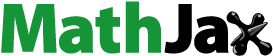
ABSTRACT
Keratoprosthesis (K-Pro) implantation and postoperative recovery often lead to severe ocular infections called keratoprosthesis-associated infections (KPAI). Antibiotics are commonly used; however, prolonged antibiotic use can lead to bacterial resistance and disrupt microbial balance in the eye. Borneol provides possibilities for developing antibiotic-independent antimicrobial K-Pro. Therefore, the development of an antibiotic-independent self-antimicrobial K-Pro is crucial for postoperative management. Herein, electrospinning technology was employed to fabricate a soluble borneol-based antibacterial coating on K-Pro surfaces for postoperative management of keratoprosthesis implantation. The modified K-Pro exhibited a distinctive nanofibrous morphology (100% borneol release ratio within 125 min), surface antibacterial properties (Escherichia coli: 2%, Staphylococcus aureus: 6%), biocompatibility, and negligible cytotoxicity towards epithelial cells. This study aimed to explore the potential application of camphor-based electrospun coatings in the field of K-Pro infection resistance, thereby providing novel strategies and material foundations for the effective postoperative management of KPAI.
Introduction
A keratoprosthesis (K-Pro), a titanium-based medical device, is used as a substitute for damaged or missing natural corneas [Citation1]. However, the implantation or postoperative recovery process of K-Pro often gives rise to severe ocular infections, known as keratoprosthesis-associated infections (KPAI) [Citation2]. Previous studies have reported an incidence rate of 8–17% for infectious keratitis following K-Pro implantation, with an annual follow-up rate of 0.034–0.073 per eye [Citation3]. Corneas with KPAI originate from tiny gaps between the implant and surrounding tissues, which pose significant risks to the exposed organ, the eye [Citation4]. Bacteria exploit these gaps to invade the eyeball, leading to severe corneal infections. Therefore, patients with K-Pro frequently require long-term use of antibiotic eye drops [Citation5] and prolonged antibiotic use can result in bacterial resistance, rendering previously susceptible bacteria difficult to treat [Citation6]. Moreover, antibiotics can disrupt normal microbial balance in the eyes, further augmenting the likelihood of infection. Consequently, the development of an antibiotic-independent self-antimicrobial, K-Pro, is crucial for postoperative management.
The surface antimicrobial modification technique offers a promising strategy for developing antibiotic-independent K-Pro [Citation7]. This technique primarily involves the surface modification of materials using chemical substances (e.g. metal ions/nanoparticles [Citation8], antibiotics [Citation9], photosensitisers, natural antimicrobial molecules, antimicrobial polymers [Citation10]). Among these, natural antimicrobial molecules (e.g. borneol [Citation11], curcumin [Citation7], tannic acid) are widely recognised as low-toxicity antimicrobial agents, possesses excellent antimicrobial properties and modifiability. Therefore, they were widely applied in the treatment of infections caused by bacteria, which provides possibilities for developing antibiotic-independent antimicrobial K-Pro. For instance, Piskin et. al. reported a poly(ε-caprolactone) fibre mat loaded with Calophyllum inophyllum (C. inophyllum) oil, achieving approximately 50% bactericidal efficiency on the material surface [Citation12]. Moreover, Razali et. al. developed an antimicrobial material loaded with curcumin, which prolonged the antimicrobial duration of natural antimicrobial small molecules, enabling their application in the treatment of infected wounds [Citation7]. Borneol, a well-known natural antibiotic found in medicinal plants, such as lavender, alfalfa, and sweet wormwood, exhibits remarkable antibacterial and anti-inflammatory properties and has been used in the development of various eye drops [Citation13,Citation14]. Borneol-based polymers exhibit excellent antibacterial adhesion and biocompatibility [Citation15]. For example, Yu et. al. reported that borneol-modified chitosan possesses excellent antibacterial activity against Escherichia coli and Bacillus Subtilis [Citation16]. However, the antibacterial efficacy of borneol is limited due to its extremely low water solubility [Citation17]. This limited solubility severely restricts the interaction between antibacterial drugs and bacterial cells, leading to treatment failure and emergence of bacterial resistance. Therefore, the development of borneol-based soluble antibacterial coatings for K-Pro surfaces is of paramount importance.
Electrospinning technology represents a method that enables rapid production of nanofibers directly from drug-containing polymer solutions [Citation18]. This technique facilitates the formation of coatings composed of nanofibers on diverse substrates [Citation19]. These coatings possess a substantial surface area and porosity, thereby enabling the loading of antibacterial drugs through electrostatic interactions, chemical bonding, and simple doping as well as the controlled release of antibacterial drugs in specific environments (e.g. water, pH, and temperature) [Citation20]. Consequently, these soluble electrospun antibacterial coatings can be effectively employed for the surface modification of K-Pro, thereby significantly enhancing its infection resistance and facilitating the postoperative management of KPAI. However, relevant studies in this field are lacking.
In this study, we employed electrospinning technology to fabricate a soluble borneol-based antibacterial coating on the surface of K-Pro to address the challenges associated with postoperative management of KPAI (). The modified K-Pro exhibited a distinctive nanofibrous morphology, excellent drug release performance (100% borneol release ratio within 125 min), surface antibacterial properties (E. coli: 4%, S. aureus: 6%), biocompatibility, and negligible cytotoxicity towards epithelial cells. This study aimed to explore the potential application of camphor-based electrospun coatings in the field of infection resistance for K-Pro, thereby providing novel strategies and material foundations for effective postoperative management of KPAI.
Experimental section
Materials and instrumentation
Polyvinylpyrrolidone (PVP, 500 g), L-borneol (99%), Cell Counting Kit-8 (CCK-8), 3‘,6’-dihydroxy-5-isothiocyanato-3 H-spiro[isobenzofuran-1,9’−xanthen]-3-one (FITC, 99%), and phosphate buffer solution (PBS, pH 7.4) were purchased from Aladdin Chemical Co., Ltd. Escherichia coli (E. coli, ATCC 43,888) and Staphylococcus aureus (S. aureus, ATCC BAA-1721) were purchased from the China Center of Industrial Culture Collection.
Preparation of borneol/PVP precursor
The PVP powder (1.0 g of PVP powder was dissolved in anhydrous ethanol (10 mL). The system was placed on a constant-temperature magnetic stirrer and stirred thoroughly until PVP was completely dissolved, resulting in a PVP solution.
0.1 g of borneol and 0.9 g of PVP powder were dissolved in 10 mL of ethanol, and then subjected to ultrasonic treatment using an ultrasonic cleaner for 30 min to obtain the PVP-B10 precursor solution.
0.2 g of borneol and 0.8 g of PVP powder were dissolved in 10 mL of ethanol and subjected to ultrasonic treatment using an ultrasonic cleaner for 30 min to obtain the PVP-B20 precursor solution.
Electrospun of borneol/PVP antibacterial coating on K-Pro
The prepared precursor solution was drawn into a 10 mL medical syringe equipped with a 19-gauge stainless-steel needle and connected to a negative high-voltage DC power supply [Citation21]. As the material of K-Pro is titanium (Ti), a titanium plate was chosen as the substrate for electrospinning. The Ti plate was connected to a positive high-voltage DC power supply and placed 17 cm from the stainless-steel needle. The needle was connected to a negative voltage of 2.0 kV, whereas the Ti plate was connected to a positive voltage of 20 kV. The precursor solution in the syringe was pushed out through the needle at a rate of 1.5 mL/h using a microinjection pump, stretched into fibres and solidified in a high-voltage electrostatic field, forming a borneol/PVP antibacterial coating on the Ti.
Characterisation of electrospun borneol/PVP antibacterial coating
The SEM and FT-IR spectra of K-Pro, K-Pro@PVP, K-Pro@PVP-B10, and K-Pro@PVP-B20 were characterised by a field emission scanning electron microscope (SEM, SU-70, Hitachi Nake high-tech enterprise, Japan) and a Nicolet 6700 FT-IR spectrometer (Thermo Scientific) in the 4000–600 cm−1 range [Citation22].
The wetting properties of K-Pro, K-Pro@PVP, K-Pro@PVP-B10, and K-Pro@PVP-B20 were characterised using a DSA100 dynamic contact angle measurement instrument [Citation23]. After drying the samples, they were placed on the test stage, and a water droplet with a volume of 2 μL was dispensed onto the surface. Once the water droplets reached complete stability, their contact angles were measured. Five different points were measured for each sample to obtain the average value.
Borneol releasing ability of electrospun borneol/PVP antibacterial coating
K-Pro, K-Pro@PVP, K-Pro@PVP-B10, and K-Pro@PVP-B20 (1 × 2 cm2) were placed in a PBS buffer (pH 7.4). At specific time intervals, the buffer solution was sampled to measure its ultraviolet (UV) absorption intensity at 267 nm using a UV-VIS spectrophotometer (UV-2550, Shimadzu, China). The release rate of the material was calculated based on the UV absorption intensity [Citation24].
Biocompatibility of electrospun borneol/PVP antibacterial coating
In each well of a 24-well plate, 2 × 104Corneal epithelial cells were seeded and incubated in a CO2 incubator for 24 h to allow cell adhesion. After disinfecting the samples (K-Pro, K-Pro@PVP, K-Pro@PVP-B10, and K-Pro@PVP-B20) with alcohol, they were placed in 24-well plates containing the cells. The cells were then further incubated for 24 hours [Citation25]. Subsequently, 10% CCK-8 solution was added, and the cells were incubated in the dark for 30 min. Cells were photographed using an inverted microscope. After that, 100 μL of the solution was taken to measure the absorbance at 450 nm. The formula for calculating cell viability (%) is as follows:
where, ODa, ODc, and ODb represent the absorbances of the test sample, positive control group, and negative control group, respectively.
In vitro antibacterial activity of electrospun borneol/PVP antibacterial coating
E. coli (ATCC 43,888) and S. aureus (ATCC BAA-1721) were used to evaluate the antibacterial effects of all samples (K-Pro, K-Pro@PVP, K-Pro@PVP-B10, and K-Pro@PVP-B20). First, 0.1 mL a bacterial suspension (1X106 CFU/mL) was dropped onto the surface of the test samples for co-cultivation. After 24 h, the bacterial suspension (200 μL) was inoculated onto agar medium for bacterial colony counting to determine bacterial viability [Citation26]:
where Na and Nb represent the bacterial colony counts of K-Pro (control group) and K-Pro@PVP, K-Pro@PVP-B10, and K-Pro@PVP-B20 (experimental group), respectively.
Next, the bacteria were labelled with FITC and 0.1 mL of the bacterial suspension (1X106 CFU/mL) was dropped onto the surface of the test samples for co-cultivation. After 24 h, the samples were rinsed with phosphate buffered saline (PBS) and observed under an inverted fluorescence microscope. The antibacterial adhesion ratio of the sample surface to the bacteria was determined by analysing the distribution of the fluorescence intensity:
where Fa and Fb represent the fluorescence intensities of K-Pro (control group) and K-Pro@PVP, K-Pro@PVP-B10, and K-Pro@PVP-B20 (experimental group), respectively.
Statistical analysis
Data are presented as mean ± standard deviation (S.D.). For in vitro experiments, a minimum of three independent experiments (sample size, n = 3) were conducted. Statistical analyses were performed using Origin 2021 and GraphPad Prism 7.00.
Result and discussion
Preparation and characterisation of electrospun borneol/PVP antibacterial coating
The borneol/PVP coating developed using electrospinning technology exhibited a typical nanofiber structure. As shown in , the surface of the unmodified K-Pro was clean and regular. However, after the introduction of the electrospinning technology, the surface of K-Pro contained many nanofibers (K-Pro@PVP). Furthermore, when borneol was added to the electrospinning system, the diameter of the nanofibers increased (). As the borneol content increased from 0% to 20%, the diameter of the nanofibers on the K-Pro surface increased from 320 to 470 nm. These results indicate that electrospinning can successfully modify the surface of K-Pro using a borneol/PVP coating. Moreover, the increase in the nanofiber diameter of the borneol/PVP coating was attributed to the incorporation of borneol, which increased the spatial hindrance between PVP molecules in the entire system.
Figure 1. Characterisation of electrospun borneol/PVP antibacterial coating. (a) SEM photo of K-Pro, K-Pro@PVP, K-Pro@PVP-B10, and K-Pro@PVP-B20; the diameter of the nanofiber on the surface of (b) K-Pro@PVP, (c)K-Pro@PVP-B10, and (d) K-Pro@PVP-B20.

The structure of the borneol/PVP coating was further confirmed using IR spectroscopy (). In the borneol/PVP coating (PVP-B10 and PVP-B20), characteristic peaks belonging to both PVP and borneol were observed. The characteristic peak of PVP appeared at 1610 cm−1 (C=O), whereas the characteristic peaks of borneol were concentrated in the range of 1000–1100 cm−1 (C-C). This demonstrates that the precursor solution based on borneol/PVP can form a well-defined coating on the surface of K-Pro using electrospinning technology. This helped improve the surface properties of K-Pro.
Hydrophilic characterisation of electrospun borneol/PVP antibacterial coating
Hydrophilicity is crucial in the development of ocular implants. The surface hydrophilicity of K-Pro modified with the borneol/PVP coating was characterised using water contact angle experiments. As shown in , unmodified K-Pro exhibited hydrophilicity with a water contact angle of 56°. When the surface was covered with PVP nanofibers, the surface hydrophilicity of K-Pro increased further, with a water contact angle of 44°. However, when borneol was introduced into the electrospinning system, the surface hydrophilicity of K-Pro@PVP-B10 decreased and the water contact angle increased to 58°. This effect became more pronounced when the borneol content increased to 20%, resulting in a further decrease in the surface hydrophilicity of K-Pro@PVP-B20 as the water contact angle increased to 67°.
Figure 3. Hydrophilic characterisation of electrospun borneol/PVP antibacterial coating. (a) Digital photographs and (b) numerical analysis of water contact angles of K-Pro, K-Pro@PVP, K-Pro@PVP-B10, and K-Pro@PVP-B20. (c) Borneol releasing kinetics of Borneol, PVP-B10, and PVP-B20.

These results can be attributed to the different hydrophilic properties of PVP and borneol. PVP exhibits excellent water solubility and further enhances the hydrophilicity of the substrate surface after the formation of a nanofiber coating. However, borneol has extremely poor water solubility. As the borneol content on the substrate surface gradually increased, the surface energy of the substrate increased, and its hydrophilicity decreased. However, although the borneol/PVP coating increased the hydrophobicity of K-Pro, it remained within the hydrophilic range (0–90), which has positive implications for ocular implants.
Borneol releasing kinetics of electrospun borneol/PVP antibacterial coating
The water solubility of borneol limits its potential as an antibacterial agent to protect the surface of K-Pro. To address this issue, we designed a borneol/polyvinylpyrrolidone electrospun coating to improve the release of borneol from the surface of K-Pro. As shown in , borneol has low solubility in water, with a dissolution ratio of only 30% within 300 min. However, when borneol is mixed with PVP to form an electrospun coating, it is rapidly released into the environment (100% within 125 min). This was attributed to the good hydrophilicity of PVP. Borneol molecules were embedded between the PVP molecules in the system. With PVP acting as a co-solvent, good dissolution from the surface of K-Pro was achieved. The release rate was inhibited as borneol content increased. The PVP-B10 coating achieved 100% release of borneol within 75 min, whereas PVP-B20 required 125 min to achieve 100% release. This was due to the decreased water solubility caused by the increase in borneol content.
Biocompatibility of electrospun borneol/PVP antibacterial coating
Biocompatibility was the primary consideration for all implants. We characterised the biocompatibility of borneol/PVP coatings using corneal epithelial cells. As shown in , K-Pro exhibited excellent biocompatibility and extremely low cytotoxicity, and the corneal epithelial cells maintained good density and morphology after co-culturing with K-Pro for 24 h. When PVP nanofibers were modified on the surface of K-Pro, the proliferation and morphology of the corneal epithelial cells were not affected. Similar results are obtained for K-Pro@PVP-B10 and K-Pro@PVP-B20. As shown in , the viability of corneal epithelial cells remained above 90% after co-culturing with K-Pro, K-Pro@PVP, K-Pro@PVP-B10, and K-Pro@PVP-B20 for 24 h. These results indicate that the components involved in the borneol/PVP coating exhibited good biocompatibility and maintained extremely low cytotoxicity, even after being modified on the surface of K-Pro.
In vitro antibacterial ability of electrospun borneol/PVP antibacterial coating
Antibacterial activity is important for the postoperative management of keratoprosthesis implantation. Therefore, we characterised the antibacterial performance of the borneol/PVP coating in vitro. As shown in , E. coli thrived on the surfaces of K-Pro and K-Pro@PVP, resulting in high-density bacterial colonies when the bacterial suspension was inoculated into the solid culture medium. This situation changed significantly with borneol intervention. On the surface of K-Pro@PVP-B10, only a few bacterial colonies formed after settling on the solid culture medium, and the number of E. coli further decreased when the borneol content increased to 20%. Similar results were obtained for S. aureus. These results indicate that the borneol/PVP coating can significantly eliminate bacteria on the surface of K-Pro, and that the antibacterial effect increases with increasing borneol content.
Figure 5. In vitro antibacterial ability of electrospun borneol/PVP antibacterial coating. (a) Images of bacterial clones and (b) CLSM diagrams of K-Pro, K-Pro@PVP, K-Pro@PVP-B10, and K-Pro@PVP-B20 co-cultured with E. coli and S. aureus for 24 h.

Furthermore, the antibacterial adhesion ability of the electrospun borneol/PVP coatings was investigated. As shown in , the bacteria were labelled with the fluorescent dye FITC, which emitted green fluorescence under excitation light (456 nm). After co-culture, the K-Pro and K-Pro@PVP surfaces exhibited a significant area and intensity of green fluorescence, indicating that they were heavily covered with E. coli. When the borneol/PVP coating was applied to the surface of K-Pro, the fluorescence area and intensity of the surfaces of K-Pro@PVP-B10 and K-Pro@PVP-B20 decreased significantly. This suggests that the incorporation of borneol enhances the bactericidal ability of the coating against E. coli, thereby reducing the adhesion of E. coli to the surface of K-Pro.
The efficient bactericidal ability was further quantified. As shown in , the survival rates of E. coli in the K-Pro, K-Pro@PVP, K-Pro@PVP-B10, and K-Pro@PVP-B20 cells were 100%, 113%, 8%, and 2%, respectively. Similarly, the survival rates of S. aureus in the K-Pro, K-Pro@PVP, K-Pro@PVP-B10, and K-Pro@PVP-B20 groups were 100, 100, 4, and 6%, respectively. When the bacteria were labelled with FITC, the adhesion rates of E. coli on the surfaces of K-Pro, K-Pro@PVP, K-Pro@PVP-B10, and K-Pro@PVP-B20 were 100%, 75%, 42%, and 15%, respectively. Similarly, the adhesion rates of S. aureus on the K-Pro, K-Pro@PVP, K-Pro@PVP-B10, and K-Pro@PVP-B20 surfaces were 100%, 125%, 22%, and 8%, respectively. These results indicate that the borneol/PVP electrospun coating could significantly kill bacteria and reduce their adhesion to the K-Pro surface.
Conclusions
In this study, electrospinning technology was employed to fabricate a soluble borneol-based antibacterial coating on K-Pro surfaces for postoperative management of keratoprosthesis implantation. In clinical scenarios, this strategy aims to address the bacterial infections that occur when a keratoprosthesis is implanted into the human eye. The components of the coatings are biocompatible. K-Pro@PVP-B20 exhibited nanofibrous morphology, good hydrophilicity (water contact angle of 67°), and could release antibacterial substances (100% borneol release ratio within 125 min) and surface antibacterial properties (E. coli: 2%, S. aureus: 6%), reducing the pain caused by frequent eye drops in patients. However, further research is required to determine whether this strategy maintains good antibacterial properties and biocompatibility in vivo.
Author contributions
Y.L. and T.W. contributed equally to this work. Conceptualisation: X.B., T.W., and Y.L.; Methodology: X.B., T.W., and Y. L.; Investigation: K.Y. and X.B.; Resources: Y.L.; Data Calculation: X.B.; Writing – Original Draft Preparation: X.B.; Writing – Review and Editing: X.B., T.W., and Y.L.; Visualisation: X.B.; Supervision: X.B.; Project Administration: X.B.; Funding Acquisition: T.L. All authors have read and agreed to the published version of the manuscript.
Disclosure statement
No potential conflict of interest was reported by the authors.
Additional information
Funding
References
- Chen M, Ng SM, Akpek EK, et al. Artificial corneas versus donor corneas for repeat corneal transplants. Cochrane Database Syst Rev. 2020;2020(5):20. doi: 10.1002/14651858.CD009561.pub3
- Khan B, Dudenhoefer EJ, Dohlman CH. Keratoprosthesis: an update. Curr Opin Ophthalmol. 2001;12(4):282–9. doi: 10.1097/00055735-200108000-00007
- Khan BF, Harissi-Dagher M, Khan DM, et al. Advances in Boston keratoprosthesis: enhancing retention and prevention of infection and inflammation. Int Ophthalmol Clin. 2007;47(2):61–71. doi: 10.1097/IIO.0b013e318036bd8b
- Prabhasawat P, Chotikavanich S, Ngowyutagon P, et al. Long-term outcomes of Boston type I keratoprosthesis, and efficacy of amphotericin B and povidone-iodine in infection prophylaxis. Am J Ophthalmol. 2021;232:40–48. doi: 10.1016/j.ajo.2021.05.022
- Dohlman C. The boston keratoprosthesis—the first 50 years: some reminiscences. Annu Rev Vis Sci. 2022;8(1):1–32. doi: 10.1146/annurev-vision-100820-021253
- Li W, Thian ES, Wang M, et al. Surface design for antibacterial materials: from fundamentals to advanced strategies. Adv Sci. 2021;8(19):2100368. doi: 10.1002/advs.202100368
- Jing W, Hussain SA, Reddy Gangireddygari VS, et al. Biocompatibility and antibacterial activity studies of gellan gum and alginate thin film encapsulating curcumin as a wound skin care dressing. Mater Technol. 2023;38(1):2282321. doi: 10.1080/10667857.2023.2282321
- Tharchanaa SB, Anupriyanka T, Shanmugavelayutham G. Ecofriendly surface modification of cotton fabric to enhance the adhesion of CuO nanoparticles for antibacterial activity. Mater Technol. 2022;37(14):3222–3230. doi: 10.1080/10667857.2022.2141169
- Pal S, Mondal S, Maity J. In situ generation and deposition of ZnO nanoparticles on cotton surface to impart hydrophobicity: investigation of antibacterial activity. Mater Technol. 2018;33(8):555–562. doi: 10.1080/10667857.2018.1483306
- Humayun A, Luo Y, Elumalai A, et al. 3D printed antimicrobial PLA constructs functionalised with zinc- coated halloysite nanotubes-ag-chitosan oligosaccharide lactate. Mater Technol. 2020;37(1):1–8. doi: 10.1080/10667857.2020.1806188
- Chen X, Chen Y, Lv S, et al. New type of borneol-based fluorine-free superhydrophobic antibacterial polymeric coating. Des Monomers Polym. 2021;24(1):147–157. doi: 10.1080/15685551.2021.1924959
- Ustundag CR, Piskin MB. Investigation of electrospun poly (ε-caprolactone) fiber mats loaded with calophyllum inophyllum essential oil for wound dressing applications: morphology, drug release and in vitro evaluation. Mater Technol. 2023;38(1):2223018. doi: 10.1080/10667857.2023.2223018
- Ma R, Lu D, Wang J, et al. Comparison of pharmacological activity and safety of different stereochemical configurations of borneol: L-borneol, D-borneol, and synthetic borneol. Biomed Pharmacother. 2023;164:114668. doi: 10.1016/j.biopha.2023.114668
- Kulkarni M, Sawant N, Kolapkar A, et al. Borneol: a promising monoterpenoid in enhancing drug delivery across various physiological barriers. AAPS Pharm Sci Tech. 2021;22(4):145. doi: 10.1208/s12249-021-01999-8
- Cheng Q, Asha AB, Liu Y, et al. Antifouling and antibacterial Polymer-Coated Surfaces Based on the combined effect of zwitterions and the natural borneol. ACS Appl Mater Inter. 2021;13(7):9006–9014. doi: 10.1021/acsami.0c22658
- Xin Y, Zhao H, Xu J, et al. Borneol-modified chitosan: Antimicrobial adhesion properties and application in skin flora protection. Carbohydr Polym. 2020;228:115378. doi: 10.1016/j.carbpol.2019.115378
- Wu JH, Wang C, Mu C, et al. A waterborne polyurethane coating functionalized by isobornyl with enhanced antibacterial adhesion and hydrophobic property. Eur Polym J. 2018;108:498–506. doi: 10.1016/j.eurpolymj.2018.09.034
- Lv CL, Li L, Jiao Z, et al. Improved hemostatic effects by Fe3+ modified biomimetic PLLA cotton-like mat via sodium alginate grafted with dopamine. Bioact Mater. 2021;6(8):2346–2359. doi: 10.1016/j.bioactmat.2021.01.002
- Kachwal V, Tan JC. Stimuli-responsive electrospun fluorescent fibers augmented with Aggregation-Induced Emission (AIE) for smart applications. Adv Sci. 2023;10(1):29. doi: 10.1002/advs.202204848
- Xi K, Gu Y, Tang J, et al. Microenvironment-responsive immunoregulatory electrospun fibers for promoting nerve function recovery. Nat Commun. 2020;11(1):18. doi: 10.1038/s41467-020-18265-3
- Song G, Cheong JY, Kim C, et al. Atomic-scale combination of germanium-zinc nanofibers for structural and electrochemical evolution. Nat Commun. 2019;10(1):11. doi: 10.1038/s41467-019-10305-x
- Zhang ZA, Xia Z, Huang J, et al. Uneven phosphoric acid interfaces with enhanced electrochemical performance for high-temperature polymer electrolyte fuel cells. Sci Adv. 2023;9(4):10. doi: 10.1126/sciadv.ade1194
- Yu QH, Yuan Y, Wen J, et al. A universally applicable strategy for construction of anti-biofouling adsorbents for enhanced uranium recovery from seawater. Adv Sci. 2019;6(13):10. doi: 10.1002/advs.201900002
- Li WL, Hua G, Cai J, et al. Multi-stimulus responsive multilayer coating for treatment of device-associated infections. J Func Biomater. 2022;13(1):17. doi: 10.3390/jfb13010024
- Li WL, Chen H, Cai J, et al. Poly(pentahydropyrimidine)-based hybrid hydrogel with synergistic antibacterial and pro-angiogenic ability for the therapy of diabetic foot ulcers. Adv Funct Mater. 2023;33(49):15. doi: 10.1002/adfm.202303147
- Li WL, Cai J, Zhou W, et al. Poly(aspartic acid)-based self-healing hydrogel with precise antibacterial ability for rapid infected-wound repairing. colloids and surfaces B-Biointerfaces. Colloids Surf B Biointerfaces. 2023;221:9. doi: 10.1016/j.colsurfb.2022.112982