media stabilize copper nanoparticles by having a powerful interaction with the surface metal atoms, in addition to securely anchoring copper ions onto the cell wall through ion-dipole interactions [Citation67,Citation68]. According to this interaction, the cell membrane may contract or separate from the wall of the cell. As a result of the cytoplasm membrane contracting or separating from the cell wall, the DNA molecules get condensed and lose their capacity to multiply when copper ions are introduced. Additionally, it has been proposed that the copper ions may interact with proteins’ thiol groups, leading to the inactivation of bacterial proteins. Furthermore, the membrane’s contraction makes it less likely that nutrients will enter the cell for continued growth and proliferation [Citation69]. Further studies on the mechanism of copper nanoparticles need to be analysed using microscopic studies to confirm the adhering property of copper nanoparticles.
In vitro cytotoxic activity of pedalium murex L. CuNPs
Effect of MTT assay
The IC50 values of aqueous extract of Pedalium murex L. copper nanoparticles & Standard was investigated and explored. The aqueous fruit extract of Pedalium murex L. copper nanoparticles against A549 Lung cancer cell line in control, 10 µg/ml, 25 µg/ml, 50 µg/ml are depicted . No pharmacological remedy was utilized as a negative control, whereas Doxorubicin has used as a positive control. All treatments have been performed in triplicate, and average absorbance and cell viability has determined and are tabulated in . The % cell viability of copper synthesized Pedalium murex L. aqueous extract decreased with increasing concentrations. These percentages of cell viability dropped as concentrations increased. The greatest concentration had the lowest percentage of viable cells, and vice versa. The methanolic fraction displayed potential anticancer activity and had significantly reduced cytotoxicity for normal cells such as MRC-5 (IC50 37 g/mL) than in cancer cells. On treatment with various solvent fractions such as acetone, petroleum ether and water, cytotoxicity was substantially lower/no cytotoxicity was detected. A dose response curve for the effect of different fractions on A549 cells was produced on . y = 13.53ln(x) + 92.768, where x is the concentration in g/mL and y is the cell viability percentage, is the regression equation for the effect of the methanolic fraction on the A549 cells [Citation70].
Figure 8. Pedalium murex L. copper nanoparticles treated against A549 lung cancer cell line in (a) control, (b) 10 µg/ml, (c) 25 µg/ml and (d) 50 µg/ml.

Figure 9. Effect of aqueous extract of Pedalium murex L. copper nanoparticles against A549 cancer cell.

Table 5. IC50 values of aqueous extract of Pedalium murex L. copper nanoparticles & standard.
Effect of AO/EB staining in cancer cells.
AO is cell permeable and penetrates both living and dead cells. Live cells exhibit consistently bright green fluorescence, whereas dead cells exhibit green or orange fluorescence, indicating cell apoptosis or necrosis. EB diffuses into cells with damaged membranes and causes orange or bright red fluorescence. The AO/EB staining method has been used to distinguish between normal, apoptotic, and necrotic cells. The Dual AO/EB fluorescence staining technique detects apoptosis-related morphological alterations in cell membranes following chemo preventive drug treatment. The morphological alterations of A549 cells following treatment with extract have been investigated using the AO/EB staining technique, which revealed live cells and are shown in . After treatment, the cells undergo morphological changes such as shrinkage, chromatin condensation, and inter nucleosomal fragmentation. Fluorescence microscopy demonstrated that the control A549 cancer cells had a low amount of acidic vesicular organelles (AVOs) in the cytoplasm and fluorescence that was primarily green with little red/orange. In contrast, the majority of the treated cells had a higher number of AVOs in the perinuclear area of the cytoplasm [Citation71].
Effect of DAPI staining (4’,6-diamidino-2-phenylindole)
Based on the outcomes of the MTT assay, DAPI staining has been used to determine if Pedalium murex L. copper nanoparticles extract promoted apoptosis. The mechanism of death of A549 cells after treatment with Pedalium murex L. aqueous fraction copper nanoparticles was investigated. Apoptosis and necrosis can be distinguished by their distinct nuclear alterations. DAPI, a nuclear stain that emits blue fluorescence when it gets activated that can be viewed under a fluorescence microscope. The DAPI staining of aqueous extract of Pedalium murex L. CuNPs against A549 cancer cell in control and cells treated with CuNPs at doses of 10, 25, and 50 g/ml, are shown in . They exhibited a nuclear morphological alteration that may be an indication of apoptosis. Morphological alterations detected in the treated cells included cell shrinkage, nucleus fragmentation, and cell budding, which results in cells of several sizes. Increased concentrations of copper nanoparticles from Pedalium murex L. seemed to produce more morphological alterations, showing that apoptosis happened in a concentration-dependent manner [Citation72].
Conclusion
This study reported a secure and effective method for producing Cu NPs using an aqueous extract of the fruit of Pedalium murex L. For a thorough investigation of the green-produced nanoparticles, several methods including UV-Vis, SEM, FTIR, and EDAX spectroscopy were applied. SEM images revealed the size range of the nanoparticles, which may have spherical or irregular geometries and ranged in size from 10 to 50 nm. Depending on the FTIR results, it was postulated that phytoconstituents found in Pedalium murex L. were in charge of reducing copper ions and capping the created Cu NPs. The crystalline character of the Cu NPs was further supported by the EDAX analysis patterns, and it was discovered that copper at 1.3 KeV dominated their elemental makeup. These nanoparticles’ antibacterial and anti-oxidant activities showed a maximum efficiency of 63.8% at the greatest concentration (125 g/L). Against both gram-positive and gram-negative bacteria, they demonstrated significant antibacterial action. The results of the current investigation showed that greenly produced Cu NPs significantly inhibited the proliferation of human lung cancer A549 cells. In investigations on antibacterial, antioxidant, and in vitro cytotoxic action on A549 cell lines, Cu NPs may thus be considered a promising substance. The fact that more morphological changes appeared in response to higher copper nanoparticle concentrations from Pedalium murex L. suggests that apoptosis happened in a concentration-dependent way. This study shows that the manufacture of Cu NPs utilizing an aqueous fruit extract of Pedalium murex L. has a very high potential for usage as life-saving anticancer medications in addition to having a much-reduced negative impact on the environment. Cu NPs may be used in the therapy of non-small cell lung cancer (NSCLC), but more research is required.
Acknowledgments
Authors are thankful to the Researchers Supporting Project number (RSPD2023R728), King Saud University, Riyadh, Saudi Arabia.
Disclosure statement
No potential conflict of interest was reported by the author(s).
Data availability statement
The authors confirm that the data supporting the findings of this study are available within the article.
References
- Thiruvengadam S, Kumar BSM, Yamini C. Applications of Nanotechnology in the world of biology -A Scientific Review. Int Jou Sci Rese Rev. 2019;8:106–16.
- Majeed S, Aripin FHB, Shoeb NSB, et al. Bioengineered silver nanoparticles capped with bovine serum albumin and its anticancer and apoptotic activity against breast, bone and intestinal colon cancer cell lines. Mater Sci Eng C. 2019;102:254–263. doi: 10.1016/j.msec.2019.04.041
- Khan F, Shariq M, Asif M, et al. Green nanotechnology: Plant-Mediated nanoparticle synthesis and application. [Internet]. Nanomaterials. [cited 2023 Aug 7]. 2022;12:673. 4. doi: 10.3390/nano12040673
- Chota A, George BP, Abrahamse H. Recent advances in green metallic nanoparticles for enhanced drug delivery in photodynamic therapy: a therapeutic approach. Int J Mol Sci. 2023;24(5):4808. doi: 10.3390/ijms24054808
- Mittal AK, Tripathy D, Choudhary A, et al. Bio-synthesis of silver nanoparticles using Potentilla fulgens wall. ex hook. And its therapeutic evaluation as anticancer and antimicrobial agent. Mater Sci Eng C Mater Biol Appl. 2015;53:120–127. doi: 10.1016/j.msec.2015.04.038
- Agnihotri SK, Prashant DV, Samajdar DP. Role of metallic nanoparticles in the optoelectronic performance enhancement of InP ultrathin film solar cell. Opt Mater. 2022;134:113129. doi: 10.1016/j.optmat.2022.113129
- Chung I-M, Park I, Seung-Hyun K, et al. Plant-mediated synthesis of silver nanoparticles: their characteristic properties and therapeutic applications. Nanoscale Res Lett. 2016;11(1):40. doi: 10.1186/s11671-016-1257-4
- Shetti NP, Bukkitgar SD, Reddy KR, et al. ZnO-based nanostructured electrodes for electrochemical sensors and biosensors in biomedical applications. Biosens Bioelectron. 2019;141:111417. doi: 10.1016/j.bios.2019.111417
- Sápi A, Rajkumar T, Kiss J, et al. Metallic nanoparticles in heterogeneous catalysis. Catal Lett. 2021;151(8):2153–2175. doi: 10.1007/s10562-020-03477-5
- Mohammed SSS, Lawrance AV, Sampath S, et al. Facile green synthesis of silver nanoparticles from sprouted Zingiberaceae species: spectral characterisation and its potential biological applications. Mater Technol. 2022;37(8):533–546. doi: 10.1080/10667857.2020.1863571
- Mafuné F, Jun-ya K, Kondow TT, et al. Structure and stability of silver nanoparticles in aqueous solution produced by laser ablation. J Phys Chem B. 2023;104:8333–8337. [Internet]. [cited 2023 Aug 7] [Internet]: https://pubs.acs.org/doi/10.1021/jp001803b
- Tabrizi NS, Xu Q, van der Pers NM, et al. Synthesis of mixed metallic nanoparticles by spark discharge. J Nanopart Res. 2009;11(5):1209–1218. doi: 10.1007/s11051-008-9568-8
- Pingali KC, A Rockstraw D, Deng S. Silver nanoparticles from Ultrasonic Spray Pyrolysis of aqueous silver Nitrate. Aero Sci and Techn. 2023;10:101–1014. [Internet]. [cited 2023 Aug 7]. [Internet]: https://www.tandfonline.com/doi/full/10.1080/02786820500380255
- Thuc DT, Huy TQ, Hoang LH, et al. Green synthesis of colloidal silver nanoparticles through electrochemical method and their antibacterial activity. Mater Lett. 2016;181:173–177. doi: 10.1016/j.matlet.2016.06.008
- Quintero-Quiroz C, Acevedo N, Zapata-Giraldo J, et al. Optimization of silver nanoparticle synthesis by chemical reduction and evaluation of its antimicrobial and toxic activity. Biomater Res. 2019;23(1):27. doi: 10.1186/s40824-019-0173-y
- Chen P, Song L, Liu Y, et al. Synthesis of silver nanoparticles by γ-ray irradiation in acetic water solution containing chitosan. Radiat Phys Chem. 2007;76(7):1165–1168. doi: 10.1016/j.radphyschem.2006.11.012
- Pryshchepa O, Pomastowski P, Buszewski B. Silver nanoparticles: synthesis, investigation techniques, and properties. Adv Colloid Interface Sci. 2020;284:102246. doi: 10.1016/j.cis.2020.102246
- Kannan BN, Thoppil J. Plant-mediated synthesis of silver nanoparticles by two species of cynanchum L. (Apocynaceae): a comparative approach on its physical characteristics. Int J Nano Dimens. 2018;9:104–111.
- Bar H, DKr B, Sahoo GP, et al. Green synthesis of silver nanoparticles using latex of jatropha curcas. Colloids Surf A Physicochem Eng Asp. 2009;339(1–3):134–139. doi: 10.1016/j.colsurfa.2009.02.008
- Ahmed S, Saifullah, Ahmad M, et al. Green synthesis of silver nanoparticles using Azadirachta indica aqueous leaf extract. J Radiat Res Appl Sci. 2016;9(1):1–7. doi: 10.1016/j.jrras.2015.06.006
- Kuppusamy P, Yusoff MM, Maniam GP, et al. Biosynthesis of metallic nanoparticles using plant derivatives and their new avenues in pharmacological applications – an updated report. Saudi Pharm J. 2016;24(4):473–484. doi: 10.1016/j.jsps.2014.11.013
- Green biosynthesis of nanoparticles: mechanisms and applications [Internet]. CABI Books. [cited 2023 Aug 7]. Available from: https://www.cabidigitallibrary.org/doi/abs/10.1079/9781780642239.0000
- Ridge CA, McErlean AM, Ginsberg MS. Epidemiology of lung cancer. Semin Intervent Radiol. 2013;30(2):093–098. doi: 10.1055/s-0033-1342949
- Greenwell M, Rahman PKSM. Medicinal plants: their use in anticancer treatment. Int J Pharm Sci Res. 2015;6:4103–4112.
- Wang J, Jiang Y-F. Natural compounds as anticancer agents: experimental evidence. World J Exp Med. 2012;2(3):45–57. doi: 10.5493/wjem.v2.i3.45
- Yu M, Gouvinhas I, Rocha J, et al. Phytochemical and antioxidant analysis of medicinal and food plants towards bioactive food and pharmaceutical resources. Sci Rep. 2021;11(1):10041. doi: 10.1038/s41598-021-89437-4
- Tinajero-Díaz E, Salado-Leza D, Gonzalez C, et al. Green metallic nanoparticles for cancer therapy: evaluation models and cancer applications. Pharmaceutics. 2021;13(10):1719. doi: 10.3390/pharmaceutics13101719
- Gul R, Saddique M, Khan MA, et al. Eco-friendly synthesis of silver nanoparticles and its biological evaluation using Tamarix aphylla leaves extract. Mater Technol. 2022;37(9):962–969. doi: 10.1080/10667857.2021.1908770
- Dehelean CA, Marcovici I, Soica C. Molecules | free full-text | plant-derived anticancer compounds as New perspectives in drug discovery and alternative therapy. [Internet]. Molecules. [cited 2023 Aug 7];26(4):1109. Available from: https://www.mdpi.com/1420-3049/26/4/1109
- Rojas B, Soto N, Villalba M, et al. Antibacterial Activity of Copper Nanoparticles (CuNPs) against a Resistant Calcium Hydroxide Multispecies Endodontic Biofilm. Nanomaterials (Basel). 2021;11(9):2254. doi: 10.3390/nano11092254
- Gnanavel V, Palanichamy V, Roopan SM. Biosynthesis and characterization of copper oxide nanoparticles and its anticancer activity on human colon cancer cell lines (HCT-116). J Photochem Photobiol B. 2017;171:133–138. doi: 10.1016/j.jphotobiol.2017.05.001
- Biogenic copper oxide nanoparticles from bacillus coagulans induced reactive oxygen species generation and apoptotic and anti-metastatic activities in breast cancer cells - PMC. [Internet]. [cited 2023 Aug 7]. Available from: https://www.ncbi.nlm.nih.gov/pmc/articles/PMC9958044/
- Chakraborty N, Banerjee J, Chakraborty P, et al. Green synthesis of copper/copper oxide nanoparticles and their applications: a review. Green Chem Lett Rev. 2022;15(1):187–215. doi: 10.1080/17518253.2022.2025916
- Yang L, Wang W-X. Comparative contributions of copper nanoparticles and ions to copper bioaccumulation and toxicity in barnacle larvae. Environ Pollut. 2019;249:116–124. doi: 10.1016/j.envpol.2019.02.103
- Ghasemi P, Shafiee G, Ziamajidi N, et al. Copper nanoparticles induce apoptosis and oxidative stress in SW-480 human colon cancer cell line [Internet]. Review. 2022. [cited 2023 Aug 7]. Available from: https://www.researchsquare.com/article/rs-1885906/v1
- AshaRani PV, Low Kah Mun G, Hande MP, et al. Cytotoxicity and genotoxicity of silver nanoparticles in human cells. ACS Nano. 2009;3(2):279–290. doi: 10.1021/nn800596w
- Hua J, Vijver M, Ahmad F, et al. Toxicity of different sized copper nano- and sub-micron particles and their shed copper ions to zebrafish embryos. Environ Toxicol Chem. 2014;33(8):1774–1782. doi: 10.1002/etc.2615
- Malhotra N, Ger T-R, Uapipatanakul B, et al. Review of copper and copper nanoparticle toxicity in fish. Nanomaterials (Basel). 2020;10(6):1126. doi: 10.3390/nano10061126
- Govindarajan M, Rajeswary M, Veerakumar K, et al. Green synthesis and characterization of silver nanoparticles fabricated using anisomeles indica: mosquitocidal potential against malaria, dengue and Japanese encephalitis vectors. Exp Parasitol. 2016;161:40–47. doi: 10.1016/j.exppara.2015.12.011
- Chauhan N, K Tyagi A, Kumar P, et al. Antibacterial potential of jatropha curcas synthesized silver nanoparticles against food Borne Pathogens Front Microbiol. 2023;7:1748. [Internet]. [cited 2023 Aug 11]. [Internet]: https://www.ncbi.nlm.nih.gov/pmc/articles/PMC5099242/
- Motakef-Kazemi N, Yaqoubi M. Green synthesis and characterization of Bismuth oxide nanoparticle using Mentha pulegium extract. [Internet]. Iran J Pharm Res. 2023;19:70–79. [cited 2023 Aug 11]. [Internet]: https://brieflands.com/articles/ijpr-124421.html
- Paramasivam G, Karnan P, Subbiah S, et al. Green synthesis of copper nanoparticles obtained from Pedalium murex. L (Yanai Nerunjil) and their antimicrobial activity. Inter Jo of Rese Advet Techn. 2019;7:301–310.
- (PDF) phytochemical and pharmacological studies in Pedalium murex L [Internet]. [cited 2023 Aug 11]. Available from: https://www.researchgate.net/publication/314654428_Phytochemical_and_pharmacological_studies_in_Pedalium_murex_L
- Devanesan AA, Zipora T, Smilin BA G, et al. Phytochemical and pharmacological status of indigenous medicinal plant Pedalium murex L.—A review. Biomed Pharmacother. 2018;103:1456–1463. doi: 10.1016/j.biopha.2018.04.177
- Sermakkani M, Thangapandian V. PHYTOCHEMICAL SCREENING FOR ACTIVE COMPOUNDS IN PEDALIUM MUREX L. Rece Rese Scie and Techn. 2010;2:110–114.
- Bharskar GR. Review on phytochemistry and pharmacological aspects of Pedalium murex Linn. Asia Journ Of Resear In Pharmac Scie. 2020;10(3):183–188. doi: 10.5958/2231-5659.2020.00035.1
- Balan L, Chandrasekaran S, Gajendiran M, et al. Synthesis of silver nanoparticles from Pedalium murex L. and its antiproliferative activity against breast cancer (MCF-7) cells. J Mol Struct. 2021;1242:130695. doi: 10.1016/j.molstruc.2021.130695
- (PDF) antioxidant activities of the aqueous extracts of Pedalium murex D. Royen EX L. Fruit and leafy stem [Internet]. [cited 2023 Aug 11]. Available from: https://www.researchgate.net/publication/354237956_Antioxidant_Activities_of_the_Aqueous_Extracts_of_Pedalium_murex_D_Royen_EX_L_Fruit_and_Leafy_Stem
- (PDF) silver nanoparticles: synthesis, mechanism of antimicrobial action, characterization, medical applications, and toxicity effects. [cited 2023 Aug 14]. InternetAvailable from: https://www.researchgate.net/publication/309584094_Silver_nanoparticles_Synthesis_mechanism_of_antimicrobial_action_characterization_medical_applications_and_toxicity_effects
- Mahajan R, Itankar P. Phytochemical analysis and standardization of Pedalium murex Linn. Extract through HPLC methods. IJNP. 2021;35(1):32–37. doi: 10.5530/ijnp.2021.1.7
- Amjad R, Mubeen B, Ali SS, et al. Green synthesis and characterization of copper nanoparticles using Fortunella margarita leaves. Polymers. 2021;13(24):4364. doi: 10.3390/polym13244364
- Bharathi D, Diviya Josebin M, Vasantharaj S, et al. Biosynthesis of silver nanoparticles using stem bark extracts of Diospyros montana and their antioxidant and antibacterial activities. J Nanostruct Chem. 2018;8(1):83–92. doi: 10.1007/s40097-018-0256-7
- Shabatina T, Vernaya O, Shumilkin A, et al. Nanoparticles of bioactive Metals/metal oxides and their nanocomposites with antibacterial drugs for biomedical applications. Materials. 2022;15(10):3602. doi: 10.3390/ma15103602
- Tahir A, Quispe C, Herrera-Bravo J, et al. Green synthesis, characterization and antibacterial, antifungal, larvicidal and anti-termite activities of copper nanoparticles derived from Grewia asiatica L. Bull National Res Centre. 2022;46(1):188. doi: 10.1186/s42269-022-00877-y
- Byczkowska A, Kunikowska A, Kaźmierczak A. Determination of ACC-induced cell-programmed death in roots of Vicia faba ssp. minor seedlings by acridine orange and ethidium bromide staining. Protoplasma. 2013;250(1):121–128. doi: 10.1007/s00709-012-0383-9
- Ultraviolet-visible spectrum of copper nanoparticles | Download Scientific Diagram [Internet]. [cited 2023 Aug 11]. Available from: https://www.researchgate.net/figure/Ultraviolet-visible-spectrum-of-copper-nanoparticles_fig1_317066284
- Rameshwari KS, Abirami M, Christobel RGJ. Partial characterization of green synthesized silver nanoparticles of Cardiospermum halicacabum and butea monosperma aqueous extract combination (CHBMCSNP). Res J Pharm Technol. 2020;13(12):6218–6223. doi: 10.5958/0974-360X.2020.01084.7
- Alshammari SO, Mahmoud SY, Farrag ES. Root extract and its applications | free full-text | synthesis of green copper nanoparticles using medicinal plant Krameria sp [Internet]. Molecules. [cited 2023 Aug 15];28(12):4629. Available from: https://www.mdpi.com/1420-3049/28/12/4629
- Suárez-Cerda J, Espinoza-Gómez H, Alonso-Núñez G, et al. A green synthesis of copper nanoparticles using native cyclodextrins as stabilizing agents. J Saudi Chem Soc. 2017;21(3):341–348. doi: 10.1016/j.jscs.2016.10.005
- Sangeetha G, Vidhya R, Xuan W. A traditional Chinese medicine compound (Jian Er) for presbycusis in a mouse model: reduction of apoptosis and protection of cochlear sensorineural cells and hearing. Int J Herb Med. 2018;6(6):127–135.
- Moini H, Arroyo A, Vaya J, et al. Bioflavonoid effects on the mitochondrial respiratory electron transport chain and cytochrome c redox state. Redox Rep. 1999;4(1–2):35–41. doi: 10.1179/135100099101534729
- Sánchez-López E, Gomes D, Esteruelas G, et al. Metal-based nanoparticles as antimicrobial agents: an overview. Nanomaterials (Basel). 2020;10(2):292. doi: 10.3390/nano10020292
- Anandalakshmi K, Venugobal J, Ramasamy V. Characterization of silver nanoparticles by green synthesis method using Pedalium murex leaf extract and their antibacterial activity. Appl Nanosci. 2016;6(3):399–408. doi: 10.1007/s13204-015-0449-z
- Gholami M, Azarbani F, Hadi F, et al. Eco-friendly synthesis of copper nanoparticles using Mentha pulegium leaf extract: characterisation, antibacterial and cytotoxic activities. Mater Technol. 2022;37(10):1523–1531. doi: 10.1080/10667857.2021.1959214
- Prakash MVD, Sampath S, Amudha K, et al. Eco-friendly green synthesis of copper nanoparticles from Tinospora cordifolia leaves: optical properties with biological evaluation of anti-microbial, anti-inflammatory and anti-oxidant applications. Mater Technol. 2023;38(1):2247908. doi: 10.1080/10667857.2023.2247908
- Tharchanaa SB, Priyanka K, Preethi K, et al. Facile synthesis of Cu and CuO nanoparticles from copper scrap using plasma arc discharge method and evaluation of antibacterial activity. Mater Technol. 2021;36(2):97–104. doi: 10.1080/10667857.2020.1734721
- S K, V A, K R, et al. One step preparation of green reduced copper oxide nanorods using Citrus sinensis L. peel extracts and evaluation of their photocatalytic degradation of Rose Bengal dye and antibacterial activity. Materials Technology. 2022;37(9):1230–1241. doi: 10.1080/10667857.2021.1929720
- Maulana I, Fasya D, Ginting B. Biosynthesis of Cu nanoparticles using polyalthia longifolia roots extracts for antibacterial, antioxidant and cytotoxicity applications. Mater Technol. 2022;37(13):2517–2521. doi: 10.1080/10667857.2022.2044217
- Aziz WJ, Abid MA, Hussein EH. Biosynthesis of CuO nanoparticles and synergistic antibacterial activity using mint leaf extract. Mater Technol. 2020;35(8):447–451. doi: 10.1080/10667857.2019.1692163
- Ramadurai M, Rajendran G, Bama TS, et al. Biocompatible thiolate protected copper nanoclusters for an efficient imaging of lung cancer cells. J Photochem Photobiol, B. 2020;205:111845. doi: 10.1016/j.jphotobiol.2020.111845
- Al-Kawmani AA, Alanazi KM, Farah MA, et al. Apoptosis-inducing potential of biosynthesized silver nanoparticles in breast cancer cells. J King Saud Univ Sci. 2020;32(4):2480–2488. doi: 10.1016/j.jksus.2020.04.002
- Pajaniradje S, Mohankumar K, Pamidimukkala R, et al. Antiproliferative and apoptotic effects of sesbania grandiflora leaves in human cancer cells. Biomed Res Int. 2014;2014:474953. doi: 10.1155/2014/474953