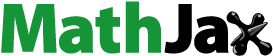
ABSTRACT
Current study aims at synthesising Eu-doped ZnS nanoparticles by sol-gel co-precipitation and then characterising them for their structural, optical, and morphological features. Eu-doped ZnS NPs with doping levels of 1, 3, 5, and 7 at.% were synthesised. The characterisation tools such as XRD, UV-Vis, FTIR, photoluminescence and FESEM were employed to analyse their properties. Zinc blend phase with particle sizes lying in the range of 5.0 to 7.2 nm was confirmed by XRD. Eu-doped ZnS NPs show a band gap decrement at a higher level of doping. Defects induced UV and visible emission peaks were observed in photoluminescence emission spectra. Random-shaped particle-like structures that effectively have a persistent enlargement of size were seen in FESEM. The particle size was seen to monitor the band gap at higher doping levels. Eu doping was found to promote the growth of particle sizes which eventually resulted in decrement of the band gap.
Introduction
Semiconductor nanostructures are a new class of materials with dimensions ranging from 1 to 100 nm, which provide tremendous potential for improving performance and extending the capabilities of products in several industrial applications [Citation1–4]. Zinc sulphide (ZnS) is one of the pioneering, commercially successful, and valuable II-VI binary semiconductor phosphors with a wide band gap (3.6 eV) and high exciton binding energy (~40 meV) at room temperature [Citation5]. Due to its wide band gap, ZnS is a suitable choice for preparing core-shell quantum structures which are much more popular nowadays for fabricating powerful light-emitting devices [Citation6,Citation7]. It has traditionally shown remarkable fundamental properties, versatility, and a promise for novel and diversified applications, including light-emitting diodes (LEDs), electroluminescence, flat panel displays, infrared windows, sensors, lasers, biodevices, etc [Citation1,Citation8–12]. Based on its large bandgap and stability in both zinc blende (ZB) and hexagonal wurtzite (WZ) structure, ZnS is therefore more suitable choice for visible-blind ultraviolet (UV)-light-based devices such as sensors or photodetectors. On the other hand, ZnS is traditionally the most suitable candidate for electro-luminescence devices [Citation1,Citation5,Citation8,Citation13,Citation14]. However, the nanostructures of ZnS have not been investigated in much detail relative to the other most popular Zinc-based nanostructures, like ZnO, though ZnS is a potentially attractive candidate to be used in modern optoelectronic industries [Citation15–17]. ZnS nanostructures exhibit relatively bright and diverse luminescence behaviour producing excellent fluorescence and electroluminescence, which makes them a good fluorescent host material. Tuning the luminescence properties of ZnS nanoparticles (NPs) is a great challenge for researchers, and they have achieved it by adding a variety of transition (TM) and rare-earth (RE) metals in relevant amounts and following appropriate synthesis methods. Since ZnS is a wide band gap semiconductor at room temperature, its nanocrystals may be considered more suitable host materials for different doping elements like RE and TM metal ions, which are optically, electronically, and magnetically active [Citation18–22]. Europium (Eu), one of the most suitable rare-earth materials attracts special attention as Eu doping significantly enhances the optical properties of ZnS nanostructures and also provides efficient luminescence centres in the host materials by means of 4f intra-shell transitions mechanism, which plays a vital role in optical applications [Citation23–25]. Recently, Eu, as a dopant, has been intensively investigated as a blue and blue-green-emitting activator in a wide variety of host materials, particularly in the form of powders or NPs, such as graphene, zeolite derivatives, and some other semiconductor oxides, etc [Citation26]. It is pertinent to mention that there is a smaller number of reported literature where the effect of Eu doping and its effect on the properties of ZnS NPs have been studied. It is highly anticipated that Eu ions can alter the colour as well as the intensity of emission from the ZnS host. The aforementioned discussion formulates a necessity to understand and analyse the observations that demonstrate how the structural and optical properties are impacted by Eu doping in ZnS NPs. Accordingly, here the Eu-doped ZnS nanophosphors have been taken for investigation. The method adopted here to synthesize the ZnS based NPs is sol-gel co-precipitation. Sol-gel methods are widely applied in the preparation of a wide variety of materials such as metal oxides, nitrides, sulphides, and carbides [Citation27–31]. A thorough understanding of the change in band gap due to RE-ion (Eu) doping in the ZnS host matrix has been explored. Changes in the morphology due to an increase in doping are also elucidated here. An attempt has been made to corroborate the observed changes in band gap energy, structural, morphological and luminescence properties of ZnS NPs due to Eu doping.
Experimental details
Materials
The materials used for the preparation of undoped and Eu-doped ZnS NPs were Zinc sulphate, Sodium sulphide, and Europium chloride. Distilled water was used as a solvent for the synthesis process. All the glass wares used in the whole procedure were acid and ethanol washed and dried properly.
Synthesis of Europium-doped ZnS nanoparticles
Sol-gel wet chemical method was employed to synthesize ZnS NPs. For preparing undoped ZnS NPs, the method has already been reported [Citation5]. Here, we synthesised the Eu-doped ZnS NPs. EuCl3 was taken as a dopant material to facilitate the Eu doping of the ZnS matrix. Dopant material was used in different concentrations. After the formation of dopant solutions in distilled water in different concentrations, they were added to zinc sulphate solutions. The mixture was stirred for half an hour on vigorous stirring. After that, sodium sulphide solution was added to the above reaction drop by drop under constant magnetic stirring at 500 rpm. The reaction was carried out for 3 h. Then the obtained white precipitate was then treated the same as ZnS nanoparticles. At the end, all of the Eu-doped samples were calcined at 300°C in a muffle furnace. The samples were allowed to cool down to room temperature and collected in glass vials for further characterisation. The corresponding amounts of EuCl3 were taken in such a way so as to obtain the Eu doping levels of 1at.%, 3at.%, 5at.%, and 7at.%, and the corresponding samples are referred to as EZ1, EZ3, EZ5, and EZ7, respectively.
Results and discussion
X-ray diffraction analysis of Europium-doped ZnS
X-ray diffraction is a widely used technique for structural analysis of synthesised NPs. It gives information about crystal lattice, phase, orientation, dislocations, defects, and several other crystallographic parameters. We used Rigaku automated multipurpose X-ray diffractometer (model: SMARTLAB) to complete the structural analysis of our four samples (EZ1, EZ3, EZ5, EZ7). shows the XRD patterns of our Eu-doped ZnS samples within a range of 20°-80°. Three intense peaks at 28.70, 47.70, and 56.50, are visible to confirm the formation of cubic or zinc blend ZnS with growth direction along (111), (220), and (311), respectively. Precise observation indicates the presence of low intense (200) (400) and (331) peaks approximately at 33.1°, 69.4° and 76.7°. All the mentioned planes are the signature of face centered cubic (fcc) ZnS structure as reported in ICDD PDF 65–1691 [Citation1]. The XRD results showed no evidence of any Europium (Eu) ions or its compound, which by itself proves that Eu is completely soluble as a dopant in the ZnS matrix. However, a careful observation indicates minor shifting in peak positions of the most important (111) plane in all three samples as shown in . Detail peak positions and FWHM are tabulated in . Calculated FWHM and peak broadening indicate the synthesised particles remain within the nano range. To confirm this, we used the Scherrer equation [Citation32] and calculated particle size (tS) for all significant diffraction peaks, i.e., (111), (220), and (311). All calculated values are listed in . The particle size varies within a range from 5.0 to 7.2 nm, which is very close to the reported Bohr diameter i.e. 4.8 nm of ZnS [Citation33]. The orientation parameters (γ) were calculated to determine the preferred orientations of the planes inside the NPs, using the equation [Citation5]:
Figure 1. X-Ray diffraction spectra of Europium (Eu)-doped ZnS nanoparticles. Curves (a), (b), (c), and (d) correspond to sample EZ1 (1 at% Eu-doped ZnS), EZ3 (3 at% Eu-doped ZnS), EZ5 (5 at% Eu-doped ZnS) and EZ7 (7 at% Eu-doped ZnS), respectively. Three major peaks suggest the NPs are formed in the cubic ZnS phase.

Figure 2. Variation in the position of diffraction XRD peaks for the samples EZ1-EZ7 with increasing concentration of Eu is shown. Curves a, and b represent major planes (111), and (220), respectively.

Table 1. Lattice constant, d-spacing, stacking faults, and orientation parameters calculated using XRD data.
Table 2. Strain and crystallite sizes obtained by the Scherrer equation (tS) along different prominent planes and by W-H plot (tWH.).
Here, represents the intensities of the corresponding planes with Miller indices (hkl). All calculated values are tabulated in , which supports a random growth distribution of the particles. We also calculated the dislocation density of the samples using the relation [Citation34]
Calculated values may be found in . The dislocation densities change significantly as particle size increases. As shown in the table, the size of the particle increases gradually for sample EZ1 to EZ7. Similar changes were observed in the dislocation density, which may be correlated with calculated particle sizes. To understand better a graphical variation of is shown in . for all samples. Further, to check the effect of Eu on the ZnS matrix, lattice parameters for all samples were calculated [Citation33]
Figure 3. Comparison of dislocation densities (δ) obtained using Scherrer equation for different peaks for samples EZ1-EZ7.

Table 3. Calculated dislocation density (δhkl.).
Here, are miller indices. It can be noticed from all computed dhkl values as listed in , that the calculated parameter shows an increment with increase in doping amount (EZ1–EZ5). It again starts to reduce for higher amount of doping (EZ7). The trend is similar for all samples and for all prominent peaks. To understand the quality of the samples, other crystallographic parameters like d-spacing, stacking fault, lattice enhancements etc. were calculated and are listed in .
Williamson-Hall plot and strain analysis
Williamson-Hall (W-H) plots were performed to recognise the strain profile of the crystal lattices. It is a known fact that the peak broadening in the XRD analysis is caused by factors including lattice strain, particle size etc. These two variables are irrelevant to one another. The following W-H equation was used to calculate the particle size and strain [Citation35].
Here, is crystallite size,
is micro-strain,
is the wavelength used and
is FWHM in radian. A graph () has been plotted between
and
. The slope and intercept of the fitted line was used to estimate micro-strain and particle size, respectively. Obtained values are listed in . The calculated strain was negative for all the samples which indicates, they are facing a compressive strain during growth [Citation36]. The calculated particle sizes (
shows slight variations, with the size obtained from Scherrer equation (
.
Energy dispersive X-ray study (EDX) for elemental analysis
To ensure the presence of the desired elements like Zn, S and Eu, EDX spectra were taken for the samples EZ1, EZ3, EZ5, and EZ7. Observed EDX results are shown in . The histogram inserted in the figure clearly depicts the confirmation of Eu doping, which is in a good agreement with our XRD results. The amount of Eu in the samples shows the expected trend.
FTIR Analysis
The FTIR spectrum was recorded to determine the vibrational and functional groups present in the samples. It also interprets the differences in vibrational, intermolecular absorption peaks and intramolecular interactions as a result of Eu doping into the ZnS lattice [Citation37]. shows FTIR spectra of Eu-doped ZnS NPs recorded in the wavenumber range of 4000 to 500 cm−1. All produced samples reveal nearly identical spectra, with negligible shifts in the Zn-S absorption band. The spectra of Eu-doped ZnS exhibit strong absorption peaks at 609, 1117, 1521, 1683, and 3700 cm−1. The observed absorption band at 609 cm−1 corresponds to the stretching of the Zn-S bond i.e. of the host material. The bands detected in the range of 3600–3800 cm−1 are assigned to the stretching mode of O-H of water molecules [Citation38]. Another prominent peak at 1683 cm−1 may be attributed to the O-H bending mode because of the moisture present on the surface of the samples [Citation39]. The band observed around 1117 cm−1 in all the samples corresponds to the formation of microstructural Eu-doped ZnS in the samples, which was also reported by Gawai et al. [Citation37]. The peaks arising at 1521 cm−1 and 2307 cm−1 are assigned to C=O stretching vibration modes which show up from atmospheric CO2 on the surface of the samples [Citation40]. No peaks linked to Eu-related bond are observed probably because of its very small presence in the sample as a dopant.
UV-Vis analysis
To realise the nano size effect and the impact of Eu doping on optical band gap, we performed the UV-Vis measurement of all the samples within the wavelength range 200-800 nm. Optical band gap ( of the samples were calculated using Tauc’s equation as given below [Citation5]:
Where is the absorption coefficient,
is energy of the photons,
is the optical band gap.
The band gap was evaluated from a graph presented in as vs.
. We observed the band gap values are 3.47, 3.55, 3.50, 3.42 eV which are sufficiently lower than the bulk reported values (3.6 eV) [Citation5]. Observed red-shift in the band gaps of synthesised particles may be attributed to the increase in crystallite size of Eu-doped ZnS NPs [Citation41]. Similar kind of nature was reported by Mahanthappa et al. [Citation42] and Bhushan et al. [Citation43] in their report for ZnS NPs prepared following sol-gel synthesis method.
PL analysis
presents the photoluminescence (PL) spectra of Eu-doped ZnS NPs obtained at room temperature under the identical excitation circumstances. The emission spectra of the samples were recorded using 320 nm excitation wavelength. Samples were dissolved in ethanol and a good dispersion of the NPs was created using a sonicator. As can be seen in the emission spectra, the excitation has produced five emission peaks at 360, 437, 450, 466, and 560 nm in all four samples. According to T T Q Hoa et al. [Citation44], the peak observed at near UV region (360 nm) originates due to the creation of defect and surface states developed in between the conduction and valence band of the energy band diagram. It has an impact on controlling optical properties of the samples [Citation34,Citation45]. Few weak peaks can be observed within the range 430 to 470 nm in our samples. According to reported literature, the peak at 437 nm is caused by interstitial zinc, whereas the peak at 450 is caused by Sulphur vacancies [Citation46]. Again, according to Poornaprakash et al. [Citation47] the emission peak arising at around 440–460 nm, may correspond to the blue emission which is a signature of electron-hole recombination in ZnS nanocrystals and it increases due to Eu inclusion. Eu itself is a well-known luminescence material which used as a dopant in Zn-sulphide/oxide to increase the photoluminescence intensity of such materials which makes them more versatile to customise the light emitting devices [Citation48,Citation49]. A strong correlation between the s-p electrons of ZnS and the d-f electrons of the-doped Eu is the origin of such noticeable luminescence in Eu-doped ZnS NPs [Citation41]. The electronic configuration of the Eu3+ ions shows that outermost f-electrons, i.e. 4f6, in general, acts as luminescence centres during the transition from higher excited to lower excited states i.e. from d to f states. An interesting nature of PL can be observed in for sample EZ5 (5at%). It can be noticed that there is a shifting in peaks, while measured in similar environment, with respect to other samples. The observation may be supported by the fact that during doping, there is a chance that defect states were created in the ZnS host matrix. Zn2+ ions were replaced by Eu3+ ions after successful doping, creating a charge imbalance in the samples, and to balance the situation, defects were created. The shifting is a result of luminescence coming out of such states. According, to Wang et al. [Citation50] 5 at% Eu doping in the ZnS matrix may be considered as an optimum doping concentration where it shows maximum luminescence and other optical properties favourable to optoelectronic applications.
FESEM analysis
The morphology of synthesised samples and particle size distribution are presented in . The field emission scanning electron microscopy (FESEM) results reveal that the synthesised powder with a low Eu concentration (1 at%) have the agglomerates to formed taking shapes of tiny flakes-like structure. The tendency to grow in a smaller particle-like structure is more visible with higher resolution () for the same sample. As said, the tendency to form random-shaped particle-like structures increases with an increase of Eu concentration, and a comparatively prominent, well-defined particle-like assembly is visible in when 5 at% Eu is included in the ZnS matrix as a dopant. It also establishes the inference made by Wang et al. [Citation50] that Eu ions, when-doped in 5 at% within the ZnS matrix, show better morphology than others. Now, if we increase the amount of doping, the growth continues and shows a densely packed, smaller particle-like structure. To understand, the average size distribution of particles in different size ranges is plotted (), which shows an increase in size with doping to support our consideration of the agglomeration of particles. The particle sizes obtained from FESEM histograms () show a persistent increase with Eu doping as shown in . The band gap decreases when the Eu level is 3 at.% and above in the ZnS matrix. It has been observed in multiple studies that the band gap decreases with an increase in the particle size. Such reports are supported by the quantum confinement theory, which suggests that the holes in the valence band and the electrons in the conduction band are confined by the potential barriers of the surface. As the particle size decreases, the band gap energy between the valence band and the conduction band increases due to the electron and hole confinement [Citation51]. Hence, it can be concluded that the Eu doping promotes the growth of particle sizes which eventually results in the decrement of the band gap.
Figure 9. FESEM images of Eu-doped ZnS nanoparticles. Images (a) to (d) correspond to FESEM micrograms with lower magnification (x50K) for samples EZ1 (1 at% Eu-doped ZnS), EZ3 (3 at% Eu-doped ZnS), EZ5 (5 at% Eu-doped ZnS) and EZ7 (7 at% Eu-doped ZnS), respectively. Images (e) to (h) are magnified (x100K) views of the FESEM images of EZ1 to EZ7, respectively.

Figure 10. Histogram to show the particle size distribution obtained from FESEM micrographs of all Eu-doped ZnS samples. Fig. (a), (b), (c), and (d) correspond to the samples EZ1, EZ3, EZ5 and EZ7. The average particle varies from within 7.62–8.57 nm. The histograms depict an increase in particle size with increases in Eu doping percentage.

Conclusion
Eu-doped ZnS NPs (1, 3, 5 and 7 at.%) were obtained successfully by sol-gel co-precipitation. The XRD confirms the zinc blend phase of ZnS and particle sizes obtained by Scherrer equation lie in the range of 5.0 to 7.2 nm. Such Eu-doped ZnS NPs show a band gap decrement at higher level of doping. Both the UV and visible emission peaks are observed in the photoluminescence emission spectra whose origins are mostly based on several defect states. Random-shaped particle-like structures that effectively have a persistent reduction of size are seen in FESEM. The particle size is found to govern the band gap at higher doping levels. Hence, it can be concluded that Eu doping in ZnS impacts the morphology of NPs which eventually affects the band gap.
Acknowledgments
The authors acknowledge Central Analytical Facility (CAF), MUJ, for UV–Vis, FTIR and PL measurements. Sophisticated Analytical Instrumentation Facility (SAIF) of MUJ, India, for FESEM measurements, is also duly acknowledged. Priyanka Kumari thankfully acknowledges the financial support in the form of Ramdas Pai Scholarship awarded by Manipal University Jaipur (MUJ).
Disclosure statement
No potential conflict of interest was reported by the authors.
References
- Fang D, Golberg Y, Zhai X, et al. ZnS nanostructures: from synthesis to applications, Prog. Mater Sci. 2011;56(2):175–10. doi: 10.1016/J.PMATSCI.2010.10.001
- Xia Y, Yang P, Sun Y, et al. One-dimensional nanostructures: synthesis, characterization, and applications. Adv Mater. 2003;15(5):353–389. doi: 10.1002/adma.200390087
- Fang X, Bando Y, Gautam UK, et al. ZnO and ZnS nanostructures: ultraviolet-light emitters, lasers, and sensors. Crit Rev Solid State Mater Sci. 2009;34:190–223. doi: 10.1080/10408430903245393
- Kuchibhatla SVNT, Karakoti AS, Bera D, et al. One dimensional nanostructured materials, Prog. Mater Sci. 2007;52(5):699–913. doi: 10.1016/j.pmatsci.2006.08.001
- Kumari P, Misra KP, Samanta S, et al. Interrelation of micro-strain, energy band gap and PL intensity in ce doped ZnS quantum structures. J Lumin. 2022;251:119258. doi: 10.1016/J.JLUMIN.2022.119258
- Das Gupta P, Chattopadhyay S, Choudhary RJ, et al. Band offset in Zn0.965Cd0.035O/ZnO bilayer films. Mater Lett. 2011;65(13):2073–2075. doi: 10.1016/j.matlet.2011.03.109
- Chattopadhyay S, Sen P, Andrews JT, et al. Effect of shell and shell thickness on photoluminescence (PL) of a CdSe/ZnS core – shell quantum dot. J Phys Conf Ser. 2012;365:12037. doi: 10.1088/1742-6596/365/1/012037
- Aboelwafa MA, Abdelghany AM, Meikhail MS. Preparation, characterization, and antibacterial activity of ZnS-NP’s filled Polyvinylpyrrolidone/Chitosan thin films, Biointerface Res. Appl Chem. 2021;11:14336–14343. doi: 10.33263/BRIAC116.1433614343
- Zhou K, Yuan Y, Chen J, et al. Fabrication of antireflective hydrophobic periodic micro-hole structures on ZnS surface by femtosecond laser direct writing. J Phys Conf Ser. 2021;1885:032080. doi: 10.1088/1742-6596/1885/3/032080
- Dhupar A, Kumar S, Tuli HS, et al. In-doped ZnS nanoparticles: structural, morphological, optical and antibacterial properties. Appl Phys A. 2021;127:1–11. doi: 10.1007/S00339-021-04425-9
- Garcia-Calvo E, Cabezas-Sanchez P, Luque-Garcia JL. In-vitro and in-vivo evaluation of the molecular mechanisms involved in the toxicity associated to CdSe/ZnS quantum dots exposure. Chemosphere. 2021;263:128170. doi: 10.1016/J.CHEMOSPHERE.2020.128170
- Pourmoslemi S, Seif F, Mahjub R. Enhanced antibacterial activity of ag-doped ZnS nanoparticles synthesised by a microwave-assisted polyol method, Mater. Res Innov. 2021;25(7):399–403. doi: 10.1080/14328917.2020.1831151
- Fang X, Zhai T, Gautam UK, et al. ZnS nanostructures: from synthesis to applications, Prog. Mater Sci. 2011;56(2):175–287. doi: 10.1016/J.PMATSCI.2010.10.001
- Dengo N, Vittadini A, Natile MM, et al. In-depth study of ZnS nanoparticle surface properties with a combined experimental and theoretical approach. J Phys Chem C. 2020;124(14):7777–7789. doi: 10.1021/acs.jpcc.9b11323
- Kumawat A, Misra KP, Chattopadhyay S. Band gap engineering and relationship with luminescence in rare-earth elements doped ZnO: an overview, Mater. Technol. 2022;37(11):1595–1610. doi: 10.1080/10667857.2022.2082351
- Kumari P, Misra KP, Chattopadhyay S, et al. A brief review on transition metal ion doped ZnO nanoparticles and its optoelectronic applications, Mater. Today Proc. 2021;43:3297–3302. doi: 10.1016/j.matpr.2021.02.299
- Islam F, Shohag S, Uddin M, et al. Exploring the journey of zinc oxide nanoparticles (ZnO-NPs) toward biomedical applications. Materials. 2022;15:2160. doi: 10.3390/ma15062160
- Shanmugam N, Cholan S, Viruthagiri G, et al. Synthesis and characterization of Ce3+-doped flowerlike ZnS nanorods. Appl Nanosci. 2014;4(3):359–365. doi: 10.1007/s13204-013-0217-x
- Manzoor K, Vadera SR, Kumar N, et al. Multicolor electroluminescent devices using doped ZnS nanocrystals. Appl Phys Lett. 2004;84:284–286. doi: 10.1063/1.1639935
- Hu H, Zhang W. Synthesis and properties of transition metals and rare-earth metals doped ZnS nanoparticles. Opt Mater (Amst). 2006;28:536–550. doi: 10.1016/J.OPTMAT.2005.03.015
- Kim MR, Chung JH, Jang D-J. Spectroscopy and dynamics of Mn2+ in ZnS nanoparticles. Phys Chem Chem Phys. 2009;11:1003–1006. doi: 10.1039/B813452A
- Bol AA, Meijerink A. Luminescence of nanocrystalline ZnS:Pb2+. Phys Chem Chem Phys. 2001;3:2105–2112. doi: 10.1039/B100968K
- Dharani Devi M, Vimala Juliet A, Ade R, et al. Enhancement in optoelectronic properties of europium-doped ZnS thin films prepared by nebulizer spray technique for UV photodetection applications. Mater Sci Semicond Process. 2022;144(2022):106572. doi: 10.1016/j.mssp.2022.106572
- Wang X, Zhang H, Li J, et al. Effect of Eu doping concentration on the morphologies and optical properties of ZnO film prepared by ultrasonic spray pyrolysis. J Mater Sci Mater Electron. 2013;24:1883–1887. doi: 10.1007/s10854-012-1029-6
- Yılmaz S, Polat İ, Tomakin M, et al. A research on growth and characterization of CdS: Eu thin films. Appl Phys A. 2019;125:67. doi: 10.1007/s00339-018-2369-8
- Rivera-Medina MJ, Hernández-Torres J, Boldú-Olaizola JL, et al. Synthesis of europium-doped ZnS nano-crystalline thin films with strong blue photoluminescence. RSC Adv. 2016;6(109):107613–107621. doi: 10.1039/c6ra24300b
- Kumawat A, Chattopadhyay S, Misra KP. Significant impact of co-doping Eu-doped ZnO nanoparticles with Li on structural–optical properties relationship. Mater Technol. 2023;38(1):2253646. doi: 10.1080/10667857.2023.2253646
- Sharma A, Khangarot RK, Chattopadhyay S, et al. Band gap reduction and improved ferromagnetic ordering via bound magnetic polarons in Zn (Al, Ce) O nanoparticles. Mater Technol. 2023;38(1):2151114. doi: 10.1080/10667857.2022.2151114
- Kumawat A, Misra KP, Chattopadhyay S. Band gap engineering and relationship with luminescence in rare-earth elements doped ZnO: an overview. Mater Technol. 2022;37(11):1595–1610. doi: 10.1080/10667857.2022.2082351
- Sharma A, Khangarot RK, Kumar N, et al. Rise in UV and blue emission and reduction of surface roughness due to the presence of Ag and Al in monocrystalline ZnO films grown by sol-gel spin coating. Mater Technol. 2021;36(9):541–551. doi: 10.1080/10667857.2020.1776029
- Misra KP, Kumawat A, Shahee A, et al. Effect on optical and structural parameters in heavy Ca-doped ZnO nanostructures. Mater Technol. 2021;36(9):529–540. doi: 10.1080/10667857.2020.1776028
- Misra KP, Jain S, Agarwala A, et al. Effective mass model supported band gap variation in cobalt-doped ZnO nanoparticles obtained by co-precipitation. Semiconductors. 2020;54(3):311–316. doi: https://doi.org/10.1134/S1063782620030136
- Jothibas M, Manoharan C, Johnson Jeyakumar S, et al. Synthesis and enhanced photocatalytic property of Ni doped ZnS nanoparticles, sol. Solar Energy. 2018;159:434–443. doi: 10.1016/J.SOLENER.2017.10.055
- Sagar CKK, Sajan P, Bushiri MJ. Eu3+ and Cu2+ ions doped ZnS microspheres emission in the yellow–orange region. J Mater Sci Mater Electron. 2019;30(19):18220–18226. doi: https://doi.org/10.1007/s10854-019-02176-1
- Chattopadhyay S, Misra KP, Agarwala A, et al. Correlated quartic variation of band gap and NBE energy in sol-gel derived Zn1−xCoxO nanoparticles. Mater Chem Phys. 2019;227:236–241. doi: 10.1016/J.MATCHEMPHYS.2019.02.003
- Kole AK, Kumbhakar P. Cubic-to-hexagonal phase transition and optical properties of chemically synthesized ZnS nanocrystals. Results Phys. 2012;2:150–155. doi: 10.1016/j.rinp.2012.09.010
- Gawai UP, Khawal HA, Shripathi T, et al. A study on the synthesis, pair distribution function and diverse properties of cobalt doped ZnS nanowires. Cryst Eng Comm. 2016;18(8):1439–1445. doi: https://doi.org/10.1039/C5CE02253C
- Iranmanesh P, Saeednia S, Nourzpoor M. Characterization of ZnS nanoparticles synthesized by co-precipitation method. Chinese Phys B. 2015;24(4):046104. doi: https://doi.org/10.1088/1674-1056/24/4/046104
- Goswami N, Sen P. Water-induced stabilization of ZnS nanoparticles. Solid State Commun. 2004;132(11):791–794. doi: 10.1016/J.SSC.2004.09.022
- Rana MS, Das SK, Rahman MO, et al. Vanadium doped ZnS nanoparticles: effect of vanadium concentration on structural, optical and electrical properties. Trans Electr Electron Mater. 2021;22(5):612–621. doi: https://doi.org/10.1007/S42341-020-00265-1
- Singh Lotey G, Jindal Z, Singhi V, et al. Structural and photoluminescence properties of Eu-doped ZnS nanoparticles. Mater Sci Semicond Process. 2013;16(6):2044–2050. doi: 10.1016/j.mssp.2013.07.039
- Mahanthappa M, Kottam N, Yellappa S. Electrocatalytic performance of a zinc sulphide nanoparticles-modified carbon paste electrode for the simultaneous determination of acetaminophen, guanine and adenine. Anal Methods. 2018;10(11):1362–1371. doi: https://doi.org/10.1039/C8AY00007G
- Bhushan M, Jha R, Bhardwaj R. Reduced band gap and diffusion controlled spherical n-type ZnS nanoparticles for absorption of UV-Vis region of solar spectrum. J Phys Chem Solids. 2019;135:109021. doi: 10.1016/J.JPCS.2019.05.018
- Quynh Hoa TT, Van Vu L, Canh TD, et al. Preparation of ZnS nanoparticles by hydrothermal method, in: j. Phys. Conf. Ser. Institute of Physics Publishing; 2009. p. 12081. doi: 10.1088/1742-6596/187/1/012081
- Liu X, Cui J, Zhang L, et al. A solvothermal route to semiconductor ZnS micrometer hollow spheres with strong photoluminescence properties, Mater. Lett. 2006;60(20):2465–2469. doi: 10.1016/j.matlet.2006.01.019
- Pal M, Mathews NR, Morales ER, et al. Synthesis of Eu+3 doped ZnS nanoparticles by a wet chemical route and its characterization. Opt Mater (Amst). 2013;35:2664–2669. doi: 10.1016/J.OPTMAT.2013.08.003
- Poornaprakash B, Vattikuti SVP, Subramanyam K, et al. Photoluminescence and hydrogen evolution properties of ZnS:Eu quantum dots. Ceram Int. 2021;47(20):28976–28984. doi: 10.1016/j.ceramint.2021.07.058
- Ahemen I, De DK, Melludu OC. Synthesis and characterization of europium-doped zinc sulphide (ZnS: eu) nano particles: nano Red Phosphor. Adv Sci Eng Med. 2013;5(11):1188–1194. doi: 10.1166/asem.2013.1403
- Bindu KR, Safeera TA, Anila EI. Pure red luminescence and concentration-dependent tunable emission color from europium-doped zinc sulfide nanoparticles. J Mater Sci Mater Electron. 2022;33(22):17793–17801. doi: https://doi.org/10.1007/s10854-022-08644-5
- Wang L, Xu X, Yuan X. Preparation and photoluminescent properties of doped nanoparticles of ZnS by solid-state reaction. J Lumin. 2010;130(1):137–140. doi: 10.1016/j.jlumin.2009.07.036
- Singh M, Goyal M, Devlal K. Size and shape effects on the band gap of semiconductor compound nanomaterials. J Taibah Univ Sci. 2018;12(4):470–475. doi: 10.1080/16583655.2018.1473946