Abstract
Context
Pileostegia tomentella Hand. Mazz (Saxifragaceae) total coumarins (TCPT) show antitumour activity in colorectal cancer (CRC) with unknown mechanism of action. Tumour angiogenesis mediated by exosomes-derived miRNA exhibits the vital regulation of endothelial cell function in metastasis of CRC.
Objective
To investigate the effect of TCPT on exosomal miRNA expression and angiogenesis of CRC cells.
Materials and methods
HT-29-derived exosomes were generated from human CRC cells (HT-29) or either treated with TCPT (100 μg/mL) for 24 h, followed by identification by transmission electron microscope, nanoparticle tracking analysis (NTA) and Western blot. Co-culture experiments for human umbilical vein endothelial cells (HUVECs) and exosomes were performed to detect the uptake of exosomes in HUVECs and its influence on HUVECs cells migration and lumen formation ability. Potential target miRNAs in exosomes were screened out by sequencing technology. Rescue assays of angiogenesis were performed by the transfecting mimics or inhibitors of targeted miRNA into HUVECs.
Results
HT-29-derived exosomes, after TCPT treatment (Exo-TCPT), inhibited the migration and lumen formation of HUVECs, reduced the expression levels of vascular marker (FLT-1, VCAM-1 and VEGFR-2) in HUVECs. Furthermore, the level of miR-375-3p was significantly upregulated in Exo-TCPT. Rescue assays showed that high expression of miR-375-3p in HUVECs inhibited migration and lumen formation abilities, which was consistent with the effects of Exo-TCPT, whereas applying miR-375-3p inhibitors displayed opposite effects.
Discussion and conclusion
TCPT exhibits anti-angiogenesis in CRC, possibly through upregulating exosomal miR-375-3p. Our findings will shed light on new target exosomes miRNA-mediated tumour microenvironment and the therapeutic application of Pileostegia tomentella in CRC.
Keywords:
Introduction
Colorectal cancer (CRC) is one of the most common malignant tumours in the gastrointestinal tract, and its incidence increases year by year (Dekker et al. Citation2019). The onset of CRC is insidious, with no obvious early symptoms, and most of them have metastasized upon diagnosis. Although current treatment strategies such as radiotherapy, chemotherapy, immunotherapy and combination therapy have made some progress, many patients are confronted with the risk of disease recurrence due to the high biological complexity of the CRC metastasis process. Additionally, patients with metastatic CRC have a poor prognosis, with a median 5-year survival rate of 18.5% in the USA and 27.7% in Europe (Sung, Parent, et al. Citation2021; Sung, Ferlay, et al. Citation2021). Therefore, studying the mechanism of CRC metastasis and finding new methods for effective prevention and treatment of CRC metastasis have become urgent issues to be addressed.
Metastasis of malignant tumours is a multi-dimensional, multi-stage and multi-step sequential regulatory process, among which tumour angiogenesis is the initial step of tumour metastasis formation and the key to the occurrence and development of tumour metastasis. Stimulating tumour angiogenesis can effectively regulate the blood supply of tumour tissue, maintain the stability of the tumour blood supply, and promote the metastasis of tumour cells through vascular access. Therefore, maintaining the functional homeostasis of vascular endothelial cells and inhibiting the activation, proliferation and angiogenesis of endothelial cells has become one of the therapeutic strategies to prevent and treat tumour progression and metastasis.
Exosomes are bilayer vesicles with a diameter of 30–150 nm that are formed by the fusion of intracellular multivesicular bodies with cell membranes and released into the extracellular matrix. Exosomes envelop mRNA, miRNA and proteins, protecting the contents from degradation. Exosomes can target distant or adjacent cells through blood or body fluids or in a paracrine manner to establish cell communication and affect the metastatic characteristics of tumour cells in situ (Ma et al. Citation2021). Blocking exosome-specific intercellular information exchange, reversing, and preventing cellular behaviour changes in the metastatic microenvironment can effectively inhibit tumour invasion and metastasis (Sung, Parent, et al. Citation2021; Sung, Ferlay, et al. Citation2021; Mashouri et al. Citation2019).
Pileostegia tomentella, the whole herb of Pileostegia tomentella Hand. Mazz (Saxifragaceae), is a traditional Yao medicine in Guangxi Zhuang Autonomous Region and is widely used in Yao folk medicine to treat malignancies. In our previous study, we preliminarily revealed the antitumour effect and mechanism of the alcohol extract of Pileostegia tomentella on H22 tumour-bearing mice (Liu et al. Citation2016). Further chemical composition analysis showed that the main chemical components of Pileostegia tomentella were coumarins and glycosides. In vitro antitumour activity screening showed that total coumarins from Pileostegia tomentella (TCPT) were the most active antitumour component (Hu et al. Citation2019), which could significantly inhibit the proliferation, migration and invasion abilities of CRC cells and promote cell apoptosis (Liu et al. Citation2019). However, no relevant studies have been conducted on the anti-angiogenesis and inhibition of tumour metastasis of TCPT.
This study attempts to employ tumour-derived exosomes as an entry point to investigate the effect of TCPT on miRNA expression and angiogenesis of human CRC cell-derived exosomes in vitro, and explore the potential antitumour molecular mechanism of action of TCPT, thus providing a theoretical reference and basis for the treatment of CRC metastasis.
Materials and methods
Preparation of TCPT
TCPT was prepared by our laboratory (Hu et al. Citation2019). Pileostegia tomentella was harvested from Guilin Yaoshan Mountain, Guangxi Zhuang Autonomous Region in June 2019, and identified as the whole herb of Pileostegia tomentella by associate researcher Huang Yun-feng, Department of Traditional Chinese Medicine, Guangxi Institute of Chinese Medicine & Pharmaceutical Science (Voucher specimen: ZLT-20190627). Pileostegia tomentella (250 g) herbs were crushed into coarse powder and extracted by the reflux approach with 95% ethanol for 5 cycles, each taking 2 h. The extraction solutions were filtered, combined and the solvent was recovered to obtain 46 g of residue. The residue was suspended in water and extracted with petroleum ether (60–90 °C) and ethyl acetate successively. The extraction fractions in ethyl acetate were separated by a silica gel column and eluted with an ethyl acetate-methanol gradient (100:0→90:10→80:20→50:50). The eluates were collected and the coumarin components were combined through thin layer chromatography, and finally 6.28 g of TCPT was obtained.
HPLC analysis
A Waters XBridge C18 column (4.6 mm × 250 mm, 5 µm) was used, and the mobile phase consisted of acetonitrile (A)-0.1% phosphoric acid solution (B) with the procedure of gradient elution. For chromatographic separation, the following gradient was used: 0–45 min, 5–40% A; 45–46 min, 40–5% A; 46–60 min, 5% A. The flow rate was 1.0 mL/min, the column temperature was 25 °C, the detection wavelength was 320 nm, and the injected volume was 10 μL. The powdered Pileostegia tomentella extract sample (0.5 g) was mixed with 25 mL of 80% methanol. After ultrasonic treatment for 60 min, the solution was filtered with 0.45 µm microporous filter membrane to obtain the TCPT sample solution.
TCPT was determined by HPLC chromatography to consist of 4 main coumarins (Huang et al. Citation2022): skimmin, 8-methoxycoumarin-7-O-β-d-glucose, 7-hydroxycoumarin and 7-hydroxy-8-methoxycoumarin (). The total content of coumarins in TCPT was 65.43% when using 7-hydroxycoumarin as the standard control (Hu et al. Citation2019).
Figure 1. HPLC chromatograms of mixed reference solution and TCPT sample solution. (A) HPLC chromatogram of mixed reference solution. (B) HPLC chromatogram of TCPT sample solution. Notes: peak 1, skimmin; peak 2, 8-methoxycoumarin-7-O-β-d-glucose; peak 3, 7-hydroxycoumarin; peak 4, 7-hydroxy-8-methoxycoumarin.
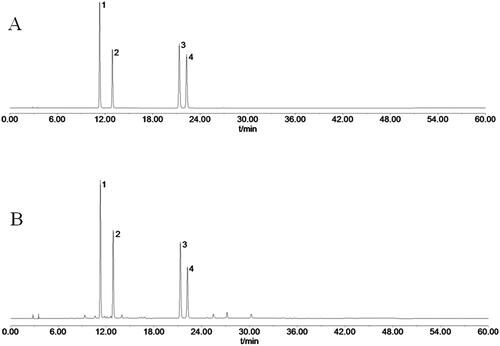
Cells
Human CRC cells HT-29 and human umbilical vein endothelial cells (HUVECs) were purchased from Guangzhou Suyan Biotechnology Co., Ltd. (Guangzhou, China) and were identified by the short tandem repeat (STR) profiling method.
Isolation and identification of exosomes
The human CRC cell line HT-29 was routinely cultured and passaged in our laboratory. At the logarithmic growth stage, HT-29 cells were divided into the control group and the TCPT group. A 100 μg/mL final TCPT concentration was added to the TCPT group. The concentration was set based on a previous study, where the IC50 of TCPT on HT-29 cells was found to be 89.77 μg/mL (Hu et al. Citation2019). In the control group, the same volume of cell medium was added. The cell supernatant was collected after culture for 24 h. Then it was centrifuged at 2000 g for 10 min at 4 °C, 10,000 g for 30 min at 4 °C and 100,000 g for 70 min at 4 °C. Subsequently, the transparent precipitate was taken and resuspended with 400 μL PBS to form an exosome solution, which was stored at −80 °C after partitioning. The characteristic morphology of exosomes was observed by transmission electron microscope (Tecnai G2 Spirit, FEI, Hillsboro, OR); the particle size of exosomes was detected by nanoparticle tracking analyzer (PMX 110, Metrix, Meerbusch, Germany) and the expressions of surface marker proteins CD9 and TSG101 were detected by Western blot.
Exosome tracing
HT-29 exosome (20 μg) was added to 1 mL 20 nmol lipophilic dye Dil (bath No.2161852; Thermo Fisher, Waltham, MA). The mixture was incubated at 37 °C for 30 min to label the exosomes. HUVEC cells at the logarithmic growth stage were inoculated into a 35 mm glass dish with 3 × 105 cells per dish. After 24 h of cell adherent growth, HT-29-exosome (20 μg/mL) labelled Dil was added. The supernatant was removed after a 24 h co-culture. The cells were washed with PBS, fixed with 4% paraformaldehyde for 15 min and then incubated with DAPI in the dark for 5 min. After being washed with PBS, the exosome uptake of HUVEC cells was observed by a fluorescence microscope (DMi8, Leica, Wetzlar, Germany).
Western blot analysis
Total protein of the cells was extracted by RIPA lysate (Sigma, Beijing Brand, China) and the protein concentration was determined by bicinchoninic acid (BCA) protein assay kit (Beyotime, Shanghai, China). Then 20 μg of total protein was taken for SDS-PAGE on 12% separating gel and 5% stacking gel, and transferred onto PVDF membranes. After blocking with PBST containing 5% skim milk for 1 h, the membranes were incubated overnight at 4 °C with mouse anti-FLT-1 (Proteintech, Wuhan, China, 13687-1-AP, 1:1000), rabbit anti-VCAM-1 (Abclonal, Wuhan, China, A0279, 1:500), rabbit anti-VEGFR-2 (Abcam, Cambridge, UK, ab134191, 1:1000) and mouse anti-GAPDH (Abcam, Cambridge, UK, ab8245, 1:5000), respectively, followed by washing three times with PBST. Then, the membranes were incubated with HRP-labelled goat anti-rabbit IgG or anti-mouse IgG (Proteintech, Wuhan, China, HRP-66467, HRP-67761, 1:10,000) for 2 h. After three washes with PBST, the membranes were immersed in ECL multifunctional substrate for 1 min, and the images were captured by the multifunctional imaging analysis system (FluorChem R, Protein simple, Silicon Valley, CA).
Exosome miRNA sequencing
Exosome miRNA sequencing was performed at Heyuan Biotechnology (Shanghai, China) Co., Ltd. Total RNA was extracted from exosome samples. The RNA of 18–30 nt was isolated, and 3′ and 5′ adaptors were ligated. CDNA was synthesized by reverse transcription extension. The cDNA library was constructed and purified by PCR and gel electrophoresis. The constructed cDNA library was sequenced using Illumina Hiseq 2000/2500 with a single-end 1 × 50 bp read length.
MiR-375-3p transfection experiment
Mimics and inhibitors of hsa-miR-375-3p were synthesized by Guangdong Ruibo Biotechnology Co., Ltd. (Guangdong, China). HUVEC cells in logarithmic growth stage were seeded in 6-well plates with 4 × 105 cells/well. After 24 h of adherent growth, the medium was removed and the cells washed twice with PBS. Lipofectamine 2000, miR-375-3p mimic and inhibitor were diluted, respectively, according to miRNA product instructions. They were added to the well plates and cultured in a CO2 incubator for 48 h.
Detection of miR-375-3p expression by qRT-PCR
The RNA purity and concentration were detected by a micronucleic acid analyzer (Nanodrop One, Thermo Fisher, Waltham, MA) after total RNA had been extracted by the Trizol method. CDNA synthesis was done using the Mir-X™ miRNA First-Strand Synthesis Kit (bath No.1908A55A; TaKaRa, Beijing Brand, China). The expression of miR-375-3p was measured with TB Green® Premix Ex Taq™ II (Tli RNaseH Plus) (bath No.ALE1615A; TaKaRa, Beijing Brand, China) and the following primers: miR-375-3p (5′-ATGTTCGGCTCGCGTGAA-3′) and U6 (forward: 5′-GGAACGATACAGAGAAGATTAGC-3′; reverse: 5′-TGGAACGCTTCACGAATTTGCG-5′). The relative expression of miR-375-3p was normalized to that of U6 using the 2−△△CT method.
Transwell cell migration assay
HUVEC cells were resuspended in serum-free medium, adjusted to 5 × 105 cells/mL, and inoculated into the upper chamber of the Transwell chamber at 0.2 mL/well for the control group and the treatment group. The lower chamber was filled with a medium containing 10% serum. After 24 h of culture in the incubator, the chamber was taken out and washed with PBS for 2–3 times. The residual cells on the upper layer of the microporous filter membrane were wiped with a cotton swab. The cells on the lower layer of the membrane were stained with 0.1% crystal violet for 30 min, rinsed with running water, dried and photographed using a light microscope after being fixed with 4% paraformaldehyde for 15 min. The membrane was decolorized with 3% acetic acid, and the absorbance (OD value) of the decolorized solution was detected by a microplate reader at a wavelength of 570 nm.
In vitro lumen formation assay
Lumen formation levels were assessed by using the Angiogenesis Assay (in vitro) Kit following the manufacturer’s protocol (bath No. ab204726; Abcam, Cambridge, UK). The precooled Matrigel matrix was added to 96-well plate at 50 μL/well, and was placed in a cell incubator for 1 h to coagulate. The matrix was supplemented with 100 µL of HUVEC cells suspension (8 × 104 cells/mL) in various treatment groups. Suramin was added to the positive group (10 μmol/L) while medium of the same volume was added to the negative control group. The medium was removed from the plates after a 4 h culture and the plate was washed with PBS before calcein AM dye was added. Thirty minutes later, images were examined and captured on camera using a fluorescent microscope to determine the lumen length and number of nodes.
Statistical analysis
Data were expressed as mean ± standard deviation and statistical analysis was performed using SPSS version 22.0 software(SPSS Inc., Chicago, IL). The comparison between two groups was performed by t-test, and the comparison between multiple groups was performed by one-way analysis of variance (ANOVA). When the total variance was p > 0.05, the LSD-t-test was used, and the data was properly converted when the normal condition was not satisfied.
Results
Identification and tracking of Exo-TCPT
To investigate the role of exosomes in endothelial cell angiogenesis, the exosomes from CRC cells HT-29 before and after TCPT treatment were isolated, and characterized using electron microscopy, nanoparticle tracking analysis (NTA) and Western blot. No significant differences in morphology were observed between the TCPT group and the control group when the typical biconcave cystic vesicles of exosomes were examined under an electron microscope in the supernatant of HT-29 cells (). Results of the NTA test showed that the average particle sizes of exosomes in the TCPT group and control group were 139.6 and 135.1 nm, respectively, with no significant difference between the two groups, which meet the criteria for particle size of exosomes (). Exosomes from both the TCPT group and the control group had positive expression of the membrane marker proteins CD9 and TSG101, according to a Western blot analysis (). Exosomes target and regulate the functions of distant or adjacent cells by delivering a variety of biomolecules. To determine whether HT-29-derived exosomes functioned inside HUVEC cells, we tracked the exosomes by incubating HUVEC cells with fluorescent dye (Dil)-labelled exosomes. The results showed that HUVECs nucleus (blue) was surrounded by exosomes (red), indicating that exosomes could be effectively taken up by HUVECs ().
Figure 2. Identification and tracking of exosomes. (A) Morphological characteristics of exosomes were observed under electron microscopy (Bar = 100 nm). (B) The particle size distribution of exosomes was analysed by nanoparticle tracking analysis (NTA). (C) The expression of exosome marker proteins (CD9, TSG101) was detected by Western blot. (D) Dil-labelled exosomes were taken up by HUVECs; Bar = 25 μm.
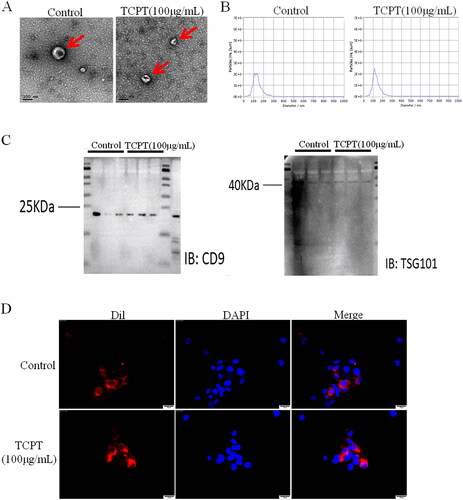
Exo-TCPT inhibits angiogenesis in HUVECs
To explore whether TCPT affects angiogenesis through exosome, we collected exosomes from TCPT-treated HT-29 (Exo-TCPT) and detected their effect on the angiogenesis of endothelial cells, HUVECs. Results of Matrigel lumen formation experiments showed that, compared with the blank control group, the lumen formation ability of HUVECs in the TCPT group and the normal exosomes control group (Exo-Control) did not change significantly, while the TCPT-treated HT-29 exosomes group (Exo-TCPT (100 μg/L)) significantly inhibited angiogenesis of HUVECs, which was manifested by the reduction in the lumen formation length and the number of nodes of HUVECs (). Consistent results were found in the Transwell chamber experiments, where Exo-TCPT effectively inhibited the migration capacity of HUVECs (). Western blot assay showed that Exo-TCPT significantly reduced the expression levels of vascular marker proteins FLT-1, VCAM-1 and VEGFR-2 in HUVECs (). These findings suggest that TCPT exerts its inhibitory effect on angiogenesis through exosomes from HT-29.
Figure 3. Exo-TCPT inhibits the angiogenic activity of HUVECs. (A) Images of lumen formation in each treatment group, Bar = 250 μm. (B) The length of lumen formation and quantitative data of nodes were analysed by ImageJ (n = 3), **p < 0.01 vs. control. (C) Images of Transwell chamber experiments in each treatment group, Bar = 200 μm. (D) Quantitative data analysis of the OD value of HUVEC migrating cells (n = 3), **p < 0.01 vs. control. (E) The protein levels of FLT-1, VCAM-1, VEGFR-2 and GAPDH were determined by Western blot. (F) The relative density was analysed by protein simple analysis software, **p < 0.01 vs. control.
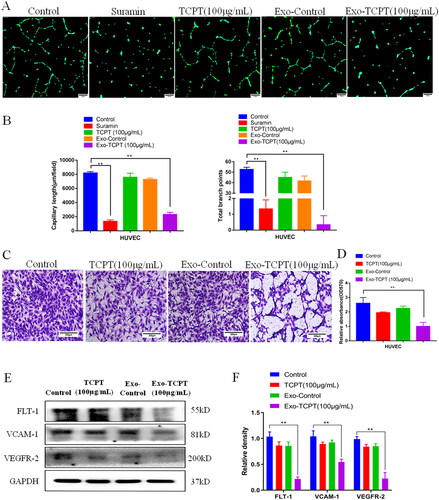
MicroRNA expression profile of Exo-TCPT
MicroRNA in exosomes is an important factor in regulating intercellular communication. We used miRNA-Seq to analyse the difference in miRNA expression profiles of exosomes before and after TCPT treatment of HT-29. Among the differentially expressed miRNAs, a total of 9 miRNAs showed significant differences in expression abundance (fold change >2, p < 0.05), including 2 up-regulated miRNAs and 7 down-regulated miRNAs. The expression level of hsa-miR-375-3p was significantly increased (). Target genes of differential miRNAs were predicted, and KEGG pathway enrichment analysis was performed. These target genes were found to be enriched in signalling pathways related to angiogenesis, including: the PI3K-AKT signalling pathway, the Ras signalling pathway, the Hippo signalling pathway and the EGFR signalling pathway (), and hsa-miR-375-3p is involved in these signalling pathways.
Figure 4. Changes in exosome miRNA expression profiles caused by TCPT. (A) Volcano map of differential miRNAs before and after TCPT treatment. Red, significantly upregulated miRNAs in the exosome; blue, significantly down-regulated miRNAs in the exosome; grey, no significant difference. (B) Cluster analysis of differential miRNAs. Compared with the control group, the expression abundance of hsa-miR-375-3p in the exosome of the TCPT treatment group was significantly increased. (C) KEGG pathway analysis of differential miRNA target genes, showing the top 14 enriched signalling pathways.
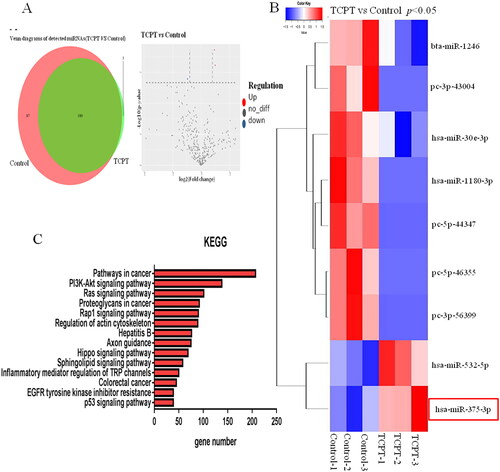
Exo-TCPT inhibits the angiogenic activity of HUVECs mediated by miR-375-3P
To clarify the inhibitory activity of miR-375-3P in Exo-TCPT on the angiogenesis of HUVECs, we conducted miRNA overexpression and inhibition verification experiments. The results showed that, compared with the blank control group, the expression of miR-375-3P in exosomes was significantly increased after HT-29 cells were treated with TCPT (). In addition, the expression of miR-375-3P in HUVECs treated with Exo-TCPT was significantly increased (), indicating that the increased expression of miR-375-3p in HUVECs was mediated by exosomes. We constructed a transfection system and transfected a synthetic miR-375-3p mimic and inhibitor into HUVEC cells () to further validate the effect of miR-375-3p on angiogenic activity. The results showed that, compared with the blank control group, miR-375-3p mimic could significantly inhibited the angiogenic activity of HUVECs. On the contrary, transfection with miR-375-3p inhibitor had the opposite result, which could significantly enhance the lumen formation ability () and migration ability of HUVECs (). These results suggested that Exo-TCPT inhibited the angiogenic activity of HUVECs via miR-375-3P.
Figure 5. Exo-TCPT inhibited the angiogenic activity of HUVECs by miR-375-3P. (A) Expression of miR-375-3P in exosomes of HT-29 cells before and after TCPT treatment (n = 3), **p < 0.01 vs. control. (B) Expression of miR-375-3P in HUVECs was detected by qRT-PCR after Exo-NC and Exo-TCPT treatment (n = 3), **p < 0.01 vs. control. (C) Mimics and inhibitors of miR-375-3p were synthesized, and after transfection with HUVECs, the expression of miR-375-3p was detected (n = 3), **p < 0.01 vs. control. (D) Images of lumen formation in each group after transfection of miR-375-3p mimic and inhibitor, Bar = 250 μm. (E) The length of lumen formation and quantitative data of nodes were analysed by ImageJ (n = 3), **p < 0.01 vs. control. (F) Images of Transwell chamber experiments of miR-375-3p mimic and inhibitor, Bar = 200 μm. (G) Quantitative data analysis of the OD value of HUVEC migrating cells (n = 3), *p < 0.05, **p < 0.01 – control.
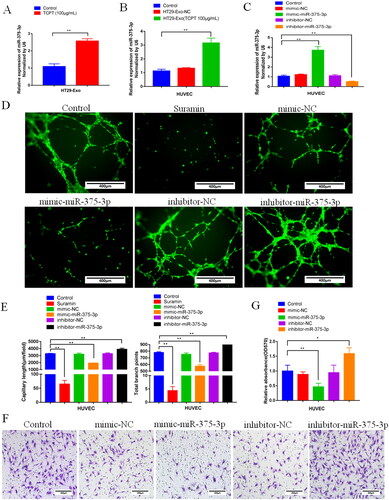
Discussion
Pileostegia tomentella, the vine stem of Pileostegia tomentella, is a traditional Yao medicine in Guangxi Zhuang Autonomous Region. It has the functions of dispersing blood stasis, alleviating pain, reducing swelling and having detoxifying effects. It is widely used in folk medicine to treat malignancies. TCPT is the main antitumour active site of Pileostegia tomentella, and our previous studies have found that TCPT can significantly inhibit the proliferation, migration, and invasion abilities of CRC cells and promote apoptosis (Hu et al. Citation2019; Liu et al. Citation2019). However, the mechanism by which TCPT works to combat CRC has not been thoroughly investigated.
In recent years, exosomes have gained a lot of attention in the field of antitumour metastasis research. They are double-layered membrane vesicles released from intracellular multivesicular bodies into the extracellular matrix with a diameter of around 30–150 nm that encapsulate mRNA, miRNA and proteins. Exosomes target distant or adjacent cells through blood, body fluids or paracrine signals, which is an important way of intercellular signal communication (Kahlert and Kalluri Citation2013). The regulation of tumour angiogenesis by circulating exosome-mediated miRNA is currently a potentially new target for antitumour metastasis. Under the action of inducing factors, the exosome miRNAs secreted by tumour cells can target vascular endothelial cells, participate in the regulation of downstream target gene expression, affect the function of endothelial cells and ultimately affect tumour angiogenesis and metastasis (Zhou et al. Citation2014; Zhuang et al. Citation2012).
In this study, exosomes in CRC cells were chosen as the entry point to investigate how TCPT affected exosomes miRNA expression and angiogenesis. Exosomes were extracted by ultracentrifugation, the shapes of the exosomes were observed by transmission electron microscopy, the particle sizes of the exosomes were analysed by NTA, and the expression of the exosome marker protein was detected by Western blot. The extract was confirmed as exosomes. Immunofluorescence was used to label and trace the exosomes, which proved that the exosomes secreted by CRC cells could be successfully taken up by HUVECs. Exosomes from TCPT-treated HT-29 cells were found to effectively inhibit the migration and lumen formation of HUVECs. However, there was no obvious effect of TCPT alone on HUVECs. These indicated that angiogenesis inhibition by TCPT was mediated by exosomes derived from HT-29.
Following TCPT treatment, an apparent different expression profile for miRNAs, such as miR-375-3p, in the exosome of HT-29 cells was determined by miRNA-Seq. MiR-375-3p is considered to maintain the integrity of vascular endothelial barrier function (Mao et al. Citation2021), inhibit tumour cell proliferation and cell cycle progression, and promote tumour cell apoptosis (Gyvyte et al. Citation2020; Chang et al. Citation2020). As an important tumour suppressor, miR-375-3p has high clinical value in the early diagnosis of tumours. It has been reported that miR-375-3p can inhibit tumour growth, metastasis and epithelial-mesenchymal transformation, thus inhibiting the malignant progression of tumours (Li et al. Citation2020; Guo et al. Citation2019). In addition, miR-375-3p can also effectively reverse tumour resistance to chemotherapy drugs (Xu et al. Citation2020). Since miR-375-3p is significantly under expressed in CRC, it is considered a potential target for CRC treatment.
The expression of miR-375-3p in the exosome of TCPT-treated HT-29 cells was significantly increased, as shown by qRT-PCR. To further clarify the role of miR-375-3p in regulating angiogenesis, we constructed a miR-375-3p transfection model and transfected miR-375-3p mimics and inhibitors into HUVECs in vitro. The biological effects of the miR-375-3p mimic were found to be consistent with those of Exo-TCPT and could significantly inhibit migration and lumen formation of HUVECs. In contract, the migration and lumen formation abilities of HUVECs were significantly increased after transfection with miR-375-3p inhibitors. It can be inferred that TCPT suppressed angiogenesis probably by upregulating miR-375-3p via exosomes in HT-29 cells. However, the specific molecular mechanisms for exosome secretion as well as upstream and downstream regulation of miR-375-3p remain to be further studied. In addition, based on the results of exosome miRNA-Seq screening, other alternative differential miRNAs should be mined and verified in the future, which may provide theoretical support for further investigation of the function of miRNAs in CRC-derived exosomes.
Conclusions
The present work provides evidence that TCPT up-regulates the expression of miR-375-3p in CRC-derived exosomes. Exosomes secreted by CRC cells containing miR-375-3p can be absorbed by HUVECs. Exosomal miR-375-3p can regulate the function of endothelial cells, inhibit tumour angiogenesis and metastasis. However, how TCPT regulates the Exo-miR-375-3p remains to be elucidated. Our next experiments will continue to explore how TCPT acts on Exo-miRNA to antitumour, with the aim of providing new ideas and strategies for the mechanism research of the antitumour effect of traditional Chinese medicine and ethnic medicine.
Disclosure statement
The authors report there are no competing interests to declare.
Data availability statement
All data and related information were included in this published paper, which could be acquired in the figures. Data of this study are available by the authors, without undue reservation.
Additional information
Funding
References
- Chang K, Wei Z, Cao H. 2020. miR-375-3p inhibits the progression of laryngeal squamous cell carcinoma by targeting hepatocyte nuclear factor-1β. Oncol Lett. 20(4):80–81. doi: 10.3892/ol.2020.11941.
- Dekker E, Tanis PJ, Vleugels JLA, Kasi PM, Wallace MB. 2019. Colorectal cancer. Lancet. 394(10207):1467–1480. doi: 10.1016/S0140-6736(19)32319-0.
- Guo F, Gao Y, Sui G, Jiao D, Sun L, Fu Q, Jin C. 2019. miR-375-3p/YWHAZ/β-catenin axis regulates migration, invasion, EMT in gastric cancer cells. Clin Exp Pharmacol Physiol. 46(2):144–152. doi: 10.1111/1440-1681.13047.
- Gyvyte U, Lukosevicius R, Inciuraite R, Streleckiene G, Gudoityte G, Bekampyte J, Valentini S, Salteniene V, Ruzgys P, Satkauskas S, et al. 2020. The role of miR-375-3p and miR-200b-3p in gastrointestinal stromal tumors. Int J Mol Sci. 21(14):5151–5162. doi: 10.3390/ijms21145151.
- Hu X, Liu Y, Huang J, Huang Z, Lu G. 2019. Screening of the anti-tumor extracts in Pileostegia tomentella Hand. Mazz (Xiaoliuteng). Pharmacol Clin Chin Mater Med. 35:52–56. Chinese.
- Huang J, Huang Z, Hu X, Tan X, Lu G, Liu Y, Li L. 2022. Determination of the contents of four coumarins in Yao medicine Pileostegia tomentella. J Chin Med Mater. 45:917–921. Chinese.
- Kahlert C, Kalluri R. 2013. Exosomes in tumor microenvironment influence cancer progression and metastasis. J Mol Med (Berl). 91(4):431–437. doi: 10.1007/s00109-013-1020-6.
- Li Q, Huyan T, Cai S, Huang Q, Zhang M, Peng H, Zhang Y, Liu N, Zhang W. 2020. The role of exosomal miR-375-3p: a potential suppressor in bladder cancer via the Wnt/β-catenin pathway. Faseb J. 34(9):12177–12196. doi: 10.1096/fj.202000347R.
- Liu Y, Lu G, Hu X, Huang J, Wang L, Lü J. 2019. Potential anti-tumor mechanisms of total coumarins from Pileostegia tomentella based on network pharmacology. Pharmacol Clin Chin Mater Med. 35:57–63. Chinese.
- Liu Y, Lu G, Wang L, Lu W, Huang Z, Hu X. 2016. Antitumor effect of alcohol extract of Pileostegia tomentella on H22 tumor-bearing mice. Chin Pharm J. 51:981–984. Chinese.
- Ma Z, Wei K, Yang F, Guo Z, Pan C, He Y, Wang J, Li Z, Chen L, Chen Y, et al. 2021. Tumor-derived exosomal miR-3157-3p promotes angiogenesis, vascular permeability and metastasis by targeting TIMP/KLF2 in non-small cell lung cancer. Cell Death Dis. 12(9):840–852. doi: 10.1038/s41419-021-04037-4.
- Mao S, Zheng S, Lu Z, Wang X, Wang Y, Zhang G, Xu H, Huang J, Lei Y, Liu C, et al. 2021. Exosomal miR-375-3p breaks vascular barrier and promotes small cell lung cancer metastasis by targeting claudin-1. Transl Lung Cancer Res. 10(7):3155–3172. doi: 10.21037/tlcr-21-356.
- Mashouri L, Yousefi H, Aref AR, Ahadi AM, Molaei F, Alahari SK. 2019. Exosomes: composition, biogenesis, and mechanisms in cancer metastasis and drug resistance. Mol Cancer. 18(1):75–88. doi: 10.1186/s12943-019-0991-5.
- Sung BH, Parent CA, Weaver AM. 2021. Extracellular vesicles: critical players during cell migration. Dev Cell. 56(13):1861–1874. doi: 10.1016/j.devcel.2021.03.020.
- Sung H, Ferlay J, Siegel RL, Laversanne M, Soerjomataram I, Jemal A, Bray F. 2021. Global cancer statistics 2020: GLOBOCAN estimates of incidence and mortality worldwide for 36 cancers in 185 countries. CA Cancer J Clin. 71(3):209–249. doi: 10.3322/caac.21660.
- Xu F, Ye ML, Zhang YP, Li WJ, Li MT, Wang HZ, Qiu X, Xu Y, Yin JW, Hu Q, et al. 2020. MicroRNA-375-3p enhances chemosensitivity to 5-fluorouracil by targeting thymidylate synthase in colorectal cancer. Cancer Sci. 111(5):1528–1541. doi: 10.1111/cas.14356.
- Zhou W, Fong MY, Min Y, Somlo G, Liu L, Palomares MR, Yu Y, Chow A, O’Connor ST, Chin AR, et al. 2014. Cancer-secreted miR-105 destroys vascular endothelial barriers to promote metastasis. Cancer Cell. 25(4):501–515. doi: 10.1016/j.ccr.2014.03.007.
- Zhuang G, Wu X, Jiang Z, Kasman I, Yao J, Guan Y, Oeh J, Modrusan Z, Bais C, Sampath D, et al. 2012. Tumour-secreted miR-9 promotes endothelial cell migration and angiogenesis by activating the JAK-STAT pathway. EMBO J. 31(17):3513–3523. doi: 10.1038/emboj.2012.183.