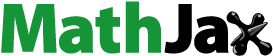
ABSTRACT
The present study aims to alloy the surface of ZK60 Mg alloy with Sn using friction stir processing (FSP). Experiments were conducted with the combination of higher and lower values of tool rotational speed and feed rate to evaluate the best combination of parameters for the alloy formation. The surface alloy has been successfully formed with improved hardness and corrosion properties in the combination of higher rotational speed of 2800 rpm and lower processing speed of 0.6 mm/min, which was attributed to the proper diffusion of Sn particles at the higher temperature produced in that combination of parameters.
Introduction
Mg and its alloys have been in focus over the past few decades as they find potential applications in automotive, aerospace, and biomedical industries due to their high strength to weight ratio. However, their practical applications are limited by their inherent challenges like poor corrosion resistance and machinability. Consequently, most of the research efforts have been directed towards enhancing the corrosion resistance by alloying with various elements like Zn, Ca, Sn etc. Poor machinability of Mg alloys can be attributed to its HCP (hexagonal close packed) structure which has only three slip systems, whereas a material ideally requires five slip systems for favourable deformation properties. To overcome this challenges, various approaches have been explored, including alloying with rare earth elements and employing severe plastic deformation processes like ECAP [Citation1,Citation2], high pressure torsion [Citation3], accumulative roll bonding [Citation4] and multi-axial forging [Citation5] etc. Notably, Misra and his group [Citation6–10] have made significant contributions in the field of multi-axial forging of Mg-RE alloys. They observed that this process is very effective in producing ultra fine grains which results in fine distribution of precipitates in the alloy leading to high strength and ductility combination. Another notable technique, friction stir processing (FSP) is also one of the severe plastic deformation techniques, which modifies the surface thereby providing the surface texture that improves the strength, ductility, hardness and corrosion resistance in some cases. Eventually, the researchers have been able to develop surface composites using FSP by incorporating various ceramic particles in-situ. The heat generated in the FSP processed zone is determined by the rotational and transverse speeds of the tool [Citation11,Citation12]. Sufficient heat generation in the processed zone is essential to ensure defect-free processing and the maximum temperatures typically fall within the range of 0.6–0.9 times of the melting temperature (Tm), as emphasized by Mishra and Ma [Citation13]. In the case of fabrication of surface composites, a higher rotational speed is necessary to effectively distribute and break up the clusters of reinforcement particles. However, it’s important to note that excessively high rotational speeds can negatively impact grain refinement due to increased heat input [Citation14]. Recently, there have been some studies focusing on the development of selective alloy on Al alloys [Citation15–17] and Cu alloys [Citation18] using FSP for improving the weldability, hardness and strength.
Most of the literature was focused on the development of surface composites using FSP by reinforcing ceramic particles and some have used metallic particles to prepare surface composites as understood from the above cited references. Very limited work has been done and systematic approach is lacking in developing the surface alloys in the solid state using FSP. The present work focuses on the solid-state surface alloying of ZK60 Mg alloy with Tin by systematically varying and studying the process parameters of FSP. The addition of Sn improves the corrosion resistance of Mg alloys by forming SnO2 during corrosion process [Citation19], refines the grain size and thereby improves the strength due to the formation of Mg2Sn [Citation20]. Furthermore, the high solid solubility limits of Sn in Mg lead to the improved creep and tensile properties by solution strengthening [Citation21].
Materials and methods
The friction stir process was performed on the ZK60 Mg alloy (5.5–6 wt.% Zn, 0.5–1 wt.% Zr, Mg balance) work specimen measuring 150 mm × 75 mm × 6 mm. A total of 41 holes were made with 2 mm diameter and 3 mm deep drilled in zig-zag manner on the specimen and then filled with Sn metal powder of size ~44 µm. FSP was carried out using the tool made of hardened H-13 tool steel consisting of conical pin with a diameter 4 mm over 4 mm length and with a shoulder diameter of 20 mm. During plunging, the axial force ranging between 3500 N and 5500 N was applied by rotating tool and the piercing rate of rotating tool was maintained as low as 0.050 mm/sec. The purpose of the slow piercing rate is to ensure proper heat generation and thereby plasticization of material. Tool rotational speed and feed rate were chosen as main variables for the effective formation of alloy in a single pass. Hence, maximum and minimum levels in both the parameters were taken and experiments were carried out in four different combinations as shown .
Table 1. Sample codes and their FSP parameters.
The microstructure of the processed zone was observed by polishing the specimen to the mirror finish as per ASTM E3–11 standard. Polished specimens were then cleaned with ethanol and etched with picral (0.4 g of picric acid, 10 ml of ethanol, 2 ml acetic acid and 5 ml distilled water). Optical micrographs were obtained using an optical microscope (Olympus, model BX 51 M). Phase analysis was carried out by scanning the samples using X-ray diffractometer with Cu Kα radiation and the scanning speed of 1°/min and a 0.05° step size. Scanning Electron Microscopy (SEM) with Energy Dispersive X-Ray Analysis (EDX) was carried out to confirm the presence of Sn in the FSPed zones. Microhardness in the processed area of the sample was measured using Vickers-microhardness machine (Schimadzu) with a 100gf load and 15s dwell time.
The electrochemical analysis was performed using a potentiostat (Gamry Instruments,Warminster, PA, U.S.A., model: Interface 1010T) with three electrode system consisting of Saturated Calomel Electrode (SCE) and a platinum wire, as reference and counter electrodes respectively. The test was conducted by exposing the surface area of 0.375 cm2 of the sample at the room temperature in 3.5 wt.⁒ NaCl solution. The potentiodynamic polarization (PDP) curves were obtained by polarizing the sample at the scan rate of 0.5 mV/s from an initial and final potentials of −0.3 V and +1.2 V respectively with reference to the open circuit potential (OCP) after an initial delay of 60 min. The PDP curves were analysed by employing the Tafel fitting of the curves using Gamry Echem Analyst software and electrochemical parameters such as icorr, Ecorr and corrosion rate.
Results and discussion
Microstructure analysis
shows the optical micrographs of the FSPed samples, and the base metal and their corresponding average grain size values are listed in . It can be clearly understood that the grain size of all the friction stir processed samples have significantly reduced compared to that of base metal. FSP being the severe plastic deformation process coupled with high temperature in the processing zone, dynamic recrystallization (DRX) occurs and newer, finer and equiaxed grain structure results. Such a microstructure would impart greater strength and hardness. In the present study, the lowest grainsize was found to be for the sample DS3 followed by DS1. Generally, with the increase in tool rotational speed the grain size increases during DRX due to the higher heat input. Conversely, in the case of DS3, grain size has largely reduced rather than increasing which could be due to the addition of tin and the alloy formation which in turn hindered the grain growth. In the case of sample DS1, both the higher feed rate and lower rotational speed of the tool along with the formation of alloy would have contributed to the lower grain size. It is further evident from the grain size of 6 μm in the sample DS4, which is higher among the friction sir processed samples, that the higher rotational speed of the tool has led to the grain growth.
Figure 1. Optical micrographs of the base metal (a) and surface alloyed samples (b) DS1, (c) DS2, (d) DS3, and (e) DS4 showing the grain reduction and refinement.

Table 2. Average grain size of base metal and FSPed samples.
Phase analysis
shows the XRD patterns of friction stir processed ZK60 Mg alloy with different combinations of rotational speed and feed in comparison with the base metal. It can be observed that the samples DS1 and DS3 contains the peaks corresponding to Mg2Sn phase at 2θ = 22.7º which matched with the standard reference pattern of Mg2Sn (JCPDS #:00-007-0274). The formation of Mg2Sn is a clear indication of the formation of Mg-Sn alloy on the surface. Further, it is evident that the intensity of this peak in DS3 is higher compared to that of sample DS1 which indicates that the higher rpm with lower feed rates aids the formation of alloy. This can be attributed to the temperature generated due to the higher rotational speed in conjunction with the longer stirring time at the same location. Furthermore, XRD patterns of samples DS1 and DS3 reveals no individual peaks of Sn confirming the formation of alloy, whereas the patterns of samples DS2 and DS4 show the peaks corresponding to Sn indicating unsuccessful formation of alloy and the Sn powder remained as it is. presents the temperature generated at the interface of tool and workpiece which was measured using laser gun during the process. It can be clearly seen that the higher temperature was generated in case of DS3, possibly by the higher rotational speed and lower feed rate of the tool. Diffusion of Sn in Mg (DSn in Mg) at different temperatures was also calculated using Arrhenius equation given in Eq. 1 [Citation22] and presented in .
Where,
D= Diffusion coefficient
Do = Temperature-independent constant (m2/s)
Qd= Activation energy for diffusion (J/mol)
R=Gas constant (8.31 J/mol.K)
T = Absolute temperature (K)
The values of Do and Qd were taken from the reference [Citation21] as 1.08 m2/s and 140,900 J/mol, respectively, and diffusion coefficients were calculated. It can be observed that the descending order of solid-state diffusion coefficients was found to be in the order of DS3>DS1>DS4>DS2. Hence, it can be understood that the higher diffusion of Sn in Mg at higher temperature produced in the case of the samples DS3 and DS1 could be the reason for the formation of surface alloy. The EDX spectrum of the sample DS3 is presented in further confirms the presence of Sn and thereby the formation of surface alloy.
Microhardness
depicts the microhardness of the samples in this study. The samples DS3 and DS1 show higher hardness than that of DS2 and DS4 which can be attributed to the solid solution strengthening effect. However, it is worth noting that, among the DS3 and DS1, the sample DS3 has shown higher hardness, indicating that the higher rpm and lower processing speed aids in the formation of alloy and thereby increasing the hardness.
Corrosion studies
shows the potentiodynamic polarization curves of all the samples in this study and their electrochemical parameters from the Tafel curve fitting are listed in . Evidently all the FSPed samples showed lower corrosion rates with corrosion current densities reduced to at least 2–3 orders compared to the base metal. Out of all the samples, the sample DS3 showed the least corrosion rates which could be attributed to the surface alloy formation. It was already established that the Sn helps in improving the corrosion resistance in Mg alloys. Hence, in the present study also, it was proved that the Sn has contributed to the increase in corrosion resistance in case of the samples DS1 and DS3 in which the Sn has formed an alloy with ZK60 Mg alloy on its surface. A little observation shows that the samples processed with higher rpm (DS3 and DS4) have shown quick passivation behaviour as can be observed from their PDP curves. It can also be noted that the higher feed rates are not favourable for the alloy formation and thereby corrosion resistance.
Table 3. Electrochemical parameters of surface alloyed samples and the base metal.
Conclusion
The present research reports the successful development of surface alloy by systematically varying the tool rotational speed and the feed rate. The Mg2Sn phase was formed in the samples processed with lower feed rates of 0.6 mm/min. The higher tool rotational speed of 2800 rpm in combination with lower feed rate of 0.6 mm/min was proved to be the best combination for the successful surface alloying. The hardness and corrosion resistance also have improved significantly in the samples in which the alloy formation has occurred indicating the solid solution strengthening and alloy formation respectively. The combination of tool rotational speed of 2800 rpm and processing speed of 0.6 mm/min yields the higher hardness (92.46 HV0.1), corrosion resistance (6.09 × 10−6 A/cm2) and lower grain size (2.9 µm). Hence, it can be concluded that the higher tool rotational speed provides the higher heat input and lower feed rates provide sufficient time for proper distribution and diffusion of alloying elements, thereby leading to the formation of surface alloy. Authors also feel that the same parameters may not be applicable in case of other alloying elements and systematic studies have to be conducted to arrive at the optimized parameters for the surface alloy formation with every alloying element.
Disclosure statement
No potential conflict of interest was reported by the author(s).
References
- Azzeddine H, Sadi S, Hanna A, et al. Grain growth activation energy of ECAP-processed Mg-ce alloy: effect of deformation temperature. Mater Today Commun. 2023;37:107161. doi: 10.1016/j.mtcomm.2023.107161
- Sun Z, Li Y, Ma M, et al. Study on the effect of the ECAP deformation on the organization and properties of the extruded Mg-sn-al alloys. Mater Lett. 2024;357:135775. doi: 10.1016/j.matlet.2023.135775
- Xue K, Luo Z, Xia S, et al. Study of microstructural evolution, mechanical properties and plastic deformation behavior of Mg-gd-Y-Zn-zr alloy prepared by high-pressure torsion. Mater Sci Eng A. 2024;891:145953. doi: 10.1016/j.msea.2023.145953
- Sun L, Li F, Zhang AX, et al. Mater Sci Eng A. 2024;892. doi: 10.1016/j.msea.2024.146105
- Misra RDK. Strong and ductile texture-free ultrafine-grained magnesium alloy via three-axial forging. Mater Lett. 2023;331:133443. doi: 10.1016/j.matlet.2022.133443
- Misra RDK. Mater Technol. 2023;38. doi: 10.1080/10667857.2023.2189769
- Misra RDK. Mater Technol. 2023;38. doi: 10.1080/10667857.2023.2278000
- Weaver MR, Maldonado AJ, Banuelos JL, et al. On precipitation hardening behaviour in a triaxial forged Mg-2Zn-2Gd alloy and relationship to mechanical properties. Mater Technol. 2023;38(1). doi: 10.1080/10667857.2023.2215038
- Li K, Injeti VSY, Trivedi P, et al. Nanoscale deformation of multiaxially forged ultrafine-grained mg-2Zn-2Gd alloy with high strength-high ductility combination and comparison with the coarse-grained counterpart. J Mater Sci Technol. 2018;34(2):311. doi: 10.1016/j.jmst.2017.07.023
- Trivedi P, Nune KC, Misra RDK, et al. Grain refinement to submicron regime in multiaxial forged Mg-2Zn-2Gd alloy and relationship to mechanical properties. Mater Sci Eng A. 2016;668:59. doi: 10.1016/j.msea.2016.05.050
- Dolatkhah A, Golbabaei P, Besharati Givi MK, et al. Investigating effects of process parameters on microstructural and mechanical properties of Al5052/SiC metal matrix composite fabricated via friction stir processing. Mater Des. 2012;37:458. doi: 10.1016/j.matdes.2011.09.035
- Khayyamin D, Mostafapour A, Keshmiri R. The effect of process parameters on microstructural characteristics of AZ91/SiO2 composite fabricated by FSP. Mater Sci Eng A. 2013;559:217. doi: 10.1016/j.msea.2012.08.084
- Mishra RS, Ma ZY. Friction stir welding and processing. Mater Sci Eng R Rep. 2005;50(1–2):1–6. doi: 10.1016/j.mser.2005.07.001
- Azizieh M, Kokabi AH, Abachi P. Effect of rotational speed and probe profile on microstructure and hardness of AZ31/Al2O3 nanocomposites fabricated by friction stir processing. Mater Des. 2011;32(4):2034. doi: 10.1016/j.matdes.2010.11.055
- Karthik GM, Ram GDJ, Kottada RS. Friction stir selective alloying. Mater Sci Eng A. 2017;684:186. doi: 10.1016/j.msea.2016.12.064
- Selvakumar S, Dinaharan I, Palanivel R, et al. Characterization of molybdenum particles reinforced Al6082 aluminum matrix composites with improved ductility produced using friction stir processing. Mater Charact. 2017;125(13):13–22. doi: 10.1016/j.matchar.2017.01.016
- Asrari G, Daneshifar MH, Hosseini SA, et al. Selective alloying of pure aluminum with varying amounts of magnesium using friction stir processing for improved mechanical and corrosion-resistant properties. Mater Chem Phys. 2023;306:128091. doi: 10.1016/j.matchemphys.2023.128091
- Moiduddin K, Siddiquee AN, Abidi MH, et al. Crystals (Basel). Crystals. 2021;11(5):498. doi: 10.3390/cryst11050498
- Jiang W, Yu W. Crystals (Basel). Crystals. 2022;12(9):1219. doi: 10.3390/cryst12091219
- Rajendran R, Dondapati S. Insights of microstructural features and their effect on degradation and the in vitro bioactivity response of as-cast mg-sn alloys for orthopedic implant applications. Materials. 2022;15(18):6327. doi: 10.3390/ma15186327
- Radha R, Sreekanth D. Mechanical, in vitro corrosion and bioactivity performance of Mg based composite for orthopedic implant applications: influence of Sn and HA addition. Biomed Eng Adv. 2022;3:100033. doi: 10.1016/j.bea.2022.100033
- Zhong W, Zhao JC. First reliable diffusion coefficients for mg-Y and additional reliable diffusion coefficients for mg-sn and mg-zn. Metall Mater Trans A Phys Metall Mater Sci. 2017;48(12):5778. doi: 10.1007/s11661-017-4378-1