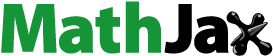
ABSTRACT
Photoconductivity and photoluminescence of highly transparent predominantly c-axis oriented hexagonal wurtzite nanocrystalline Mg doped zinc oxide thin films are reported here. These films prepared by sol-gel spin coating are useful for switching and UV detector applications. The increase in dopant concentration tunes the optical band-gap from 3.15 to 3.41 eV. Amplified NBE emission with low defect emission is obtained in appropriately doped sample along with high suitability for switching applications since the photocurrent sharply falls to a very low value as the illumination is put off and the rise and decay pattern is reproducible for several consecutive cycles. The films have crystallite size less than 16 nm and rod-like structures of diameter 50 to 100 nm are seen to grow out of spherical granular background. Doping reduces the size of grains and rods as well as the surface roughness.
Introduction
ZnO, being a versatile direct wide band gap semiconductor material, finds application in transparent semiconductors, electronics, optoelectronics, photocatalysts, UV/blue LED, photodetectors, piezoelectric devices, gas sensors, surface acoustic – wave devices, acousto-optic devices and short wavelength semiconductor diode lasers [Citation1–6]. By introducing dopant in varying amounts in ZnO, the band gap energy and luminescence can be controlled [Citation4]. MgO is a compound semiconductor with a very wide band gap of 7.8 eV, and the doping of Mg in ZnO leads to an increase in the band gap depending on the Mg content [Citation7]. Mg doped ZnO have attracted much attention because of their unique property of luminescence in UV and visible regions of the spectrum. Band gap engineering in ZnO thin films have been achieved by moderate contents of MgO and CdO, MgZnO and CdZnO alloys retain the wurtzite crystal structure of the parent compound, providing a wide range of band gaps (2.3–4.0 eV) for practical device applications [Citation8–13]. Synthesis of films or nanostructures using different deposition methods results in varying nanostructures and crystallite orientation which alter the photoresponse significantly [Citation14]. Nature of photoconductivity (PC) relaxation under UV illumination is generally related with the adsorption or desorption of oxygen species at the film surface or grain boundaries [Citation14,Citation15]. In conventional semiconductors as soon as the illumination is turned off the photogenerated electrons and holes quickly disappear since the underlying solid-state process is very fast. Fortunately, ZnO based materials show comparatively slow photoresponse and so are much more useful [Citation16]. Reports about photoluminescence (PL) in Mg doped ZnO thin films are in abundance but there are very few reports on PC [Citation17–20] in such films. The current-voltage (I-V) characteristics may have been studied [Citation19,Citation20] but reports on photoresponse could scarcely be seen [Citation17,Citation18,Citation21]. In this paper, optical band gap, photoluminescence, photoconductivity and surface morphology along with composition and structure of nanocrystalline Mg doped ZnO thin films (ZnO:Mg) prepared by sol-gel spin coating method [Citation22], are reported.
Experimental
Synthesis
0.2M solution of zinc acetate dihydrate (99.99% from sigma Aldrich) is prepared in isopropanol (99.9% pure, Merck). Small amount of diethanolamine (99.9% pure, Ranbaxy chemicals) is added to achieve a viscous solution. The mixture is magnetically stirred at 60°C for half an hour for homogenization, which serves as the precursor solution for depositing undoped ZnO films. Three other precursor solutions having magnesium doping of 5, 10 and 15% are prepared, for which appropriate volumes of 0.2 M solution of magnesium chloride (99.5% pure, Ranbaxy chemicals) in isopropanol (99.9% pure, Merck) are added to the earlier obtained precursor solution for undoped ZnO. These solutions are again stirred for half an hour at 60°C. Both undoped and doped precursor solutions are aged for 5 days to obtained proper viscosity and sol stability. It helps in achieving the desired thickness and uniformity of the films.
The precursor solutions thus obtained are coated on properly cleaned substrates one by one, using photoresist spinner unit. Two drops of the precursor solution are put on the glass substrate which is fixed properly to the spinner unit. It is then spun at the speed of 3000rpm for 30 sec. After each coating the sample is heated from room temperature to 400°C at the rate of 10°C/min for 1 hour and then cooled back to room temperature naturally. This process is repeated 25 times for obtaining appreciable thickness. The precursor films thus obtained are annealed at 450°C for four hours. Thus, four samples are prepared i.e. ZnO; ZnO:5at% Mg, ZnO:10at% Mg and ZnO:15at% Mg, which are named as samples ZM0, ZM1, ZM2 and ZM3, respectively.
Characterization
The crystal phase and crystallinity of the samples have been investigated using X-Ray diffractometer (Model – Rigaku Ultima IV installed at our department) for 2θ values ranging from 25 to 70º using CuKα radiation (λ = 1.5418Å). Transmittance and PL spectra have been recorded using UV-Vis spectrophotometer (Model- V670, Jasco) and fluorescence spectrometer (Model- LS-55, Perkin Elmer) respectively. Thickness of film was measured by Dektak Surface Profilometer (Model No. Veeco150). FESEM and EDX have been done to obtain surface morphology and elemental composition. Topology of films are recorded AFM (Nanomagnetics Instruments). The photoconductivity characteristics of the samples, at room temperature, have been obtained by studying the current-voltage (I – V) characteristics and rise and decay of photocurrent. Electrical contacts on the film were made using silver paste with electrode spacing of 10 mm. The area of illumination is 1 cm2 and the photo-excitation source is a 300W UV lamp. Dark current (Idc) in absence of light and photocurrent (Ipc) under ultraviolet illumination of 365 nm are measured using multimeter (RISH Multi-18S) with adapter RISH Multi SI-232. All the measurements have been performed at room temperature [Citation4].
Results and discussion
X – ray diffraction
The XRD patterns for the samples are shown in . All the films are of polycrystalline nature having three major peaks along (100), (002) and (101) planes indicating hexagonal wurtzite phase of ZnO with small peaks along (102), (110), (103) and (112) [Citation4,Citation23–25]. No impurity phase of MgO is observed in doped samples indicating that the Zn2+ ions are successfully substituted by Mg2+ ions in the ZnO lattice. The XRD peak along c-axis, i.e. (002) plane is prominent and occurs at 2θ = 34.52°, 34.50, 34.38° and 34.44° for samples ZM0, ZM1, ZM2 and ZM3 respectively. This change in 2θ along (002) plane indicates that there is regular change in the volume of hexagonal cell of ZnO due to doping of Mg. The angle of diffraction regarding (002) plane is highest for undoped ZnO. The orientation parameter γ(hkl) is calculated from the relative heights of the (100), (002), (101), (102), (110), (103) and (112) reflection peaks [Citation4,Citation23]. The values of orientation parameter vary from 0.0.036 to 0.329 and are given in , indicating that all the films are preferably oriented along (002) plane except sample ZM3. The crystallite size tDS in the samples estimated by Debye-Scherer formula [Citation24,Citation26], along (100), (002) and (101) crystallographic planes lies between 3 to 16 nm, . Williamson and Hall plot has been performed and is shown in . The trend line equations obtained from for the four samples are y = −1.046× + 0.33, y = −0.794× + 0.253, y = −0.488× + 0.159 and y = −0.488× + 0.159. Comparison of these equations with βcosθ = Cλ/tWH +2ε sinθ [Citation27,Citation28], determines the strain and particle size in the film samples. The value of strain varies from 6.95 × 10−2 to −5.23 × 10−1 as given in . The particle size tWH obtained by WH plot are very small as compared to tDS. A sufficient amount of compressive strain exists in all samples with least strain in sample ZM3. Since strain in all samples is large and the crystallite size obtained by the two methods are quite different, it is clear that the broadening of FWHM is due to strain existing in the films and not due to the crystallite size. The lattice constants [Citation4,Citation29] a and c for ZnO and Mg doped ZnO thin films are mentioned in . The a-lattice constant of ZnO thin film is larger than those of doped samples ZM1 and ZM2 and is same for sample ZM3. The c-lattice constant increases monotonically, with increment in doping, from 5.199 to 5.211 Å. Increment in lattice constant c occurs in spite of the Zn++ ion being little large (0.74 Å) as compared to Mg++ ion (0.65 Å) indicating that the Mg++ lodges in the interstitials also. The bond length [Citation4] lies between 1.977and 1.979 Å. It decreases for sample ZM1, increases for sample ZM3 and remains same for sample ZM0 and ZM2 (). The dislocation density δ [Citation28] for the undoped and doped samples, calculated along each plane, varies randomly from 40.15 × 108 to 1162.63 × 108 lines/mm2
Figure 1. XRD patterns of ZnO and mg doped ZnO thin films. Here a, b, c and d correspond to samples ZM0, ZM1, ZM2 and ZM3 respectively.

Figure 2. Williamson-hall plots for ZnO and mg doped ZnO thin films. Here a, b, c and d correspond to samples ZM0, ZM1, ZM2 and ZM3 respectively.

Table 1. The orientation parameter γ(hkl) along different planes for undoped and mg doped ZnO thin films.
Table 2. Particle size determined by DS formula and WH plot, strain, lattice constant and bond length for undoped and mg doped ZnO thin films.
EDX analysis
The EDX spectra, , revealed the presence of Zn and O as the only elementary species in the sample ZM0 i.e. undoped ZnO. For doped samples the presence of Mg is also confirmed, besides Zn and O. The amount of Mg in the solid films is found to be 3.8, 8.7 and 12. 5at.% which is lesser than those in the corresponding precursor solutions where it was 5, 10 and 15at%, respectively.
Surface morphology
FESEM of the four samples have been performed and the micrographs are shown in . The surface of undoped ZnO film shows rod-like structures grown on a granular background. The diameter of these rods varies from 50 to 100 nm and the size of grains in background varies from 25 to 35 nm throughout the film surface. For sample ZM1, spherical grains in the background reduce in size and coalesce and randomly oriented rods still remain. Spherical grains become clear for sample ZM2 and few new rods grow up. For sample ZM3 the rods growing out of the spherical grain background become thinner. Thus, randomly oriented rods with different dimensions grow up in all the samples.
Atomic force microscopy
The two- and three-dimensional AFM images of all the thin film samples over a scanning area 2 × 2 µm2 are presented in , respectively. Sample ZM0 shows the cluster of grains whereas in sample ZM1 clear spherical grains are observed with some nanorods. In samples ZM2 and ZM3 rod-like structures occur. The RMS roughness is found to be 50.5, 15.2, 40.5 and 36.8 nm for samples ZM0, ZM1, ZM2 and ZM3,respectively. The RMS roughness is highest for undoped sample and it decreased for doped samples. First the RMS roughness decreased for sample ZM1 and then increased for sample ZM2 and again decreased for sample ZM3.
Optical transmission
The films are highly transparent in the wavelength range 350 t0 900 nm as revealed by the transmission spectra shown in . All the films exhibit sharp absorption near 375 nm (3.30 eV) as the optical band gap of ZnO/ZnO based films lies in the UV range. The absorption edge undergoes a blue shift as dopant is increased. The transmittance for all the films varies between 82 to 92% in visible and infrared region. The transparency depends upon crystallinity, homogeneity, thickness, annealing temperature and dopant. Transmission increases with increase in doping amount up to 8.7at.% and decreases for 12.5at% Mg doping. The thicknesses of samples ZM0, ZM1, ZM2 and ZM3 are found to be 140, 115,100 and 160 nm, respectively.
Optical band gap
The band gap energy Eg of undoped and Mg doped thin films is determined by Tauc’s plot i.e. the graph between (αhν)2 versus hν [Citation28,Citation30,Citation31]. Thus, the values of band gap are found to be 3.15, 3.32, 3.38 and 3.41 eV for samples ZM0, ZM1, ZM2 and ZM3, respectively, . A systematic enhancement of band gap from sample ZM0 to ZM3 is an indicative of proper crystallinity. Thus, the total band gap enhancement or in other words a blue shift of 8.25% is obtained by doping Mg up to 12.5at. %. The monotonic variation in the band gap with Mg concentration is shown in the inset of . The linear fit to this variation is obtained as Eg(x) = 0.019 × +3.192. Band gap variation from some earlier reports on Mg doped ZnO thin films are presented here. Huang et al reported that the optical band gap varies between 3.26 to 3.34 eV, for 0 to 8at% Mg doped ZnO thin films, respectively, i.e only 2.45% enhancement is obtained for 8at.% Mg doping [Citation32]. Ding et al reported 6.04% band gap enhancement for 3.8at%Mg doping i.e. band gap variation between 3.31 to 3.51 eV [Citation33]. Kim et al. showed a 14.4% increment, from 3.26 to 3.73 eV, in band gap as Mg content increased from 0 to 30% [Citation34]. An increase of 8.92% in band gap, from 3.36 to 3.66 eV, by 10% doping of Mg in ZnO films prepared by modified Pechini method is reported by Fang et al. [Citation35]. Shan et al reported an optical band gap tuning between 3.274 to 3.430 eV for 5at.% doping of Mg i.e. an enhancement of 4.76% [Citation1]. All these references report a blue shift in optical band gap. Our result compares favourably well with the first three of the references stated here. It should be noted that new defects appear after Mg ions substitute Zn ions, due to difference in the electronegativity and ionic radius. ZnO is an n-type semiconductor and therefore the Fermi level is inside the conduction band. Mg dopant, due to lower electron affinity of MgO as compared to ZnO contributes more electrons, which raises the Fermi level for the Mg-doped ZnO thin films and consequently increases the optical band gap which is also termed as Burstein-Moss shift. Increase in optical band gap with the Mg concentration indicates the suitability of ZnO:Mg thin film for optoelectronic ZnO based devices. The absorption edge at 375 nm (3.30 eV) corresponds to band-to-band transition from valence band to conduction band [Citation32].
Photoluminescence
The photoluminescence excitation spectra have been recorded for an emission at 393 nm equivalent to 3.15 eV the optical band gap of ZnO film in the present study. Inset of shows possibility of excitation at three wavelengths 256, 266 and 325 nm. Excitation peak at 325 nm is considerably stronger than the other two peaks, hence is used for excitation of the samples here and the photoluminescence spectra are shown in . Curves a, b, c and d correspond to samples ZM0, ZM1, ZM2 and ZM3, respectively. The undoped sample ZM0 exhibits UV emission peak at 399 nm (3.10 eV) with intensity higher than the peaks occurring in visible region i.e. violet-blue emission at ~451 nm (2.74 eV) and blue – green emission at 486 nm (2.55 eV). The UV peak is related to near-band-edge (NBE) emission which is an indication of good crystalline quality of the films and may be attributed to free and bound exciton recombination [Citation36,Citation37]. NBE emission peak shifts to higher energy region monotonically with increase in dopant. It occurs at 395(3.14 eV), at 384(3.23 eV) and at 383 nm (3.24 eV) for samples ZM1, ZM2 and ZM3, respectively. The intensity of NBE emission is more in all doped samples being very high for sample ZM1. The PL emission from sample ZM1 in the UV region is extremely good with suppressed defect-emissions and the intensity of NBE emission is fourteen-fold higher as compared to that from undoped sample. The ratio of the intensities of NBE to defect emissions for individual samples ZM0, ZM1, ZM2 and ZM3 is 1.74, 6.0, 2.40 and 3.12, respectively.
Figure 8. PL spectra for ZnO and mg doped ZnO thin films. Here a, b, c and d correspond to samples ZM0, ZM1, ZM2 and ZM3, respectively. Inset shows PLE spectra for ZnO thin film for emission wavelength 393nm.

If any dopant alters the band gap of the material, then the peak position of band gap emission or NBE emission may change. Peak wavelength of the UV emissions is reported to be blue-shifted, although slightly [Citation38], due to band gap broadening of ZnO by the doping of Mg. Shan et al also reported a blue shift of 10 meV in NBE emission (3.28 eV, 378 nm) in films deposited by PLD [Citation1]. In Ref [Citation35]. in doped samples prepared by modified Pechini method, NBE emission shifts to 368 nm from 371 nm. Also, the PL intensity increases. Ding et al reported blue shift in NBE emission (377 to 354 nm) with increase in intensity by the doping of Mg [Citation33]. Huang et al also reported enhancement in NBE emission intensity with blue shift in emission-peak position from 402 nm to 384 nm [Citation32]. Similar to other references, a blue shift has also been reported by Ref [Citation34], with increase in Mg concentration in samples deposited by laser ablation, but in this case, intensity of NBE emission decreased.
The violet-blue emission from undoped sample centred on 451 nm (2.74 eV) may be attributed to transitions from conduction band to singly ionized VZn− and the blue – green emission centred on ~486 nm (2.55 eV) is due to radiative transition of an electron from the shallow donor level of Zni to an acceptor level of neutral VZn as discussed in Ref [Citation4].
Photoconductivity study
Photocurrent
The photoconductivity study on the Mg doped ZnO sample films ZM0 to ZM3 have been performed. The applied voltage has been varied from 0 to 30 volts and the current is noted. The I – V characteristics for undoped and Mg doped ZnO thin films in dark and in UV illumination are shown in . The plot of dark current as well as photocurrent with applied voltage for all the samples consists of two straight line segments with slopes r1 and r2 corresponding to lower and higher voltage regions respectively which follow the power-law relationship [Citation4]. For undoped ZnO thin film, the variation of the dark current with voltage have slopes greater than 1 (r1 = 1.11, r2 = 1.01) thus showing super-linear behaviour in both the segments. Sample ZM1 shows sub-linear behaviour in both the voltage regions as r1 = 0.53 and r2 = 0.36. Sample ZM2 shows sub-linear (r1 = 0.98, r2 = 0.80) behaviour in both the voltage regions. ZM3 shows sub-linear (r1 = 0.98) behaviour in lower- whereas super-linear (r2 = 1.24) behaviour in higher voltage region ().
Figure 9. Variation of dark current (a) and photo current (b) as a function of voltage in ZnO and mg doped ZnO thin films on log-log scale. Here a, b, c and d correspond to samples ZM0, ZM1, ZM2 and ZM3, respectively.

Table 3. Slopes for dark and photo current for ZnO and mg doped ZnO thin films calculated from .
The photocurrent Ipc for each value of applied voltage is more in doped films compared to that in undoped ZnO film, . The photocurrent exhibits super-linear nature (r > 1) in both the voltage regions for samples ZM0, ZM1, ZM2 and ZM3. For all samples the slopes r > 2 in lower voltage region. Flow of trap-limited as well as space-charge-limited current (SCLC) inside the materials is responsible for this sub- and super- linear behaviour. When higher voltages are applied, one of the electrodes injects excess carriers which lead to SCLC. If surface to volume ratio of a sample is high then the process of adsorption and desorption of chemisorbed oxygen molecules on the film affects the SCLC by depleting free electrons as described in Ref [Citation4].
Photoresponse
The photoresponse is recorded at a fixed voltage of 5 V for all the four samples using OFF, ON for 15 minutes and OFF conditions as described in our earlier article [Citation14]. For sample ZM0, in OFF condition very small dark current appears. For ON condition initially a sudden rise of photocurrent takes place which further increases slowly in the next few minutes () of observation. When UV light is put off i.e. during decay portion, firstly the current falls down suddenly and then decreases slowly. For sample ZM1, in ON condition a sudden rise of photocurrent takes place which quickly gets saturated. When UV light is turned off, initially the current falls off with rapidity greater than in the case of undoped sample followed by slow decrease down to a magnitude near to that of the initial dark current. For sample ZM2, in ON condition photocurrent rises suddenly which quickly gets saturated, remains constant for a short while and then starts falling gradually even under steady UV illumination for remaining time of observation. When UV light is turned off initially the current falls off with rapidity greater than in the case of sample ZM1 followed by slow decrease down to a magnitude near to that of the initial dark current. For sample ZM3, in ON condition a sudden rise of photocurrent takes place which slows down considerably and then further increases slowly. During OFF condition, first the current falls down suddenly, then decreases slowly. The rise time τr is 399, 71, 26 and 125 seconds for samples ZM0, ZM1, ZM2 and ZM3, respectively (). During the ON condition, the holes of generated electron-hole pairs are trapped by the adsorbed O2− through the surface electron-hole recombination, while the unpaired UV-excited electrons significantly increase the conductivity [Citation39]. The photocurrent increases with time until equilibrium occurs in the absorbed and desorbed oxygen. Oxygen is adsorbed from atmosphere on the surface of films according to the equations and
. The desorption of adsorbed oxygen on illumination can be understood according to the equations
,
and
[Citation40,Citation41]. The adsorption of oxygen is more in the present samples since the surface to volume ratio is higher and the electronegativity of dopant Mg is less than that of Zn. Falling of photocurrent even under steady UV illumination is an anomalous behaviour. Similar behaviour has also been reported by others [Citation42]. The decrease in photocurrent during ‘ON’ condition can be attributed to the re-adsorption of oxygen as O−2 ions, thereby depleting free electrons or the recombination of electrons to the Zni recombination centre, or trapping of electron at oxygen vacancy. When UV light is put off decay is quite sharp for undoped ZnO film being even sharper for the doped ones. Using ORIGIN software, it is found that the decay portion of the photo-response curve for all the samples fits well with bi-exponential equation i.e. I = I0 + I1 exp(−t/τd1) + I2 exp(−t/τd2) [Citation10,Citation43]. The two relaxation time constants τd1 and τd2 for sample ZM0 are estimated to be 30.23 and 217.85 seconds respectively from the photoresponse curve a, . For sample ZM1, τd1 and τd2 are estimated to be 17.78 and 179.80 seconds respectively. Relaxation time constants for sample ZM2 are 22.39 and 134.80 seconds whereas for sample ZM3 they are 34.72 and 206.00 seconds, respectively. It can therefore be seen that doped samples give faster photoresponse with considerably lesser rise- and decay- times (τr and τd1), . When the UV illumination is put off, the hole-density is much smaller than electron-density and therefore even after ensuing fast electron-hole recombination there remain many unpaired electrons. Further, in the dark, these unpaired electrons are captured by the oxygen gas molecule which then gets adsorbed on the oxide surface as a negatively charged ion O2−, thus reducing the current slowly to nearly the initial value.
Figure 10. Time resolved rise and decay photocurrent spectrum of undoped and mg doped ZnO thin films. Here a, b, c and d correspond to samples ZM0, ZM1, ZM2 and ZM3 respectively.

Table 4. Rise and decay time constants τr, τd1, τd2, p calculated from and trap depth (E) for undoped and mg doped ZnO thin films.
Photo-switching
The switching performance of all the samples is studied by putting the UV illumination ON and OFF for 2 minute each and repeated five times. shows that the rise and decay pattern of the photocurrent recorded for all the samples is reproducible. In samples ZM0, ZM1, ZM2 and ZM3, in the decay portion of the first cycle, the photocurrent drops down to nearly 34, 11, 4 and 30% of the peak value, repectively. In the next cycle the photocurrent again rises to its respective original peak value, for each sample, becomes constant and then decays to its initial value of the second cycle as can be clearly seen from . Same pattern of rise and decay repeats itself in the next three cycles of observation. Thus, the doped samples except sample ZM3 are suitable for switching applications since the photocurrent sharply falls to less than 5 and 15% of the peak value as the illumination is put off for samples ZM1 and ZM2, respectively, and the rise and decay pattern is reproducible in several consecutive cycles. Sample ZM0 does not offer as good a switching behaviour as doped samples in want of sharp decay. With good photoresponse, photosensitivity and photo switching behaviour Mg doped ZnO films prepared by sol-gel method are potential candidate for related applications. It should be mentioned that there are very few reports on photo-switching of Mg doped ZnO thin films [Citation17,Citation18].
Trap depth determination
The frequency factor S and probability p for traps corresponding to different exponentials are determined by the equations I = I0e−pt and p = Se−E/kT , where I0 and I are the current at the moment the decay starts and at the moment of interruption; E, T, S and k are trap depth, absolute temperature, frequency factor (S = 109 at room temperature) and the Boltzmann constant, respectively [Citation4]. The p-values, determined by the bi-exponential decay portions for all the four samples vary between 1.77 × 10−3 to 28.84 × 10−3 and the trap depths lie between 0.628 to 0.699 eV, .
Conclusion
Highly transparent, c-axis oriented hexagonal wurtzite nanocrystalline ZnO and Mg doped ZnO thin films with crystallite size less than 16 nm have been obtained by sol-gel spin coating. An enhancement of 260 meV is obtained in the band gap by increasing the Mg concentration which amounts to 8.25% increment. NBE emission shows blue shift with increase in Mg dopant concentration. For 3.8at% Mg doped sample NBE emission is extremely good, with 14-fold higher intensity as compared to that from undoped sample, and suppressed defect-emissions.Faster photoresponse is obtained in doped samples as the related rise and decay times decrease. Mg doping in appropriate amount renders ZnO film suitable for switching applications since the photocurrent sharply falls to less than 15% of the peak value as the illumination is put off and the rise and decay pattern is reproducible in several consecutive cycles. With good photoresponse, photosensitivity and photo switching behaviour Mg doped ZnO films prepared by sol-gel method are potential candidate for related applications such as for development of smart materials viz. light-responsive transistors and switches, for improving the efficiency and functionality of solar cells and for environmental monitoring & chemical sensing. FESEM shows rod-like structures of diameter 50 to 100 nm grown on spherical granular background and doping reduces the size of grains and rods. The surface roughness is found to decrease with increase in dopant concentration.
Acknowledgments
Authors are thankful to DST New Delhi India for providing UV-Vis-NIR spectrometer (vide project no.SR/S2/CMP −0028/2010), and to the Department of Biotechnology, New Delhi, Government of India for providing financial assistance vide project no. BT/PR40089/NER/95/1663/2020. Thanks, are also due to Govt. of Uttar Pradesh for providing XRD facility, to DST New Delhi for providing AFM facility through PURSE grant, and to UGC New Delhi for providing Fluorescence spectrometer facility through SAP.
Disclosure statement
No potential conflict of interest was reported by the authors.
References
- Shan FK, Kim BI, Liu GX, et al. Blueshift of near band edge emission in mg doped ZnO thin films and aging. J Appl Phys. 2004;95(9):4772. doi: 10.1063/1.1690091
- Zhang X-M, Ming-Yen L, Zhang Y, et al. Fabrication of a high-brightness blue-light-emitting diode using a ZnO-Nanowire array grown on p-GaN thin film. Adv Mater. 2009;21(27):2767–11. doi: 10.1002/adma.200802686
- Ali GM, Chakrabarti P. ZnO-based interdigitated MSM and MISIM ultraviolet photodetectors. J Phys D Appl Phys. 2010;43(41):415103. doi: 10.1088/0022-3727/43/41/415103
- Kumar N, Srivastava A. Faster photoresponse, enhanced photosensitivity and photoluminescence in nanocrystalline ZnO films suitably doped by cd. J Alloys Compd. 2017;706:438–446. doi: 10.1016/j.jallcom.2017.02.244
- Moret M, Abou A, Chaaya MB, et al. Atomic Layer deposition of zinc oxide for solar cell applications. Superlattices Microstruct. 2014;75:477–484. doi: 10.1016/j.spmi.2014.07.050
- Bie Y-Q, Liao Z-M, Wang P-W, et al. Single ZnO Nanowire/p-type GaN heterojunctions for photovoltaic devices and UV light-emitting diodes. Adv Mater. 2010;22(38):4284–4287. doi: 10.1002/adma.201000985
- Xue L, Xaing L, Peng-Ting L. Mg doping reduced full width at half maximum of the near-band-edge emission in mg doped ZnO films. Chin Phys B. 2010;19(2):027202. doi: 10.1088/1674-1056/19/2/027202
- Ohtomo A, Tamura K, Kawasaki M, et al. Room-temperature stimulated emission of excitons in ZnO/(Mg, Zn)O superlattices. Appl Phys Lett. 2000;77(14):2204. doi: 10.1063/1.1315340
- Jin Y, Zhang B, Shuming Y, et al. Room temperature UV emission of MgxZn1−xO films. Solid State Commun. 2001;119(6):409. doi: 10.1016/S0038-1098(01)00244-7
- Makino T, Segawa Y, Kawasaki M, et al. Band gap engineering based on MgxZn1−xO and CdyZn1−yO ternary alloy films. Appl Phys Lett. 2001;78(9):1237. doi: 10.1063/1.1350632
- Gruber T, Kirchner C, Kling R, et al. Optical and structural analysis of ZnCdO layers grown by metalorganic vapor-phase epitaxy. Appl Phys Lett. 2003;83(16):3290. doi: 10.1063/1.1620674
- Gruber T, Kirchner C, Kling R, et al. ZnMgO epilayers and ZnO–ZnMgO quantum wells for optoelectronic applications in the blue and UV spectral region. Appl Phys Lett. 2004;84(26):5359. doi: 10.1063/1.1767273
- Janotti A, Van de Walle CG. Fundamentals of zinc oxide as a semiconductor. Rep Prog Phys. 2009;72(12):126501. doi: 10.1088/0034-4885/72/12/126501
- Yadav HK, Gupta V. A comparative study of ultraviolet photoconductivity relaxation in zinc oxide (ZnO) thin films deposited by different techniques. J Appl Phys. 2012;111(10):102809. doi: 10.1063/1.4714715
- Li QH, Gao T, Wang YG, et al. Adsorption and desorption of oxygen probed from ZnO nanowire films by photocurrent measurements. Appl Phys Lett. 2005;86(12):123117. doi: 10.1063/1.1883711
- Sharma P, Mansingh A, Sreenivas K. Ultraviolet photoresponse of porous ZnO thin films prepared by unbalanced magnetron sputtering. Appl Phys Lett. 2002;80(4):553–555. doi: 10.1063/1.1445480
- Shewale PS, Yu YS. Structural, surface morphological and UV photodetection properties of pulsed laser deposited mg-doped ZnO nanorods: effect of growth time. J Alloys Compd. 2016;654:79–86. doi: 10.1016/j.jallcom.2015.09.048
- Das A, Roy PG, Dutta A, et al. Mg and al co-doping of ZnO thin films: effect on ultraviolet photoconductivity. Sci Semicond Process. 2016;54:36–41. doi: 10.1016/j.mssp.2016.06.018
- Jiang DY, Zhang JY, Liu KW, et al. A high-speed photoconductive UV detector based on an mg 0.4 zn 0.6 O thin film. Sci Technol. 2007;22(7):687–690. doi: 10.1088/0268-1242/22/7/001
- Young S-L, Kao M-C, Chen H-Z, et al. Mg doping effect on the microstructural and optical properties of ZnO nanocrystalline films. J Nanomater. 2015;2015:1–5. doi: 10.1155/2015/627650
- Kumar N, Srivastava A. Green photoluminescence and photoconductivity from screen-printed mg doped ZnO films. J Alloys Compd. 2018;735:312–318. doi: 10.1016/j.jallcom.2017.11.024
- Sharma A, Khangarot RK, Chattopadhyay S, et al. Band gap reduction and improved ferromagnetic ordering via bound magnetic polarons in Zn(Al, Ce)O nanoparticles. Mater Technol. 2023;38(1):2151114. doi: 10.1080/10667857.2022.2151114
- Kumawat A, Chattopadhyay S, Misra KP. Significant impact of co-doping eu-doped ZnO nanoparticles with Li on structural–optical properties relationship. Mater Technol. 2023;38(1):2253646. doi: 10.1080/10667857.2023.2253646
- Srivastava A, Kumar N, Khare S. Enhancement in UV emission and band gap by fe doping in ZnO thin films. Opto-Electronics Review. 2014;22(1):68–76. doi: 10.2478/s11772-014-0179-x
- Srivastava A, Shukla RK, Misra KP. Photoluminescence from screen printed ZnO based nanocrystalline films. Cryst Res Technol. 2011;46(9):949–955. doi: 10.1002/crat.201100155
- Kumawat A, Misra KP, Chattopadhyay S. Band Gap Engineering and relationship with luminescence in rare-earth elements doped ZnO: an overview. Mater Technol. 2022;37(11):1595–1610. doi: 10.1080/10667857.2022.2082351
- Sharma A, Kumawat A, Chattopadhyay S, et al. Low temperature induced red-shift in violet-blue emission from Zn(Al, Ag)O nanoparticles. Mater Technol. 2022;37(11):1629–1638. doi: 10.1080/10667857.2021.1967550
- Srivastava A, Kumar N, Misra KP, et al. Enhancement of band gap of ZnO nanocrystalline films at a faster rate using sr dopant. Electron Mater Lett. 2014;10(4):703–711. doi: 10.1007/s13391-014-3131-9
- Sharma A, Khangarot RK, Kumar N, et al. Rise in UV and blue emission and reduction of surface roughness due to the presence of ag and al in monocrystalline ZnO films grown by sol-gel spin coating. Mater Technol. 2021;36(9):541–551. doi: 10.1080/10667857.2020.1776029
- Srivastava A, Kumar N, Misra KP, et al. Blue-light luminescence enhancement and increased band gap from calcium-doped zinc oxide nanoparticle films. Mater Sci Semicond Process. 2014;26:259–266. doi: 10.1016/j.mssp.2014.05.001
- Feng J, Tong SY, Thian ES, et al. Nanostructuring of biomaterials and reducing implant related infections via incorporation of silver and copper as antimicrobial elements: an overview. Mater Technol. 2022;37(9):867–879. doi: 10.1080/10667857.2022.2080347
- Huang K, Tang Z, Zhang L, et al. Preparation and characterization of mg-doped ZnO thin films by sol–gel method. Appl Surface Sci. 2012;258(8):3710–3713. doi: 10.1016/j.apsusc.2011.12.011
- Ding R, Xu C, Gu B, et al. Effects of mg incorporation on microstructure and optical properties of ZnO thin films prepared by sol-gel method. J Mater Sci Technol. 2010;26(7):601–604. doi: 10.1016/S1005-0302(10)60092-8
- Kim TH, Park JJ, Nam SH, et al. Fabrication of mg-doped ZnO thin films by laser ablation of Zn: Mg target. Applied Surface Science. 2009;255(10):5264–5266. doi: 10.1016/j.apsusc.2008.07.105
- Fang D, Li C, Wang N, et al. Structural and optical properties of mg-doped ZnO thin films prepared by a modified pechini method. Cryst Res Technol. 2013;48(5):265–272. doi: 10.1002/crat.201200437
- Bae J-S, Won M-S, Yoon J-H, et al. Preparation and characterization of Ce-doped ZnO nanofibers by an electrospinning method. JAST. 2011;2(1):1–6. doi: 10.5355/JAST.2011.1
- Fatima A, Devadason S, Pure J. Growth and haracterization of cerium oped zinc oxide nanocrystalline thin film. Appl & Ind Phy. 2011;2:115–120.
- Fujihara S, Ogawa Y, Kasai A. Tunable visible photoluminescence from ZnO thin films through mg-doping and annealing. Chem Mater. 2004;16(15):2965–2968. doi: 10.1021/cm049599i
- Dhara S, Giri PK. ZnO/anthracene based inorganic/organic nanowire heterostructure: photoresponse and photoluminescence studies. J Appl Phys. 2012;111(4):044320. doi: 10.1063/1.3687936
- Ta Y, Takahashi Y, Kanamori M, et al. Photoconductivity of Ultrathin Zinc Oxide Films. Jpn J Appl Phys Part. 1994;1(33):6611–6615. doi: 10.1143/JJAP.33.6611
- Hassan NK, Hashim MR, Allam NK. Low power UV photodetection characteristics of cross-linked ZnO nanorods/nanotetrapods grown on silicon chip. Sens Actuators A. 2013;192:124–129. doi: 10.1016/j.sna.2012.12.040
- Pandey N, Srivastava RK, Prakash SG. Study of dark conductivity and Photoconductivity in dysprosium doped zinc oxide synthesized by heat treatment method. Natl Acad Sci Lett. 2013;36(5):521–526. doi: 10.1007/s40009-013-0164-9
- Mackenzie JD, Ulrich DR. Sol-gel optics, present status and future trends. Proc Of SPIE Conf On Sol-Gel Optics. 1999;1328:2–13.