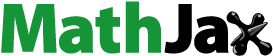
Abstract
Context
Coix [Coix lacryma-jobi L. var. mayuen (Roman.) Stapf (Poaceae)], a crop of medicinal and edible significance, contains coixol, which has demonstrated anticancer properties. However, the limited solubility of coixol restricts its potential therapeutic applications.
Objective
This study prepared a water-soluble coixol-β-cyclodextrin polymer (CDP) inclusion compound and evaluated its anticancer effect.
Materials and methods
The coixol-CDP compound was synthesized through a solvent-stirring and freeze-drying technique. Its coixol content was quantified using HPLC, and its stability was tested under various conditions. The anticancer effects of the coixol-CDP compound (4.129, 8.259, 16.518, and 33.035 mg/L for 24, 48, and 72 h) on the proliferation of non-small cell lung cancer (NSCLC) A549 cells were evaluated using an MTT assay; cell morphology was examined by Hoechst nuclear staining; apoptosis and cell cycle was detected by flow cytometry; and the expression of apoptosis-related proteins was assessed by Western blots.
Results
The water-soluble coixol-CDP inclusion compound was successfully prepared with an inclusion ratio of 86.6% and an inclusion yield rate of 84.1%. The coixol content of the compound was 5.63% and the compound remained stable under various conditions. Compared to coixol alone, all 24, 48, and 72 h administrations with the coixol-CDP compound exhibited lower IC50 values (33.93 ± 2.28, 16.80 ± 1.46, and 6.93 ± 0.83 mg/L) in A549 cells; the compound also showed stronger regulatory effects on apoptosis-related proteins.
Discussion and conclusions
These findings offer a new perspective for the potential clinical application of Coix in NSCLC therapy and its future research.
Introduction
Coix [Coix lacryma-jobi L. var. mayuen (Roman.) Stapf (Poaceae)] is a versatile crop with medicinal and edible properties cultivated in various regions worldwide. Over the last decade, it has gained significant recognition in China as ‘the health crop of life’ due to its abundant nutrients and benefits. Coix is prominently used as a valuable ingredient in functional foods and related products, such as Coix seed wine, Coix cake, and Coix drink (Tan et al. Citation2017; Tian et al. Citation2017; Yang YS et al. Citation2017; Yang ZT et al. Citation2017; Zhang et al. Citation2018). Coix contains numerous active components. One of the main active components is coixol which is effective in various cancers, including sarcoma, liver, stomach, and lung cancers (Hsu et al. Citation2003; Latha et al. Citation2009; Chung et al. Citation2011; Jiang and Miao Citation2014; Lee et al. Citation2015; Sharma et al. Citation2015; Ali et al. Citation2017; Hameed et al. Citation2019; Igbokwe et al. Citation2021; Suzuki and Konaya Citation2021; Zhu et al. Citation2018). Presently, coixol is used to produce an anticancer medicine called ‘Kanglaite’, available in the form of Coix seed oil capsules and injections, widely used in China for cancer treatment. However, the clinical application of coixol preparations faces challenges due to their low solubility, resulting in reduced biological absorption. Furthermore, the pharmacological mechanism of coixol is not fully understood, warranting further investigation.
Cyclodextrins (CD) are cyclic oligosaccharides connected by α-1, 4-glucosidic bonds, comprising α-CD, β-CD, and γ-CD. CDs possess unique molecular structures featuring hydrophobic internal cavities and hydrophilic external surfaces. These characteristics enable CD molecules to form inclusion complexes, thus improving the water solubility, dissolution rate, and bioavailability of drugs with low water solubility (Liu et al. Citation2021). Consequently, CDs have found extensive applications in various fields, including electroanalysis (Zhu et al. Citation2012), biological medicine (Delogu et al. Citation2016), and environmental protection (Morillo et al. Citation2014). However, certain drawbacks hinder the full-scale implementation of CDs, such as their potential for strong haemolysis effects and nephrotoxicity (Liu et al. Citation2021). Researchers have developed amorphous and non-crystalline derivatives to overcome these limitations through chemical modifications of native CDs (Morin-Crini and Crini Citation2013). A notable derivative is β-cyclodextrin polymer (CDP), obtained by reacting β-CD with epichlorohydrin (EPI) (Gidwani and Vyas Citation2014). Studies have shown that the CDP host polymer exhibits improved water solubility, dissolution rate, and bioavailability compared to native β-CD (di Cagno et al. Citation2014).
In this study, we synthesized a water-soluble inclusion compound of coixol with β-cyclodextrin polymer. The physical properties of this compound were thoroughly examined. Subsequently, we evaluated the in vitro anticancer effects of this coixol inclusion compound using A549 cells, a type of non-small cell lung cancer (NSCLC). The findings of this research present a promising option for the effective use of coixol in both food and clinical applications. Furthermore, the study provides valuable information for cultivating new exceptional varieties of germplasm resources and contributes to expanding the development of Coix resources.
Materials and methods
Preparation of CDP
The preparation of β-cyclodextrin polymer involved the following steps: β-CD was dissolved in 30% NaOH and heated to 30 °C in a water bath, followed by adding EPI and reacting at 30 °C for 24 h (EPI:β-CD:30% NaOH = 1:2:5). After completion of the reaction, the solution was cooled to room temperature and then dialysed against distilled water using a dialysis bag (Solarbio, Beijing, China) placed in a beaker. The distilled water was replaced every hour until the reaction solution reached a neutral pH. The content inside the dialysis bag was transferred to a beaker. To precipitate the white flocculent CDP, absolute ethanol was slowly added to the beaker. The precipitate was then filtered under vacuum for 6 h at 60 °C (Xu et al. Citation2017b).
Preparation of the coixol-CDP inclusion compound
Based on our previous research (Xu et al. Citation2017a) and pilot experiments, coixol (Shanghai Yuanye Biotechnology Co., Ltd., 100% purity, batch number: YM0325YB14, China) was mixed with CDP (molar crosslinking degree 8.47) in molar ratios of 8:1, 8:2, and 8:3. The precise weights of CDP were 24.01, 48.02, and 72.03 g, respectively. The samples were immersed in 350 mL of distilled water and allowed to swell for 1 h before being dissolved at 3500 rpm. Three portions of 3.31 g of coixol were accurately weighed and dissolved in a small amount of absolute ethanol. The coixol solution was then added dropwise to each of the three portions of the CDP solution. After stirring for 6 h, a coixol-CDP inclusion compound was formed. The resulting mixture was pre-frozen at −80 °C for 24 h and then subjected to freeze drying using the FreeZone 1-Liter Benchtop Freeze Dry System (LABCONCO Company, USA) for 48 h to obtain the lyophilized powder of the coixol-CDP inclusion compound.
Characterization of CDP and coixol inclusion compound
Scanning electron microscopy
The appropriate amounts of β-CD, CDP, coixol, a physical mixture of CDP and coixol, and the coixol-CDP inclusion compound powder were subjected to conductive adhesive bonding and gold plating and then observed under a high vacuum mode of 15 KV using a field emission scanning electron microscope (Hitachi Company, Japan).
Fourier transform infrared spectroscopy
We precisely weighed 30.2 mg of β-CD and CDP, which were dried under infrared light, together with 1.8 mg of coixol. Furthermore, we combined 30.2 mg of CDP with 1.8 mg of coixol and 32.0 mg of the coixol-CDP inclusion compound. β-CD and CDP were obtained with an external content of 0.6 mg per sheet. A Fourier transform Tensor 27 Infrared Spectrometer (Bruker, Billerica, MA, USA) was used to analyse the infrared absorption (4000 to 400 cm−1).
Differential scanning calorimetry
The differential scanning calorimetry (DSC) analysis was performed using a PerkinElmer DSC 8500 instrument (Waltham, MA, USA). For each sample, 1.5 mg was used. The heating rate was 10 °C/min with a nitrogen purge gas (20 mL/min). The temperature range was set between 40 °C and 200 °C.
X-ray diffraction
Following the above method, 8 mg of each sample was collected. X-ray diffraction analysis (XSD) was performed with a scanning angle ranging from 5° to 40°, using Cu-Kα radiation. The tube voltage and current were set at 40 kV and 40 mA, respectively. The scanning speed was adjusted to 5°/min. The analysis was performed using a Bruker Lynxeye array detector (Billerica, MA, USA). Changes in the diffraction peak of the samples were compared to complete the XSD analysis for each sample.
Preparation of standard solution and test solution
A coixol standard (4.0 mg) was dissolved in the mobile phase and then diluted to a final volume of 10 mL using a brown volumetric flask, resulting in a 0.4 mg/mL standard coixol stock solution. Subsequently, 0.2 mL of the standard coixol stock solution was taken and further diluted with the mobile phase to a final volume of 10 mL, producing an 8 μg/mL coixol standard solution.
The coixol-CDP compound powder (6.0 mg) was dissolved in absolute ethanol and then diluted to a final volume of 25 mL using a volumetric flask. Subsequently, the solution was centrifuged, and the supernatant was collected. After the anhydrous ethanol was evaporated, the resulting supernatant was further diluted with the mobile phase to a final volume of 100 mL, producing the unpacked test solution of the coixol-CDP compound.
The comparison sample solution was prepared following the same method described above, except that coixol was not added. The negative test solution was prepared using CDP following the same preparation method described above.
Determination of the detection wavelength
The coixol standard solution (1 mL) and the negative sample solution (1 mL) were diluted to 10 mL with the mobile phase. The samples were scanned using a U-3900 ultraviolet spectrophotometer (Shanghai Heli Instrument Co. Ltd., China) in the 200–400 nm wavelength range. Coixol exhibited two ultraviolet absorption peaks at 232 and 290 nm (). To enhance the detection sensitivity and precision, 290 nm was selected as the quantitative wavelength for the coixol-CDP compound. This choice was made to avoid CDP interference.
Chromatographic conditions
The analytical set-up involved an e2695 high-performance liquid chromatography (HPLC) and a 2998 PDA detector (Waters Company (USA). The column used was Symmetry C18 (Waters Company, USA, 5 μm, 4.6 × 250 mm). The mobile phase consisted of acetonitrile: 0.1% phosphoric acid aqueous solution in a ratio of 30:70. The flow rate was 1 mL/min, and the column temperature was set at 25 ± 5 °C. UV detection was performed at a wavelength of 290 nm, and each sample injection size was 20 μL.
Specificity test
Under chromatographic conditions, the coixol standard solution, the test solution, and the negative test solution were analysed. Their respective chromatograms were recorded (). The chromatogram for the free coixol standard showed a peak at 7.694 min (), while the unpacked coixol in the test solution exhibited a peak at 7.690 min (). Importantly, no peak was observed for CDP, indicating the high specificity of our method ().
Standard curve for determining coixol content by HPLC
A set of standard coixol solutions (5, 10, 25, 50, 75, and 150 μg/mL) were prepared by diluting different volumes (0.125, 0.25, 0.5, 1.25, 2.5, and 3.75 mL) of standard coixol stock solution (0.4 mg/mL) into a final volume of 10 mL using HPLC mobile phase. These standard solutions were then subjected to chromatographic analysis under the established conditions.
Linear regression was performed using the concentration (μg/mL) as the abscissa (x) and the corresponding chromatographic peak area as the ordinate (y). The regression equation obtained is y = 142948x − 33981 (R2 = 0.9998), indicating a highly satisfactory linear relationship within the concentration range of 5-150 μg/mL ().
Coixol HPLC quantitative precision test
Standard coixol solutions (10, 50, and 150 μg/mL) were analysed separately under established chromatographic conditions. The results are presented in . The relative standard deviation (RSD%) of the peak area at different concentrations was 0.74%, 0.99%, and 0.77%, respectively. These RSD values indicate satisfactory precision in the detection method.
Table 1. The accuracy test of coixol.
Quantitative stability test coixol high-performance liquid chromatography
Standard coixol solutions with concentrations of 10, 50, and 150 μg/mL were loaded onto the chromatograph after being kept at room temperature for different time intervals: 2, 6, 12, 18, and 24 h. The RSD% values of the peak area detected at each time point were 0.59%, 1.15%, and 0.39%, respectively. These results demonstrate that the detection stability was satisfactory within 24 h ().
Table 2. The stability test of coixol.
Coixol HPLC quantitative sample recovery test
Nine aliquots (1 mL each, containing 13.5 μg of coixol) of the same batch of coixol-CDP compound were divided into low, medium, and high groups. Standard coixol solutions containing 5.5, 15.5, and 25.5 μg of coixol were sequentially added to each group. The standard solutions were thoroughly mixed and subsequently analyzed under chromatographic conditions. The average recovery of the sample was determined to be 99.92%, with an RSD% of 0.73 (). These results demonstrate that our method achieves high quantitative accuracy and a good sample recovery rate.
Table 3. The average recovery test of coixol.
Quantitative analysis of the coixol-CDP compound
An adequate amount of coixol-CDP compound was used in various molar ratios to prepare the test solutions, which were subsequently analysed under the established chromatographic conditions. Following the analysis, the average inclusion rate, yield rate, and comprehensive score were calculated based on the data obtained (Xu and Liu Citation2018).
Investigation of the stability of the coixol-CDP compound
Nine portions of coixol-CDP compound powder (1 g per part) were taken and distributed in culture plates. The samples were divided into three groups: (1) light exposure group: three parts were continuously irradiated in a 4500 ± 500LX light box at 25 °C; (2) high-temperature group: three parts were placed in an oven at 60 °C; and (3) humidity exposure group: the remaining three parts were placed in a closed container with saturated NaCl solution to maintain a relative humidity of 75 ± 5% at 25 ± 2 °C for 10 days. The appearance, rehydration capacity, inclusion ratio, and coixol content of the coixol-CDP compound were examined before and after treatment in each group.
Preparation of the solution of the thiazole blue (MTT) assay
Preparation of coixol solutions: To create the mother liquor at a concentration of 3.3035 g/L, 49.52 mg of coixol was dissolved in 14.89 mL of Dulbecco’s Modified Eagle Medium (DMEM) cell culture medium (Gibco, Waltham, MA, USA), along with 0.1 mL of dimethyl sulphoxide (DMSO) (Sinopharm Chemical Reagent, Beijing, China). Subsequently, 62.5, 125, 250, and 500 μL of the mother liquor were mixed with 49.9375, 49.875, 49.75, and 49.5 mL of medium, respectively, to obtain coixol solutions with concentrations of 4.129, 8.259, 16.518, and 33.035 mg/L. All coixol solutions were properly stored at 4 °C for future use.
Coixol-CDP Compound Solutions: a mother liquor (3.3035 mg/L) was prepared by dissolving 879.57 mg of the coixol-CDP compound in 14.99 mL of the medium. Next, 62.5, 125, 250, and 500 μL of mother liquor were mixed with 49.9375, 49.875, 49.75, and 49.5 mL of medium, respectively. As a result, coixol-CDP compound solutions with concentrations of 4.129, 8.259, 16.518, and 33.035 mg/L were obtained. All solutions were stored properly at 4 °C for future use.
Thiazole Blue (MTT) solution: The MTT solution was prepared by dissolving 0.5 g of MTT powder (Sigma-Aldrich, St. Louis, MO, USA) in 100 mL of phosphate-buffered saline (PBS) (HyClone, Marlborough, MA, USA).
Cell culture and detection of proliferation inhibition rate
NSCLC A549 cells (purchased from the Institute of Biochemistry and Cell Biology, Chinese Academy of Sciences) in the logarithmic growth phase were digested with trypsin, followed by centrifugation at 1000 rpm for 5 min. An appropriate amount of cell culture solution was added to the supernatant. Approximately, 4000 cells per well were seeded in 96-well plates and incubated overnight in a Thermo Series II CO2 cell incubator (Thermo Scientific, Waltham, MA, USA). After the cells had attached to the wells, 100 μL of different concentrations of coixol solutions and coixol-CDP compound solutions were added, with 5 replicate wells per group. The cells were further incubated for 24, 48, and 72 h. Following each incubation, 10 μL of MTT reagent (5 mg/mL) was added to each well.
After incubation for 4 h, the upper culture medium was discarded, and 100 μL of DMSO was added to each well, followed by shaking for 10 min to completely dissolve the blue crystal in the wells. The absorbance optical density (OD) value of each well was measured at 570 nm using an EnSpire multifunction microplate reader (PerkinElmer, Waltham, MA, USA). The inhibition rate of cell proliferation affected by coixol and the coixol-CDP compound was calculated using the following formula (Zhao et al. Citation2018; Priyanga et al. Citation2019; Rahayu and Sularsih Citation2019).
Hoechst staining
The cell density was adjusted to 1 × 105 cells/mL. The cells (2 mL) were then inoculated in 6-well plates and cultured until the cells were fully attached. Different concentrations (0, 8.259, 16.518, and 33.035 mg/L) of coixol or the coixol-CDP inclusion compound were added in a volume of 2 mL per well. After incubation for 24 h, the upper medium was discarded, and 0.5 mL of 4% paraformaldehyde was added to cover the surface of the cells. The cells were then fixed in a cell culture incubator for 30 min. After discarding the supernatant, 0.1 mL of 5% Hoechst33258 staining solution (Nanjing Kaiji Biotechnology, Jiangsu, China) was added to each well and stained at room temperature for 10 min in the dark. Cell morphology was observed under 340 nm excitation light using an inverted microscope (Olympus Company, Japan) (Song et al. Citation2014).
Annexin V-FITC/PI double staining
A549 cells were cultured and treated with different concentrations of coixol or the coixol-CDP inclusion compound, as described above. After incubation for 24 h, the suspended cells were collected and combined with adherent cells obtained by trypsinization and centrifugation. The combined cells were centrifuged at 1000 rpm for 5 min, and the supernatant was discarded. Next, 500 μL of binding buffer was added to suspend the cells, followed by 5 μL of Annexin V-fluorescein isothiocyanate (FITC) and -propidium iodide (PI) staining solution (Nanjing Kaiji Biotechnology Co., Ltd., China). Apoptotic cells were detected and analyzed using Calibur FACS flow cytometry (BD Company, USA), and the apoptotic rate was calculated (Zhao et al. Citation2017).
Cell cycle detection
A549 cells were cultured and treated with different concentrations of coixol or the coixol-CDP inclusion compound, as described above. After incubation for 24 h, the suspended cells were collected and fixed with 700 μL of absolute ethanol at 4 °C for 12 h. After centrifugation at 1000 rpm for 5 min and discarding the supernatant, the cells were stained with PI and RNaseA staining solution (Nanjing Kaiji Biotechnology Co., Ltd., China) in the staining buffer, following the manufacturer’s instructions. Cell cycle analysis was performed using flow cytometry (Wang et al. Citation2017).
Western blots
A549 cells were cultured and treated with different concentrations of coixol or the coixol-CDP inclusion compound, as described above. Cell lysate was added to each well to extract cellular proteins. The protein content was determined using the Pierce BCA protein assay kit (Thermo Scientific, Waltham, MA, USA) with a standard equation y = 0.0011x + 0.1551 (R2 = 0.9983). The total protein concentration of each sample was used as a reference for loading the samples onto the electrophoresis gel. The cell protein solution was mixed with 1/5 volume of 5× loading buffer and heated at 100 °C for 15 min to denature the proteins before analysis by electrophoresis.
Western blot experiments were conducted following previously described methods (Pan et al. Citation2016, Citation2018, Citation2022a, Citation2022b, Citation2023a, Citation2023b; Pan and Deng Citation2019). Briefly, separation and concentrated gel were prepared using acrylamide (Acr), bisacrylamide (Bis), tris(hydroxymethyl)aminomethane (Tris), sodium dodecyl sulphate (SDS), N,N,N′,N′-tetramethyl ethylenediamine (TEMED), ammonium persulphate (APS), etc., according to the manufacturer’s instructions (Solarbio, Beijing, China). Samples containing 20 μg of protein were loaded into each gel well. The running system was set to 110 V for 30 min. Subsequently, all samples were transferred from gels to polyvinylidene difluoride (PVDF) membranes at 200 V for 1.5 h. After blocking with 5% bovine albumin (BSA) for 1 h at room temperature, all PVDF membranes were incubated with primary antibodies diluted in 1% BSA overnight at 4 °C. The next day, after incubation with a secondary antibody, protein bands were visualized using a ChemiDoc XRS + chemiluminescence imaging system (Bio-Rad, Hercules, CA, USA). ImageJ software was used to analyse the grey values of the bands. The data were then corrected based on their corresponding actin levels.
Statistical analysis
All values are presented as means ± standard deviation (x ± s). Statistical analysis was conducted using one-way analysis of variance (ANOVA), followed by Student–Newman–Keuls tests to determine significant differences between the two groups. p < 0.05 was considered statistically significant. To ensure consistency, all experiments were performed in triplicate.
Results
Quantitative analysis of the coixol-CDP inclusion compound
The following formulas were used to calculate the inclusion rate, the yield rate, the coixol content, and the comprehensive score of the coixol-CDP inclusion compound. Inclusion rate = (mcompound/(mcoixol + mCDP)) × 100%; yield rate = (mcoixol in the compound/mcoixol) × 100%; coixol content = (mcoixol/mcompound) × 100%; and, comprehensive score = inclusion rate × 0.2 + yield rate × 0.8 (). Scheme B was selected to prepare the coixol-CDP inclusion compound, because, in Scheme B, the coixol content (5.63%) obtained from the HPLC data was the highest among the three schemes.
Table 4. The yield and inclusion rate of coixol-CDP compounds.
Characterization of the coixol-CDP inclusion compound
Stability of the coixol-CDP inclusion compound
The data indicated that the coixol-CDP inclusion compound was stable under various conditions ().
Table 5. Data of light stability experiments.
Scanning electron microscopy
β-CD showed a rough and loose block morphology (). CDP exhibited a smooth and compact block structure (). Coixol is presented as columnar crystals (). The physical mixture of CDP and coixol showed irregular blocks of CDP and columnar crystals of coixol (). The coixol-CDP inclusion compound appeared as irregular long plates (). Significant morphological differences suggested that the molecules changed lattice arrangement, forming a new phase, indicating the successful synthesis of the CDP and coixol-CDP inclusion compound (Upadhyay and Ali Citation2018).
Fourier transform infrared spectroscopy
The strong -OH absorption peak of β-CD at 3,369 cm−1 () shifted to 3,456 cm−1 in CDP (). The peak shape became wider, and the absorption became weaker, indicating that the vibration of -OH after polymerization required higher energy to be excited. On the map of the physical mixture of coixol and CDP, a simple superposition absorption peak of CDP and coixol was observed (). The disappearance of the stretching vibration peaks of -CH, -CH3, and -NH presented by coixol at 3,097, 2,763, 1,957, 1,936, and 1,739 cm−1 () after being included in CDP () suggests that the corresponding vibrations might be blocked in the CDP cavity. However, the peaks of C = O and C = C of coixol at 1,790 cm−1 and 1,643 cm−1 were still observed, although their intensity became weaker after being enclosed by CDP (). The weakening of these peaks may be attributed to the formation of repulsive dipoles or hydrogen bonds between the corresponding functional groups of coixol and the -OH groups of CDP. This interaction weakened the OH bond strength of CDP, leading to its characteristic absorption peak at 3,456 cm−1, shifting to a lower frequency of 3399 cm−1 after the inclusion of coixol.
Differential scanning calorimetry
The heat absorption peaks of β-CD and CDP were observed at 123.02 °C and 109.72 °C, respectively (). Due to the higher molecular weight and lower crystallinity of CDP, its melting point is lower than that of β-CD. After physically mixing CDP with coixol, the heat absorption peak of CDP reduced to 102.62 °C, and the absorption peak of coixol decreased slightly from 155.84 °C to 155.49 °C (). This indicates that they slightly impact each other, as previously reported (Amiri and Nalbandi Citation2018; Mikhail et al. Citation2018).
Figure 6. DSC figures of different samples. (A) β-CD; (B) CDP; (C) coixol; (D) physical mixture of CDP and coixol; (E) coixol-CDP inclusion compound.
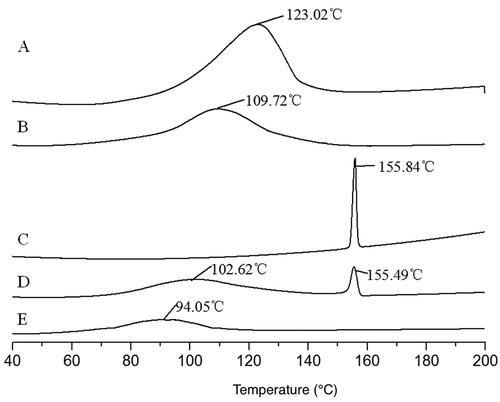
In the coixol-CDP inclusion compound, the heat absorption peaks of CDP and coixol were not observed. Instead, a new heat absorption peak appeared at 94.05 °C (), suggesting a significant reduction in crystallinity and melting point of the inclusion compound (Yildiz et al. Citation2018). This indicates that coixol entered the CDP cavity, leading to the coexistence of both compounds in a new phase.
X-ray diffraction
β-CD displayed several sharp crystallization peaks at 10.64°, 12.48°, 17.68°, and 19.62° (), indicating its crystalline nature. On the other hand, CDP showed a broad and diffuse peak (), indicating its amorphous phase. Coixol, being a crystalline compound, exhibited multiple sharp crystallization peaks (). In the physical mixture of coixol and CDP, coixol crystallization peaks (with reduced intensity and the number of peaks) and diffuse CDP peaks were observed (), indicating a weak interaction between the two compounds.
Figure 7. XRD figures of different samples. (A) β-CD; (B) CDP; (C) coixol; (D) physical mixture of CDP and coixol; (E) coixol-CDP inclusion compound.
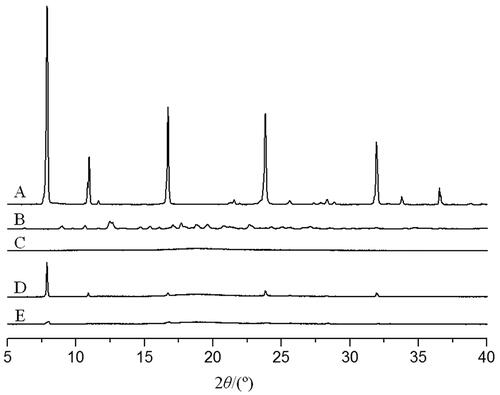
In the diffraction pattern of the coixol-CDP inclusion compound, the crystallization peaks of coixol at 7.88° and 16.71° were markedly weak, and the characteristic peaks of coixol at 10.97°, 23.82°, 31.95°, and 36.5° disappeared (). The diffuse peak observed was similar to that of CDP, indicating that CDP inhibited the crystallization of coixol. This suggests that CDP encapsulated coixol to form a new phase, suppressing coixol crystallization within the inclusion compound.
MTT assay
The MTT experiments demonstrated that different concentrations of coixol significantly inhibited the growth and proliferation of A549 cells () (). The inhibition rate of coixol in A549 cells increased with time, the concentration of coixol, and its inclusion compound. The IC50 values of coixol and its inclusion compound at 24, 48, and 72 h were as follows: 82.04 ± 5.37, 36.17 ± 3.25, and 16.15 ± 1.39 mg/L for coixol, and 33.93 ± 2.28, 16.80 ± 1.46, and 6.93 ± 0.83 mg/L for the inclusion compound coixol-CDP, respectively. Compared to the coixol-only group, the coixol-CDP inclusion compound group exhibited a stronger inhibitory effect on the growth of A549 cells at the same dose (all p < 0.05).
Figure 8. Inhibitory effects of coixol and the coixol-CDP inclusion compound on the A549 cells (x ± s, n = 3). Note: the coixol-CDP inclusion compound compares with coixol (**p < 0.01).
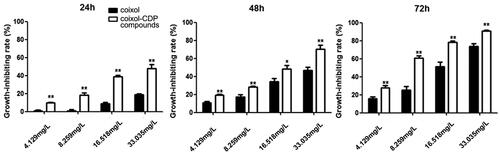
Table 6. The inhibition effects of the coixol-CDP inclusion compound on A549 cells (x ± s, n = 3).
Hoechst staining
The results of Hoechst staining revealed fluorescence staining of A549 cells (). Treatment with different concentrations of coixol and the coixol-CDP inclusion compound increased the number of apoptotic bodies in each group, indicating that coixol induced cell apoptosis, inhibiting cell growth. In particular, at the same dose, the coixol-CDP inclusion compound demonstrated a greater ability to produce apoptotic bodies than coixol alone.
Figure 9. Hoechst33258 Fluorescent staining assay for morphological changes of the A549 cells induced by coixol and the coixol-CDP inclusion compound (×200). (A, B, C, D) the concentrations of coixol are 0, 8.259, 16.518, and 33.035 mg/L in sequence. (A′, B′, C′, D′) the concentrations of coixol-CDP inclusion compound are 0, 8.259, 16.518, and 33.035 mg/L in sequence.
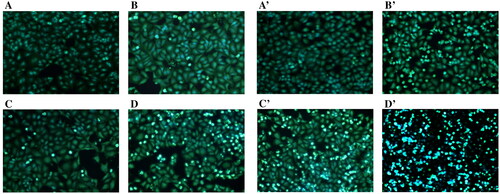
Annexin V-FITC/PI assay
The annexin V-FITC/PI assay showed a significant increase in the ratio of early and late apoptosis in all experimental groups compared to the control group (). Furthermore, compared to the coixol group, the coixol-CDP inclusion compound induced a more significant level of apoptosis in A549 cells at the same dose (all p < 0.01) (). These results were consistent with the results of the staining experiment, collectively indicating that both coixol and the coixol-CDP inclusion compound can induce apoptosis and inhibit cell proliferation in A549 cells. Furthermore, the pro-apoptotic effect of coixol on A549 cells was significantly increased when coixol was included in CDP ().
Figure 10. Flow cytometry results. (A, B, C, D) The concentrations of coixol are 0, 8.259, 16.518, and 33.035 mg/L in sequence; (A′, B′, C′, D′) the concentrations of the coixol-CDP inclusion compound are 0, 8.259, 16.518, and 33.035 mg/L in sequence. (E) The apoptotic effects of coixol and the coixol-CDP inclusion compound on the A549 cells (x ± s, n = 3). Note: the coixol-CDP inclusion compound compares with coixol (** p < 0.01).
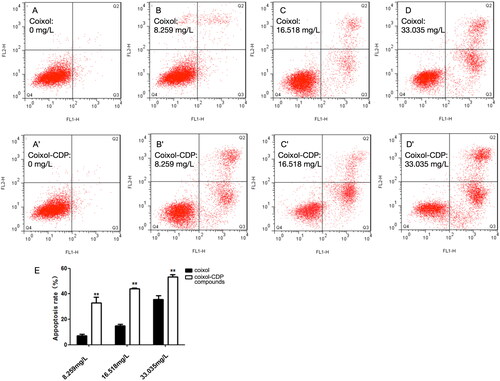
Cell cycle detection
The ratio of cells in phase S and phase G2/M increased dose-dependently after applying coixol and the coixol-CDP inclusion compound to A549 cells (). These results indicate that coixol and the coixol-CDP inclusion compound effectively arrested A549 cells in the S and G2/M phases of the cell cycle. Furthermore, at the same dose, the coixol-CDP inclusion compound demonstrated a more significant effect in arresting A549 cells in the G0/G1 phase compared to coixol alone (p < 0.05 or 0.01) (). This finding suggests that the coixol-CDP inclusion compound possesses a higher level of biological activity regarding cell cycle arrest than coixol alone.
Figure 11. Cell cycle examination on the A549 cells. (A, B, C, D) The concentrations of coixol are 0, 8.259, 16.518, and 33.035 mg/L in sequence; (A′, B′, C′, D′) the concentrations of the coixol-CDP inclusion compound are 0, 8.259, 16.518, and 33.035 mg/L in sequence. (E) The cycle effect of coixol and its compounds on A549 cell (x ± s, n = 3). (*, p < 0.05 vs. corresponding coixol; **, p < 0.01 vs. corresponding coixol).
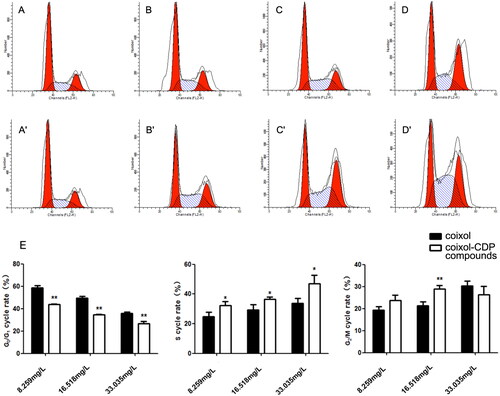
Western blots
The Western blot results demonstrated that both coixol and the coixol-CDP inclusion compound up-regulated the protein expression of cleaved-Caspase-3, Caspase-8, Caspase-9, and B-cell lymphoma 2 (Bcl-2) associated X-protein (Bax) while down-regulating Bcl-2 in A549 cells in a concentration-dependent manner (). These findings suggest that coixol regulates apoptosis by inducing the Bax/Bcl-2 protein ratio and then triggers the caspase cascade. It should be noted that, at the same dose, the regulatory effects of the coixol-CDP inclusion compound on the expression of these proteins were significantly more pronounced compared to the coixol group (most p < 0.05). This indicates that the biological activity of coixol was significantly enhanced by its inclusion in CDP.
Discussion
Coix is a functional crop known for its various health benefits. Coixol, as an active component of Coix, has been extensively studied for its anticancer properties. However, the low solubility of coixol has limited its clinical application. In this study, we synthesized a coixol-CDP inclusion compound using CDP, which showed improved water solubility and favourable physical properties compared to coixol alone. Furthermore, this new coixol-CDP inclusion compound demonstrated enhanced anticancer effects compared to coixol. Its potential as an effective anticancer drug, particularly for NSCLC, holds great promise for future therapeutic applications.
By forming an inclusion compound with CDP, the aqueous solubility and bioavailability of coixol were significantly improved, as reported in previous studies (di Cagno et al. Citation2014). Extensive examinations of the physical properties of this compound confirmed that coixol was effectively included in the CDP, resulting in a stable inclusion compound that could withstand various environmental conditions. These favorable physical properties indicate that the coixol-CDP inclusion compound has great potential for easy production, storage, transportation, and clinical use.
In our in vitro experiments, coixol effectively inhibited NSCLC A549 cells. These results are consistent with previous findings and further validate the anticancer effects of coixol, a key active ingredient in widely used anticancer preparations, ‘Kanglaite’ injections, and soft capsules in China. Additionally, we successfully synthesized a stable and water-soluble coixol-CDP inclusion compound, converting water-insoluble coixol into a water-soluble form. Subsequent in vitro experiments revealed that the coixol-CDP inclusion compound exhibited improved anticancer biological activity in A549 cells compared to coixol alone. The enhanced anticancer effect of the coixol-CDP inclusion compound can be attributed to its high-water solubility, which potentially increases the biological absorption of coixol, consequently enhancing its overall biological effects. These findings expand the scope of potential clinical applications of coixol and provide a promising direction for developing new coixol preparations with enhanced therapeutic properties.
Numerous reports have been made on the anticancer properties of various ingredients found in Coix, such as lipids, fatty acids, and sugars. However, the pharmacological activity and formulation of other components, particularly coixol, have not been extensively studied. In this study, we successfully synthesized a new coixol-CDP inclusion compound and evaluated its physical properties and anticancer effects in A549 NSCLC cells. Our findings contribute significantly to the field as they shed light on the underexplored aspects of Coix and its active ingredients.
Although there have been reports on Coix fingerprints in the research literature covering medicinal and edible plants and food crops, the methodologies used in these studies vary significantly. As a result, current information lacks regular and systematic data and does not adequately correspond to the nutritional and physiological efficacy of Coix. Consequently, this information is not reliable technical support for quality monitoring. The Chinese Pharmacopoeia recommends triglyceride as a quality control indicator for Coix, Coix seed, and Coix seed oil. However, considering the remarkable anticancer effect of coixol demonstrated in our study, we propose that coixol could be a promising candidate as a new quality control indicator for Coix, Coix seed, Coix seed oil, and their preparations.
Conclusions
The current study successfully synthesized a stable and water-soluble coixol-CDP inclusion compound, which exhibited enhanced anticancer effects compared to coixol alone. These results provide valuable information about the applications of coixol and might pave the way for the investigations of other active components of Coix.
Authors’ contributions
X.C.W., S.Q.D., and B.P. contributed to the study conception and design. X.C.W., X.Y.S., L.C., and R.W. performed compound synthesis and identification. X.C.W., X.Y.S., M.Y.W., C.H.G., and R.R.X. performed cell culture and Western blots. X.C.W., X.Y.S., S.Q.D., and B.P. analyzed and interpreted the data. X.C.W., X.Y.S., S.Q.D., and B.P. wrote the first draft of the manuscript. All authors contributed to the manuscript revision and approved the submitted version.
Disclosure statement
No potential conflict of interest was reported by the authors.
Data availability statement
The datasets used and/or analyzed are available from the corresponding author upon reasonable request.
Additional information
Funding
References
- Ali A, Haq FU, Ul Arfeen Q, Sharma KR, Adhikari A, Musharraf SG. 2017. Sensitive quantification of coixol, a potent insulin secretagogue, in Scoparia dulcis extract using high-performance liquid chromatography combined with tandem mass spectrometry and UV detection. Biomed Chromatogr. 31:e3964.
- Amiri S, Nalbandi B. 2018. Improve solubility and bioavailability of silver sulfadiazine via formation of inclusion complex by cyclodextrin. J Inorg Organomet Polym. 28(5):1764–1774. doi: 10.1007/s10904-018-0809-8.
- Chung CP, Hsu CY, Lin JH, Kuo YH, Chiang W, Lin YL. 2011. Antiproliferative lactams and spiroenone from adlay bran in human breast cancer cell lines. J Agric Food Chem. 59(4):1185–1194. doi: 10.1021/jf104088x.
- Delogu G, Juliano CCA, Usai M. 2016. Thymus catharinae Camarda essential oil: beta-cyclodextrin inclusion complexes, evaluation of antimicrobial activity. Nat Prod Res. 30(18):2049–2057. doi: 10.1080/14786419.2015.1107558.
- di Cagno M, Terndrup Nielsen T, Lambertsen Larsen K, Kuntsche J, Bauer-Brandl A. 2014. beta-Cyclodextrin-dextran polymers for the solubilization of poorly soluble drugs. Int J Pharm. 468(1-2):258–263. doi: 10.1016/j.ijpharm.2014.04.029.
- Gidwani B, Vyas A. 2014. Synthesis, characterization and application of epichlorohydrin-beta-cyclodextrin polymer. Colloids Surf B Biointerfaces. 114:130–137. doi: 10.1016/j.colsurfb.2013.09.035.
- Hameed A, Hafizur RM, Khan MI, Jawed A, Wang H, Zhao M, Matsunaga K, Izumi T, Siddiqui S, Khan F, et al. 2019. Coixol amplifies glucose-stimulated insulin secretion via cAMP mediated signaling pathway. Eur J Pharmacol. 858:172514. doi: 10.1016/j.ejphar.2019.172514.
- Hsu HY, Lin BF, Lin JY, Kuo CC, Chiang W. 2003. Suppression of allergic reactions by dehulled adlay in association with the balance of TH1/TH2 cell responses. J Agric Food Chem. 51(13):3763–3769. doi: 10.1021/jf021154w.
- Igbokwe CJ, Wei M, Feng Y, Duan Y, Ma H, Zhang H. 2021. Coix seed: a review of its physicochemical composition, bioactivity, processing, application, functionality, and safety aspects. Food Rev Inter. 38(sup1):921–939. doi: 10.1080/87559129.2021.1892129.
- Jiang LL, Miao MS. 2014. Cogongrass rhizome chemistry, pharmacology and clinical application. China J Chinese Med. 29:9448–9453.
- Latha M, Pari L, Ramkumar KM, Rajaguru P, Suresh T, Dhanabal T, Sitasawad S, Bhonde R. 2009. Antidiabetic effects of scoparic acid D isolated from Scoparia dulcis in rats with streptozotocin-induced diabetes. Nat Prod Res. 23(16):1528–1540. doi: 10.1080/14786410902726126.
- Lee HJ, Ryu J, Park SH, Seo EK, Han AR, Lee SK, Kim YS, Hong JH, Seok JH, Lee CJ. 2015. Suppressive effects of coixol, glyceryl trilinoleate and natural products derived from Coix Lachryma-Jobi var. ma-yuen on gene expression, production and secretion of airway MUC5AC mucin. Arch Pharm Res. 38(5):620–627. doi: 10.1007/s12272-014-0377-6.
- Liu J, Ding X, Fu Y, Xiang C, Yuan Y, Zhang Y, Yu P. 2021. Cyclodextrins based delivery systems for macro biomolecules. Eur J Med Chem. 212:113105. doi: 10.1016/j.ejmech.2020.113105.
- Mikhail N, Lin TK, Mohd ZNI, Abd HSB. 2018. Treatment of cotton by β-cyclodextrin/triclosan inclusion complex and factors affecting antimicrobial properties. Fibers Polymers. 19:548–560.
- Morillo E, Sánchez-Trujillo MA, Villaverde J, Madrid F, Undabeytia T. 2014. Effect of contact time and the use of hydroxypropyl-beta-cyclodextrin in the removal of fluorene and fluoranthene from contaminated soils. Sci Total Environ. 496:144–154. doi: 10.1016/j.scitotenv.2014.07.027.
- Morin-Crini N, Crini G. 2013. Environmental applications of water-insoluble β-cyclodextrin-epichlorohydrin polymers. Prog Polymer Sci. 38(2):344–368. doi: 10.1016/j.progpolymsci.2012.06.005.
- Pan B, Deng C. 2019. Modulation by chronic antipsychotic administration of PKA- and GSK3beta-mediated pathways and the NMDA receptor in rat ventral midbrain. Psychopharmacology (Berl). 236(9):2687–2697. doi: 10.1007/s00213-019-05243-x.
- Pan B, Han B, Zhu X, Wang Y, Ji H, Weng J, Liu Y. 2023a. Dysfunctional microRNA-144-3p/ZBTB20/ERK/CREB1 signalling pathway is associated with MK-801-induced schizophrenia-like abnormalities. Brain Res. 1798:148153. doi: 10.1016/j.brainres.2022.148153.
- Pan B, Lian J, Deng C. 2018. Chronic antipsychotic treatment differentially modulates protein kinase A- and glycogen synthase kinase 3 beta-dependent signaling pathways, N-methyl-d-aspartate receptor and gamma-aminobutyric acid A receptors in nucleus accumbens of juvenile rats. J Psychopharmacol. 32(11):1252–1263. doi: 10.1177/0269881118788822.
- Pan B, Lian J, Huang XF, Deng C. 2016. Aripiprazole increases the PKA signalling and expression of the GABAA receptor and CREB1 in the nucleus accumbens of rats. J Mol Neurosci. 59(1):36–47. doi: 10.1007/s12031-016-0730-y.
- Pan B, Wang Y, Shi Y, Yang Q, Han B, Zhu X, Liu Y. 2022a. Altered expression levels of miR-144-3p and ATP1B2 are associated with schizophrenia. World J Biol Psychiatry. 23(9):666–676. doi: 10.1080/15622975.2021.2022757.
- Pan B, Xu L, Weng J, Wang Y, Ji H, Han B, Zhu X, Liu Y. 2022b. Effects of icariin on alleviating schizophrenia-like symptoms by regulating the miR-144-3p/ATP1B2/mTOR signalling pathway. Neurosci Lett. 791:136918. doi: 10.1016/j.neulet.2022.136918.
- Pan B, Zhu X, Han B, Weng J, Wang Y, Liu Y. 2023b. The SIK1/CRTC2/CREB1 and TWIST1/PI3K/Akt/GSK3beta signaling pathways mediated by microRNA-25-3p are altered in the schizophrenic rat brain. Front Cell Neurosci. 17:1087335. doi: 10.3389/fncel.2023.1087335.
- Priyanga S, Khamrang T, Velusamy M, Karthi S, Ashokkumar B, Mayilmurugan R. 2019. Coordination geometry-induced optical imaging of L-cysteine in cancer cells using imidazopyridine-based copper (II) complexes. Dalton Trans. 48(4):1489–1503. doi: 10.1039/c8dt04634d.
- Rahayu RP, Sularsih S. 2019. Cytotoxicity of combination chitosan with different molecular weight and ethanol extracted Aloe vera using MTT assay. IOP Conf. Ser.: Earth Environ. Sci.217:012030. doi: 10.1088/1755-1315/217/1/012030.
- Sharma KR, Adhikari A, Hafizur RM, Hameed A, Raza SA, Kalauni SK, Miyazaki J, Choudhary MI. 2015. Potent insulin secretagogue from Scoparia dulcis Linn of Nepalese origin. Phytother Res. 29(10):1672–1675. doi: 10.1002/ptr.5412.
- Song HY, Deng XH, Hou XF, Yuan GY, Zhu ZD, Ren MX. 2014. Effect of ECRG2 in combination with cisplatin on the proliferation and apoptosis of EC9706 cells. Exp Ther Med. 8(5):1484–1488. doi: 10.3892/etm.2014.1972.
- Suzuki Y, Konaya Y. 2021. Coix seed may affect human immune function. Nat Prod Commun. 16(10):1934578X2110486. doi: 10.1177/1934578X211048642.
- Tan MM, Chen ZL, Xiong SB, Zhao SM, Xue BY. 2017. Analysis on nutritional properties of Adlay (Coixl acryma-jobi L.) seeds. J Chinese Cereals Oils Assoc. 32:43–48 + 55.
- Tian HX, Zheng XX, Die HU, Jian XU. 2017. Research progress on chemical components and quality control of Coix seed. Guizhou Agric Sci. 45:82–87.
- Upadhyay SK, Ali SM. 2018. Molecular recognition of flunarizine dihydrochloride and beta-cyclodextrin inclusion complex by NMR and computational approaches. Chem Cent J. 12:33.
- Wang HY, Lian N, Gao LP. 2017. Effect of oligomeric proanthocyanidins from grape seeds combined with cisplatin on A549 cell proliferation and cell cycle. Food Sci. 38:213–218.
- Xu HN, Liu YM. 2018. Synergistic antioxidant effect of hexahydro-β-acid-cyclodextrin inclusion complex and food additives. Food Sci. 39:33–40.
- Xu RR, Gao YY, Zhou YL, Hu R. 2017a. Preparation and characterization of Adlay seed oil and β-cyclodextrin inclusion complexes. Sci Tech Food Ind. 38:258–263.
- Xu RR, Hu R, Chen L, Pan B, Wang XC, Wu HH, Song XH. 2017b. Preparation, characterization, toxicity and pharmacodynamics of the inclusion complex of Brucea javanica oil with beta-cyclodextrin polymers. Curr Pharm Biotechnol. 18(10):855–861. doi: 10.2174/1389201019666171211153209.
- Yang YS, Du Y, Sun YQ, Han T, Jia M, Qin LP. 2017. Determination of effective contents triolein and coixol in Coix lacrymajobi var. mayuen from different origins. Chinese Trad Herbal Drugs. 48:578–581.
- Yang ZT, Wu TX, Tang QL, Wei GD, Tang X, Wang JT. 2017. Determination of functional components in coixseed and Poria yellow rice wine. Liquor-Making Sci Technol. 12:122–129.
- Yildiz ZI, Celebioglu A, Kilic ME, Durgun E, Uyar T. 2018. Fast-dissolving carvacrol/cyclodextrin inclusion complex electrospun fibers with enhanced thermal stability, water solubility, and antioxidant activity. J Mater Sci. 53(23):15837–15849. doi: 10.1007/s10853-018-2750-1.
- Zhang YB, He XH, Liu FZ, Meng QY, Liu Y, Shen G. 2018. Screening and analysis of wild Coix germplasm with high Coixin content. J Guizhou Normal Univ (Natural Sci). 36:43–46.
- Zhao C, Li Y, Qiu W, He F, Zhang W, Zhao D, Zhang Z, Zhang E, Ma P, Liu Y, et al. 2018. C5a induces A549 cell proliferation of non-small cell lung cancer via GDF15 gene activation mediated by GCN5-dependent KLF5 acetylation. Oncogene. 37(35):4821–4837.,. doi: 10.1038/s41388-018-0298-9.
- Zhao Y, Jing Z, Lv J, Zhang Z, Lin J, Cao X, Zhao Z, Liu P, Mao W. 2017. Berberine activates caspase-9/cytochrome c-mediated apoptosis to suppress triple-negative breast cancer cells in vitro and in vivo. Biomed Pharmacother. 95:18–24. doi: 10.1016/j.biopha.2017.08.045.
- Zhu G, Gai P, Wu L, Zhang J, Zhang X, Chen J. 2012. beta-Cyclodextrin-platinum nanoparticles/graphene nanohybrids: enhanced sensitivity for electrochemical detection of naphthol isomers. Chem Asian J. 7(4):732–737. doi: 10.1002/asia.201100839.
- Zhu LX, Tang CY, Yu JD, Zhi C, Yang X. 2018. Impact of Coix seed oil on fluorescence excretion pharmacokinetics and protein expression in doxorubicin-resistant cells MCF-7/DOX. Yaoxue Xuebao. 53:84–89.