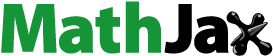
ABSTRACT
Texture is an important aspect that impacts the formability of magnesium (Mg) and its alloys. Recently, the unique characteristics of multiaxial forging of Mg-2Zn-2Gd alloy were discussed, where it was demonstrated that multiaxial forged processed alloy is characterized by high strength and high ductility combination and the texture is significantly weakened or almost texture-free. The objective of the study described here is to obtain some fundamental insights that explain the underlying reasons for the weakening of texture in the multiaxial forged Mg-2Zn-2Gd alloy.
Introduction
Grain refinement and introducing an ultrafine grain size through a severe plastic deformation process in metals and alloys are considered a viable approach to significantly enhance the mechanical properties beyond what can be achieved by conventional processing methods. The underlying reason is that the severe plastic deformation methods enable stable deformation to be introduced at large strains.
In recent years, severe plastic deformation processing has attracted significant attention to processing bulk ultrafine-grained (UFG) metals and alloys. While there are several severe plastic deformation methods, multiaxial or multidirectional forging is viewed as a plastic deformation process that is generally believed to involve dynamic recrystallization and dynamic precipitation. UFG alloys are envisaged to exhibit outstanding cyclic stability together with a good combination of strength and ductility [Citation1–5]. High strength and high ductility combination governs the formability of structural metals and alloys [Citation6–16].
Magnesium (Mg) with density lower than steel (<78%) and aluminium (<37%) is attractive for use in automotive and aerospace applications [Citation4,Citation5]. However, the hexagonal close-packed structure of Mg with limited number of slip systems makes it difficult to plastically deform and process. This leads to the development of texture [Citation4,Citation6–8]. Recently, it was demonstrated that the Mg alloys strengthened by rare earth (RE) elements, for instance, gadolinium (Gd), can be successfully processed by multiaxial forging (MAF) such that a UFG structure with high strength and high ductility combination is obtained with the promise of a texture-free Mg RE alloy [Citation6–10]. The design, preparation and details of the MAF process for Mg-2Zn-2Gd alloy have been described previously [Citation6–10].
In sequel to the recent studies [Citation6–10], the objective of the study described here is to obtain a perspective and some fundamental insights on the weakening and development of texture-free Mg-2Zn-2Gd alloy processed by MAF by utilizing the recent results and the information documented in the literature. We first briefly revisit the microstructure and mechanical properties of Mg-2Zn-2Gd alloy in the following section prior to the presentation of recent results and discussion on the weakening of texture.
Microstructure and mechanical properties of Mg-2Zn-2Gd alloy processed by multiaxial forging
The as-cast annealed alloy with a coarse-grained (CG: average grain size of 44 ± 5 µm) microstructure is presented in together with the microstructure of the UFG-MAF alloy (UFG: average grain size of ~ 416 ± 140 nm) () [Citation6–8]. The mechanical properties were as follows: CG (yield strength: 46 ± 2 MPa, tensile strength: 70 ± 2 MPa, elongation: 7 ± 1%) and UFG (yield strength: 227 ± 2 MPa, tensile strength: 272 ± 1 MPa, elongation: 30 ± 2%). The increase in strength from 46 MPa in the CG alloy to 227 MPa in the UFG Mg-2Zn-2Gd alloy was obtained via combination of UFG structure and nanoscale precipitation of Mg3Zn3Gd () [Citation6–8].
Figure 1. (a) Microstructure of coarse-grained (CG) and (b) multiaxial forged ultrafine-grained (UFG) Mg-2Zn-2Gd alloy. (c) A representative transmission electron micrograph illustrating Mg3Zn3Gd precipitates in the α–mg matrix. (d) Electron diffraction pattern [Citation6–8].
![Figure 1. (a) Microstructure of coarse-grained (CG) and (b) multiaxial forged ultrafine-grained (UFG) Mg-2Zn-2Gd alloy. (c) A representative transmission electron micrograph illustrating Mg3Zn3Gd precipitates in the α–mg matrix. (d) Electron diffraction pattern [Citation6–8].](/cms/asset/cbde4c77-2ddb-4d29-8416-0912b2c26109/ymte_a_2321411_f0001_oc.jpg)
Texture results and discussion on the weakening of texture
The common textures in Mg alloys include basal texture (<0001>∥ND), commonly observed in the rolling deformation process, and double twin texture (<0001>∥RD), which typically forms during the extrusion process. Additionally, under specific processing conditions, such as in high-temperature environment, a random texture can form.
The pole figures in different formats for the CG alloy are presented in . Analyzing the {0001} pole figures in reveals the presence of basal texture and a strong near-twin texture, as shown in regions R1 and R2 of , with the texture intensity peak in region R2 at 52.59. There is a decrease in texture on {1–100} plane with a maximum value of ~24. Comprehensive analysis suggests strength on all the slip planes, indicative of significant texture in the CG Mg-2Zn-2Gd alloy.
Continuing the analysis of texture of UFG-MAF Mg-2Zn-2Gd alloy () and focusing mainly on the {0001} pole figures, it is evident that compared to the CG alloy (), the texture distribution in the UFG alloy is more dispersed and random. Both types of textures are present on the {0001} plane, with maximum texture intensity significantly reduced to 8.29. The texture on the remaining planes is weak.
In pure Mg, basal slip dominates, which generally leads to basal texture in mechanically worked alloys [Citation17]. The near-absence of texture in the experimental Mg-2Zn-2Gd alloy suggests that a significantly greater spread of grain orientations such that many grains are favourably oriented towards basal slip when the alloy is plastically deformed in the random direction [Citation4].
Some studies indicated that the addition of RE elements to Mg enhances elongation [Citation4,Citation18–21] and the formability is improved because of weakening of texture. For instance, small additions of Gd (~0.1 at.%) increased ductility and reduced yield anisotropy [Citation19,Citation22,Citation23]. The concentration of Gd required to weaken texture was below the solubility limit of Gd in Mg [Citation24–26]. This aspect was considered as a generic rule for the majority of the RE elements and implied that particles are not necessary to induce weakening of texture [Citation4]. Irrespective of the effect of RE addition, the deformation condition and presumably deformation mechanism was also considered to influence the final texture [Citation4]. This viewpoint was based on the observation that there was no increase in ductility when the addition of RE exceeded a critical value.
If we now consider that strong texture was present in the CG Gd-containing Mg alloy () and was almost absent in the UFG multiaxial forged alloy (), it then becomes important to consider the role of dynamic recrystallization and dynamic precipitation that occurs during multiaxial deformation and is responsible for the UFG structure. In this regard, it was noted that in both non-Gd and Gd-containing Mg alloys, the orientations of dynamic recrystallized nuclei were spread about the deformed grains [Citation27]. Based on texture studies on static and dynamic recrystallized alloys, it was proposed that RE addition not only weakens texture but also significantly retards dynamic recrystallization because of grain boundary segregation of RE atoms [Citation28–30]. However, it may be noted based on our observations that in the MAF Mg-2Zn-2Gd alloy, the UFG structure would not have been obtained in the absence of dynamic recrystallization. Preliminary studies on our experimental Mg-2Zn-2Gd alloy show the occurrence of continuous dynamic recrystallization with a necklace type structure (). This is envisaged to occur via continuous dynamic recrystallization [Citation31,Citation32] during the MAF process. Several extremely fine equiaxed grains formed in the vicinity of the elongated grains. Moreover, these elongated large grains with near <120> orientation were also broken or fragmented.
Figure 4. The occurrence of continuous dynamic recrystallization mechanism with a necklace-type structure in the multiaxial forged Mg-2Zn-2Gd alloy after one-pass. There are several ultrafine grains in the vicinity of elongated grains.

If we now consider the post-deformation of Mg-2Zn-2Gd alloy, besides the basal plane, a high percentage of prismatic and pyramidal dislocation activity was observed () [Citation6]. The enhanced activity on the non-basal slip systems is envisaged to impact the recrystallization process such that sub-grain structure forms at the grain boundaries [Citation32,Citation33], as observed in , and requires increased number of slip systems (). It is also likely that slow but continuous dynamic recrystallization led to extremely low intensity of texture. The UFG structure and strong weakening of texture is currently believed to be a cumulative contribution of MAF, activation of non-basal slip systems besides basal slip, continuous dynamic recrystallization and Mg3Zn3Gd-nanoparticle-simulated nucleation of new grains. Dynamic precipitation influences nucleation of new grains and restricts grain growth [Citation6]. Slow dynamic recrystallization may have contributed to the weakening of texture. Lastly, grain fragmentation via kinking mechanism during the MAF process cannot be ignored [Citation6–8].
Table 1. Frequency of dislocations observed in the UFG-MAF Mg-2Zn-2Gd alloy after post-deformation. The analysis is based on complete Burgers vector analysis in at least 25 random selected grains [Citation6].
Conclusions
The addition of the RE element, Gd, significantly weakens the texture in the multiaxial forged UFG Mg-2Zn-2Gd alloy. Other factors that are currently believed to have contributed to the UFG structure and weakening of texture are cumulative effect of severe plastic deformation, activation of slip systems besides basal slip, dynamic recrystallization and Mg3Zn3-Gd-nanoparticles-simulated nucleation of sub-grains.
Acknowledgments
The author is grateful to the National Science Foundation for financial support through grant # CMMI 2130586.
Disclosure statement
No potential conflict of interest was reported by the author(s).
Additional information
Funding
References
- Moreno IP, Nandy TK, Jones JW, et al. Microstructural characterization of a die-cast magnesium-rare earth alloy. Scripta Materialia. 2001;45(12):1423–6. doi: 10.1016/S1359-6462(01)01179-4
- Tsuji N, Saito Y, Utsunomiya H, et al. Ultra-fine grained bulk steel produced by accumulative roll-bonding (ARB) process. Scripta Materialia. 1999;40(7):795–800. doi: 10.1016/S1359-6462(99)00015-9
- Maier HJ, Gabor P, Gupta N, et al. Cyclic stress–strain response of ultrafine grained copper. Int J Fatigue. 2006;28(3):243–250. doi: 10.1016/j.ijfatigue.2005.05.004
- Griffiths D. Explaining texture weakening and improved formability in magnesium rare earth alloys. Mater Sci Technol. 2015;31(1):10. doi: 10.1179/1743284714Y.0000000632
- Polmear IJ. Light alloys: metallurgy of the light metals. Oxford, UK and Burlington, MA: Butterworth Heinemann; 1989.
- Misra RDK. Strong and ductile texture-free ultrafine-grained magnesium alloy via three-axial forging. Materials Letters. 2023;31:133443. doi: 10.1016/j.matlet.2022.133443
- Misra RDK. Enabling manufacturing of multi-axial forging-induced ultrafine-grained strong and ductile magnesium alloys: a perspective of process-structure-property paradigm. Mater Technol. 2023;38(1):2189769. doi: 10.1080/10667857.2023.2189769
- Weaver MR, Maldonado AJ, Banuelos JL, et al. On precipitation hardening behaviour in a triaxial forged mg-2Zn-2Gd alloy and relationship to mechanical properties. Mater Technol. 2023;38(1):2215038. doi: 10.1080/10667857.2023.2215038
- Trivedi P, Nune KC, Misra RDK. Grain refinement to submicron regime in multiaxial forged Mg-2Zn-2Gd alloy and relationship to mechanical properties. Mater Sci Eng A. 2016;668:59–65. doi: 10.1016/j.msea.2016.05.050
- Li K, Injeti VSY, Trivedi P, et al. Nanoscale deformation of multiaxially forged ultrafine-grained Mg-2Zn-2Gd alloy with high strength-high ductility combination and comparison with the coarse-grained counterpart. J Mater Sci Technol. 2018;34(2):311–316. doi: 10.1016/j.jmst.2017.07.023
- Guo L, Su X, Dai L, et al. Strain ageing embrittlement behaviour of X80 self-shielded flux-cored girth weld metal. Mater Technol Adv Perform Mater. 2023;38(1):2164978. doi: 10.1080/10667857.2023.2164978
- Yang C, Xu H, Wang Y, et al. Hot tearing analysis and process optimisation of the fire face of al-cu alloy cylinder head based on MAGMA numerical simulation. Mater Technol Adv Perform Mater. 2023;38(1):2165245. doi: 10.1080/10667857.2023.2165245
- Li Q, Zuo H, Feng J, et al. Strain rate and temperature sensitivity on the flow behaviour of a duplex stainless steel during hot deformation. Mater Technol Adv Perform Mater. 2023;38(1):2166216. doi: 10.1080/10667857.2023.2166216
- Li SF, Misra RDK, Liu ZQ. Towards strength-ductility synergy in nanosheets strengthened titanium matrix composites through laser power bed fusion of MXene/Ti composite powder. Mater Technol Adv Perform Mater. 2023;38(1):2181680. doi: 10.1080/10667857.2023.2181680
- Niu G, Zurpb H, Guyuen M, et al. Superior fracture toughness in a high-strength austenitic steel with heterogeneous lamellar microstructure. Acta Materialia. 2022;226:117462. doi: 10.1016/j.actamat.2022.117642
- Misra RDK, Challa VSA, Injeti YS. Phase reversion-induced nanostructured austenitic alloys: an overview. Mater Technol Adv Perform Mater. 2022;37(7):437–449. doi: 10.1080/10667857.2022.2065621
- Wang Y, Huang JC. Texture analysis in hexagonal materials. Materials Chemistry & Physics. 2003;81(1):11–26. doi: 10.1016/S0254-0584(03)00168-8
- Agnew SR, Nie J. Preface to the viewpoint set on: the current state of magnesium alloy science and technology. Scripta Materialia. 2010;63(7):671–673. doi: 10.1016/j.scriptamat.2010.06.029
- Bohlen J, Nurnberg MR, Senn JW, et al. The texture and anisotropy of magnesium–zinc–rare earth alloy sheets. Acta Materialia. 2007;55(6):2101–2112. doi: 10.1016/j.actamat.2006.11.013
- Stanford N, Atwell D, Beer A, et al. Effect of microalloying with rare-earth elements on the texture of extruded magnesium-based alloys. Scripta Materialia. 2008;59(7):772–775. doi: 10.1016/j.scriptamat.2008.06.008
- Al-Samman T, Li X. Sheet texture modification in magnesium-based alloys by selective rare earth alloying. Mater Sci Eng A. 2011;528(10–11):3809–3822. doi: 10.1016/j.msea.2011.01.080
- Agnew SR, Duygulu O. Plastic anisotropy and the role of non-basal slip in magnesium alloy AZ31B. Int J Plast. 2005;21(6):1161–1193. doi: 10.1016/j.ijplas.2004.05.018
- Farzadfar A, Martin E, Sanjari M, et al. On the deformation, recrystallization and texture of hot-rolled Mg–2.9Y and Mg–2.9Zn solid solution alloys—A comparative study. Mater Sci Eng A. 2012;534:209–219. doi: 10.1016/j.msea.2011.11.061
- Hantzsche K, Bohlen J, Wendt J, et al. Effect of rare earth additions on microstructure and texture development of magnesium alloy sheets. Scripta Materialia. 2010;63(7):725–730. doi: 10.1016/j.scriptamat.2009.12.033
- Stanford N. Micro-alloying mg with Y, ce, Gd and La for texture modification—A comparative study. Mater Sci Eng A. 2010;527(10–11):2669–2677. doi: 10.1016/j.msea.2009.12.036
- Hadorn J, Hantzsche K, Yi S, et al. Role of solute in the texture modification during hot deformation of mg-rare earth alloys. Metall Mater Trans A. 2012;43(4):1347–1362. doi: 10.1007/s11661-011-0923-5
- Stanford N. The effect of rare earth elements on the behaviour of magnesium-based alloys: part 2 – recrystallisation and texture development. Mater Sci Eng A. 2013;565:469–475. doi: 10.1016/j.msea.2012.10.084
- Farzadfar SA, Martin E, Sanjari M, et al. Texture weakening and static recrystallization in rolled Mg–2.9Y and Mg–2.9Zn solid solution alloys. J Mater Sci. 2012;47(14):5488. doi: 10.1007/s10853-012-6440-0
- Cottam R, Robson J, Lorimer G, et al. Dynamic recrystallization of mg and mg–Y alloys: crystallographic texture development. Mater Sci Eng A. 2008;485(1–2):375–382. doi: 10.1016/j.msea.2007.08.016
- Stanford N, Barnett MR. Effect of composition on the texture and deformation behaviour of wrought mg alloys. Scripta Materialia. 2008;58(3):179–182. doi: 10.1016/j.scriptamat.2007.09.054
- Humphreys FJ, Hatherly M. Recrystallization and related annealing phenomena. Oxford: Elsevier; 1995.
- Galiyev A, Kaibyshev R, Gottstein G. Correlation of plastic deformation and dynamic recrystallization in magnesium alloy ZK60. Acta Materialia. 2001;49(7):1199–1207. doi: 10.1016/S1359-6454(01)00020-9
- Ion S, Humphreys FJ, White S. Dynamic recrystallisation and the development of microstructure during the high temperature deformation of magnesium. Acta Metall. 1982;30(10):1909–1919. doi: 10.1016/0001-6160(82)90031-1