Abstract
Context
The therapeutic potential of andrographolide is hindered by its poor oral bioavailability and unpredictable pharmacokinetics, primarily due to its limited water solubility.
Objective
This work aimed to enhance the solubility and pharmacokinetics of andrographolide, a bioactive compound in Andrographis paniculata (Burm. f.) Nees (Acanthaceae), using solubilizing agents and a bioenhancer.
Materials and methods
Four groups of beagles were compared: (1) A. paniculata powder alone (control), (2) A. paniculata powder with 50% weight/weight (w/w) β-cyclodextrin solubilizer, (3) A. paniculata powder with 1% w/w sodium dodecyl sulfate (SDS) solubilizer, and (4) A. paniculata powder co-administered with 1% w/w SDS solubilizer and 10% piperine bioenhancer. All groups received a consistent oral dose of 3 mg/kg of andrographolide, administered both as a single dose and multiple doses over seven consecutive days.
Results
Thirteen chemical compounds were identified in A. paniculata powder, including 7 diterpenoids, 5 flavonoids, and 1 phenolic compound. A. paniculata co-administration with either 50% w/w β-cyclodextrin or 1% w/w SDS, alone or in combination with 10% w/w piperine, significantly increased systemic andrographolide exposure by enhancing bioavailability (131.01% to 196.05%) following single and multiple oral co-administration. Glucuronidation is one possible biotransformation pathway for andrographolide, as evidenced by the excretion of glucuronide conjugates in urine and feces.
Conclusion
The combination of solubilizing agents and a bioenhancer improved the oral bioavailability and pharmacokinetics of andrographolide, indicating potential implications for A. paniculata formulations and clinical therapeutic benefits. Further investigation in clinical studies is warranted.
Introduction
The herbaceous plant Andrographis paniculata (Burm. f.) Nees (Acanthaceae), commonly known as Fah-Talai-Jone in Thailand, has gained attention for its antiviral activity against severe acute respiratory syndrome coronavirus 2 (SARS-CoV-2) (Sa-Ngiamsuntorn et al. Citation2021). Recent clinical studies have shown that A. paniculata has the potential to be used in the treatment of mild to moderate symptoms of coronavirus disease 2019 (COVID-19) (Tanwettiyanont et al. Citation2022). The beneficial pharmacological activities of A. paniculata with proven safety profiles are documented in the literature of Ayurvedic medicine and allopathic medicine (Jarukamjorn and Nemoto Citation2008; Mehta et al. Citation2021; Intharuksa et al. Citation2022). The World Health Organization (WHO) monograph (WHO Citation2002) provides characteristics of this plant and its uses in the form of dried powder. The evidence shows that a ground powder obtained from dried aerial parts of A. paniculata contains several diterpenoid lactones and flavonoids, which may be responsible for its biological effects (Song et al. Citation2013; Zhao et al. Citation2014; Wang et al. Citation2018). This herbal plant contains a unique phytochemical profile, which gives it specific pharmacological properties. Therefore, this present study characterized the profile of phytoconstituents in A. paniculata powder using the high performance liquid chromatography quadrupole time of flight mass spectrometry (LCMS-QTOF).
Although the evidence indicates that andrographolide is a major chemical marker responsible for pharmacological effects of A. paniculata (Cheung et al. Citation2001; Naik and Hule Citation2009; Chao and Lin Citation2010), its clinical use is hindered by the limited oral bioavailability. This limitation is possibly due to its diterpenoid chemical structure, which hinders water solubility and consequently restricts its absorption into the systemic circulation after oral administration (Loureiro Damasceno et al. Citation2022; Songvut et al. Citation2022). To enhance the clinical therapeutic potential, this research has developed pharmaceutical formulations using ground A. paniculata powder. These formulations aim to enhance water solubility through the incorporation of solubilizers and bioenhancers. The European Medicines Agency (EMA) recommends the use of β-cyclodextrin as a solubilizing agent (EMA Citation2017a), which has been extensively studied and showed the improved water solubility of drugs. The optimal stoichiometry of drug/β-cyclodextrin complexation has been reported to be 50:50% w/w (Brewster and Loftsson Citation2007; Saokham et al. Citation2018). Sodium dodecyl sulfate (SDS) is commonly used as a minor component in immediate-release tablets or capsules, typically in ranges from 0.2% to 1.5% w/w (Rowe et al. Citation2006; EMA Citation2017b). For safety considerations, a long-term study conducted on rats indicated that the administration of SDS at a concentration of 1.0% w/w mixed in their diet did not result in any abnormalities (Elder Citation1983). In pharmaceutical applications, solubilizers are commonly combined with bioenhancers, which are agents that enhance the bioavailability and bioefficacy of a co-administered substance. In several studies, piperine has been used in combination with the test compound at dose ratios ranging from 1% to 50% w/w (Johnson et al. Citation2011; Zeng et al. Citation2017; Junsaeng et al. Citation2019). In addition, a previous investigation has demonstrated that the addition of piperine resulted in a significant increase in the systemic exposure of herbal extract’s active compounds (Boonyarattanasoonthorn et al. Citation2022).
Due to the inadequate production of A. paniculata extract and the shortage of pure andrographolide during the COVID-19 pandemic (Chokwarakul Citation2021), the uses of dried ground powder of A. paniculata is recommended for phytopharmaceutical applications. However, the pharmacokinetic study of A. paniculata powder combining with solubilizer and bioenhancer are still lacking. It is of interest to determine the pharmacokinetic improvements of andrographolide in A. paniculata powder in combination with different mechanism solubilizers and bioenhancer. In the preclinical studies for drug development, beagle dogs were chosen due to their suitability for ingesting drug formulations in capsule form. An in vivo pharmacokinetic analysis with these dogs is crucial before proceeding to human studies. Therefore, the objective of this present study is to investigate the comparative pharmacokinetics in beagle dogs by comparing the pharmacokinetic profiles of a capsule containing A. paniculata powder alone compared with the newly developed combination capsules containing solubilizers (β-cyclodextrin or SDS) either individually or in combination with a bioenhancer (piperine).
Materials and methods
Chemicals
Andrographolide analytical standard (100.00% purity) was purchased from Phytolab GmbH & Co.KG (Vestenbergsgreuth, Germany). Digoxin (purity 95.0%) internal standard (IS), was purchased from Sigma-Aldrich (St. Louis, MO, USA). HPLC-grade methanol and acetonitrile were purchased from Merck (Darmstadt, FR, Germany). Formic acid (AR grade) was purchased from Merck (Darmstadt, FR, Germany). Milli-Q water was obtained from a purification system (Millipore, Bedford, MA, USA) and was used throughout the analysis. A lyophilized powder of β-glucuronidase type IX-A, derived from Escherichia coli, was purchased from Sigma-Aldrich (St. Louis, MO, USA). The powder had a glucuronidase activity ranging between 1,000,000 to 5,000,000 units/g of protein (glucuronidase activity = 2,354,185 units/g protein). Sodium dihydrogen phosphate and disodium hydrogen phosphate were also obtained from Sigma-Aldrich (St. Louis, MO, USA).
Characterization of phytochemical profile of A. paniculata powder using LCMS- QTOF analysis
Chromatographic experiments were performed with an Agilent 1260 infinity series high performance liquid chromatography (HPLC) system (Agilent, Waldbronn, Germany) coupled with an Agilent 6540 QTOF-MS spectrometer (Agilent Technologies, Singapore). The separation was performed on a Luna C18(2) column, size 4.6 mm × 150 mm, 5 µm (Phenomenex, Torrance, CA, USA) at a flow rate of 500 µL/min and the control temperature at 35 °C. The mobile phase A was milli-Q water and B was acetonitrile. Both phases contained 0.1% (v/v) formic acid. The gradient elution mode started with a 25% B to 95% B linear gradient within 15 min and was maintained at this ratio for 10 min with a post-run for 5 min before the next injection. The injection volume was 10 µL. The operating parameters for MS detection were as follows: drying gas (N2) flow rate 10.0 L/min; temperature 350 °C; nebulizer pressure 30 psig; capillary 3500 V; skimmer 65 V; octapole RFV 750 V; and a fragmentation voltage of 100 V in positive mode. The mass range was set at m/z 100–1000 Da with a 250 ms/spectrum. The non-target MS/MS mode was set up at three collision energies of 10, 20, and 40 V using high purity nitrogen (99.999%) as the collision gas. All data acquisition and analyses were controlled by MassHunter Data Acquisition Software Version B.05.01 and MassHunter Qualitative Analysis Software B.06.00, respectively (Agilent Technologies, USA). The m/z and fragmentation patterns of each compound were identified using metabolite databases including the METLIN PCD/PCDL database (Agilent Technologies, USA), the Human Metabolome Database (http://www.hmdb.ca), and the Lipid maps Database (https://www.lipidmaps.org).
Formulation development and preparation
The study medications were provided by the Chao Phya Abhaibhubejhr Hospital Foundation. Four preparations of A. paniculata capsules were developed and formulated, as shown in . A. paniculata powder was prepared by using the dried aerial parts of the plant. This raw material was then analyzed for its content of andrographolide () following the previously published method (Pholphana et al. Citation2013). The first formulation of A. paniculata powder alone was prepared by filling a capsule shell with 200 mg of coarse powder. To develop the combination formulation, two main kinds of solubilizing agents were used in this study [β-cyclodextrin (Zou et al. Citation2022) and sodium dodecyl sulfate (SDS) (Bahr et al. Citation2019)]. β-Cyclodextrin was formulated using a ratio of 50:50% w/w of andrographolide:β-cyclodextrin. The other solubilizer was SDS, which was prepared as 1% w/w of andrographolide. For the duo-combination, a bioenhancer (10% w/w of piperine) and a solubilizer (1% w/w of SDS) were used in preparation.
Table 1. Formulation development of A. paniculata capsules.
Table 2. Andrographolide contents in A. paniculata capsules (data are expressed as means ± SD, n = 10).
Animals
Eight healthy male beagle dogs between 2.0 and 2.5 years of age, with body weights of 10–15 kg, were obtained from the Chulalongkorn University Laboratory Animal Centre (CULAC), Thailand. All dogs were reused once after a washout period of one week. The animals were housed under conditions as follows: 22 ± 1 °C temperature with 50 ± 20% humidity, and 12 h light-dark cycles. Food was provided once a day and all dogs had unrestrained (ad libitum) access to water. The animal study protocol was approved by the Institutional Animal Care and Use Committee of the CULAC, Chulalongkorn University, Thailand (animal use protocol number: 2273004, approval number 2273004, date of approval: 07/02/2022). This study was performed in accordance with institutional policy and regulations regarding animal care and use at Chulalongkorn University and the Animal Research: Reporting of In Vivo Experiments (ARRIVE).
Pharmacokinetic study
The study animals were divided into four groups (n = 4 dogs/group) receiving oral administration of A. paniculata capsules according to the group categorization in . Group 1 received capsules containing A. paniculata powder (calculated as andrographolide 3 mg/kg); groups 2–4 received capsules containing the same amount of the powder (equivalent to 3 mg/kg of andrographolide) plus either solubilizer or solubilizer together with bioenhancer. More specifically, group 2 received β-cyclodextrin, group 3 received SDS, and group 4 received piperine plus SDS, respectively. The pharmacokinetic study of the four groups was investigated comparatively, with both single and multiple oral doses administered over a period of 7 consecutive days. Animals were maintained in the facilities at ambient temperature and were fed commercial dog food. Beagle dogs were fasted overnight, 10 h before the administration, but water was still available to them. During the pharmacokinetic study, each dog was transferred to an isolated steel cage (300 × 190 × 200 cm). Blood collection (2 mL) was performed from the cephalic vein at 15 min, 30 min, and 1, 2, 4, 8, and 24 h (on Day 1) after single oral dosing; and at 15 min, 30 min, and 1, 2, 4, 8, 24, 48, and 72 h (on Days 7–9) after multiple oral dosing. All collected serial blood samples were centrifuged at 5,000 × g, 4 °C for 10 min and plasma was stored at −20 °C until analysis. For safety and hematological evaluations, blood collection at pre- and post-dose (day 1 and day 7) was performed; these blood samples were analyzed for hemoglobin, white blood cell count (WBC), red blood cell count (RBC), and platelets. The biochemical components; including blood urea nitrogen (BUN), creatinine, aspartate aminotransferase (AST), alanine aminotransferase (ALT), and alkaline phosphatase (ALP) were also evaluated at pre- and post-dose to determine the safety profiles of each formulation.
Sample preparation
All plasma and urine samples collected from beagles were first centrifuged at 5,000 × g, 4 °C, for 10 min. The supernatant was transferred to a cryotube as an aliquot sample and then stored at −20 °C until analysis. Feces samples were collected, mixed with methanol (50:50% w/v), and the supernatants were subsequently filtered for LCMS-QqQ analysis. An aliquot of plasma, urine, or feces was further processed by protein precipitation using 100% methanol with an additional internal standard (IS). Briefly, 50 µL of sample was added to 200 µL of methanol containing 50 µg/L of digoxin (IS). These pretreated samples were vortexed for 10 min then centrifuged at 14,000 × g, 4 °C, for 10 min. The supernatant was filtered through a 0.2 µM PVDF membrane (Chrome Tech Inc, USA.) before analysis.
In order to analyze a preliminary conjugated metabolite of andrographolide glucuronide in urine and feces samples, the previous published method was modified in this step (Songvut et al. Citation2023). Briefly, pre-incubation of 50 μL of each sample was carried out at 37 °C, with 50 μL of β-glucuronidase (1,000 U/mL) in sodium phosphate buffer (pH 6.8) for 30 min. Subsequently, 150 μL of methanol containing 50 µg/L of IS was added for sample extraction. The mixtures were vortexed and centrifuged at 14,000 × g at 4 °C for 10 min. The supernatants (150 μL) were then filtered using a 0.2-µM PVDF membrane (Chrome Tech, Inc.). Finally, the extracted samples were injected into a LCMS-QqQ instrument.
Analytical procedures and method validation
The bioanalytical assay was developed by Laboratory of Pharmacology, Chulabhorn Research Institute (Thailand) using a liquid chromatography triple quadrupole mass spectrometry (LCMS-QqQ), following a previously published method (Songvut et al. Citation2023). The analytical procedures were partially validated according to the U.S. Food and Drug Administration (USFDA) bioanalytical Method Validation Guideline (USFDA Citation2018) and the International Conference on Harmonization (ICH) guideline M10 on bioanalytical method validation (ICH Citation2019). The quality control samples, including lower limit of quantification (LLOQ), low concentration (LQC), medium concentration (MQC), and high concentration (HQC) samples, were prepared for analysis during method validation. All key performance characteristics of the method were within the acceptable requirements. The selectivity was evaluated and no influences of endogenous interference were observed in blank beagle plasma, urine, or feces; indicating that the method was highly selective. The calibration curve of andrographolide in beagle plasma, urine, and feces showed a good linearity at 3.90–1,000 µg/L (correlation coefficient, R2 ≥ 0.99) with LLOQ at 3.90 µg/L. The calibration curve for determination of the total amount of andrographolide after hydrolysis of β-glucuronidase was carried out under the same incubation conditions, as previously described in the urine and feces sample preparation. The intraday and interday assay of accuracy and precision met the acceptance criteria (i.e., %RSD and %CV were less than 15% for LQC, MQC, and HQC samples, and less than 20% for LLOQ samples). Analysis of matrix interferences indicated that there was no significant matrix effect in beagle plasma, urine, and feces samples. The stability testing of plasma, urine, and feces samples was assessed under the conditions of freeze-thaw stability at −20 °C, long-term stability at −20 °C for 2 months, short-term stability at room temperature for 6 h, and in an autosampler at 4 °C for 24 h. All QC samples were deemed stable within the accepted criteria of 85–115% range.
LCMS-QqQ instrument conditions
The optimized LC-MS method was operationalized using an LCMS-8060NX (Shimadzu, Japan) equipped with an electrospray ionization (ESI) source. The stationary phase of liquid chromatography was run on a VertiSep (Vertical Chromatography Co., Ltd.) C18 column, size 100 mm x 3.0 mm, with C18 guard column. The LC system was run using a mobile phase of Milli-Q water (A) and acetonitrile (B) following gradient: 0.0–1.5 min, mobile phase was maintained at 40%B v/v then the gradient was increased up to 100%B v/v during 1.5–3.0 min, and maintained at 100%B v/v during 3.0–4.5 min. The gradient was returned to 40%B v/v at 4.5–6.0 min. These LC conditions were run at a flow rate of 0.5 mL/min with automatic injection of 10 µL sample volume, at 40 °C column oven temperature. For quantitative determination of andrographolide, the MS system was operated using negative mode ESI with multiple reaction monitoring (MRM). Mass transition of andrographolide was determined using a precursor ion/product ion at 349.1/287.2 for the quantitative chromatogram, and 349.1/331.1 for the qualitative chromatogram. The MRM of digoxin (IS) was 779.3/649.2. Data acquisition was operated using LabSolution software (Shimadzu, Japan).
Data processing and statistical analysis for pharmacokinetics
Pharmacokinetic profiles and parameters were analyzed by non-compartmental calculation using PK solutions software (version 2.0). The linear trapezoidal rule was used to calculate the area under the curve (AUC(0-t)). The AUC(0-∞) was extrapolated following the equation: AUC(0-∞) = AUC(0-t) + (Clast/kel), where kel is the elimination rate constant and Clast is the quantifiable concentration at the last time point. The half-life (t1/2) was calculated following the equation: t1/2 = 0.693/kel. The clearance (Cl) was calculated following the equation: Cl = Dose/AUC. The volume of distribution (Vd) was calculated following the equation: Vd = Dose/(AUC x kel). The maximum plasma concentration (Cmax) and time to reach maximum plasma concentration (Tmax) were taken from the graph of plasma concentration-time profiles. The comparative profiles of plasma concentration-time relationships between each group were plotted using GraphPad Prism 9.3.0 (GraphPad Software, USA). The relative bioavailability of the combination formula was calculated as follows: F= (AUC(0-t) of combination formula/AUC(0-t) of A. paniculata alone) ×100%. The accumulation ratio was calculated based on the AUC at steady state over the AUC of single dose.
Data were analyzed using SPSS statistical software (Version 22.0). The continuous data were expressed as the mean ± standard deviation (SD) and the non-continuous data was presented as the median [IQR]. The Shapiro–Wilk test was used to determine the normality of the data. To compare the pharmacokinetic parameters between treatment groups receiving different formulations, comparisons in analysis of variance (ANOVA) were used with Scheffe’s post hoc test in parametric analysis, or Kruskal-Wallis test in nonparametric analysis, where appropriate. Differences were considered statistically significant at p-values less than 0.05.
Results
Characteristics of the phytochemical profile of A. paniculata powder
Characterization of the phytochemical profile of A. paniculata powder was performed using a modified method of the LCMS-QTOF analytical technique (Songvut et al. Citation2023). The fingerprint was found to be much more informative when analyzed in the positive ion mode. A total of 13 compounds (, ) were tentatively identified using the chromatographic spectra of A. paniculata ground powder that was dissolved in 70% methanol. All phytochemical constituents were determined by their specific characteristics of fragmentation patterns using metabolite databases.
Table 3. Characterization of the phytochemical constituents of A. paniculata powder.
Compound M1 (M1, tR = 4.286)
Compound M1 turned up at a retention time of 4.286 min, and showed a protonated molecular ion [M + H]+ at m/z 355.1016 (molecular formula C16H18O9). M1 had subsequent fragment ions at m/z 163.0379, 145.0273, 135.0429, 117.0328, and 89.0374. Therefore, M1 was proposed as caffeoylquinic acid. The binding position of caffeic acid to quinic acid cannot be defined.
Compound M2 (M2, tR = 5.972)
Compound M2 at m/z 333.2052 [M + H-H2O]+ was detected at a retention time of 5.972 min and was tentatively identified as 14-deoxy-11-hydroxyandrographolide (molecular formula C20H30O5). The product ion at 315.1941 was produced due to the loss of an H2O molecule, which subsequently led to the fragment ion at 297.1839 through further losses of H2O, followed by the derived fragment ions at 257.1525/205.1571/175.1468/145.0997/111.0797.
Compound M3 (M3, tR = 6.157)
The MS chromatogram of compound M3, which appeared at a retention time of 6.157 min, showed a protonated ion [M + H]+ at m/z 787.1926. The fragment ions of M3 were characterized in the MS spectrum at m/z: 625.1387(-162Da), 463.0856(-162Da), 287.0539(-176Da), and 145.0463, which corresponded to the characteristic fragment ions of kaempferol 3-O-sophoroside 7-O-glucuronide, based on the HMDB database. Therefore, compound M3 was tentatively identified as kaempferol 3-O-sophoroside 7-O-glucuronide (molecular formula C33H38O22).
Compound M4 (M4, tR = 6.288)
Compound M4 was observed at m/z 701.4252 as a [2M + H]+ protonated ion. As a result of losing neutral H2O molecules, the first loss of H2O produced the m/z at 333.2050 as a [M + H-H2O]+ ion, then additional H2O losses resulted in the observed MS ion at 315.1946 as a [M + H-2H2O]+ ion. These MS characteristics of M4 were consistent with the molecular formula of C20H30O5 and were determined to be isoandrographolide.
Compound M5 (M5, tR = 6.580)
A protonated ion [M + H]+ of compound M5 was detected at m/z 447.0913 with a retention time of 6.580 min. Its fragmentation indicated a deductive glucuronic acid (-176 Da) at m/z 271.0593 followed by the corresponding fragment ion at m/z 153.0169. This inferred that M5 could be a conjugated glucuronide of apigenin. Thus, M5 was determined to be apigenin 7-glucuronide (molecular formula C21H18O11).
Compound M6 (M6, tR = 6.647)
Compound M6, with a protonated ion [M + H]+ of m/z 463.1225, was deduced as kaempferide 7-glucoside (molecular formula C22H22O11), due to the presence of its MS/MS at 301.0695, which is a predicted product ion of this compound when using LCMS-QTOF positive ionization analysis.
Compound M7 (M7, tR = 7.148)
A protonated molecular ion of m/z 463.0863 [M + H]+ of compound M7 was found at a retention time of 7.148 min. The MS/MS product ion was fragmented by losing a glucuronide structure (-176 Da), resulting in an m/z of 287.0542 for kaempferol. Thus, M7 was deduced as kaempferol-3-glucuronide (molecular formula C21H18O12) based on the experimental LCMS spectrum in the HMDB online database.
Compound M8 (M8, tR = 7.591)
Compound M8, a protonated ion [M + H]+ detected at m/z 477.1385, corresponded to a molecular formula of C23H24O11. The MS product ion at 315.0858 indicated a deduction of a glucoside (-162 Da), due to the loss of a C6H10O5 molecule. A further fragment ion was subsequently observed at 145.0485, which was specified as a fragmentation of 4′,5-dihydroxy-7,8-dimethoxyflavone. Therefore, M8 was tentatively identified as 4′,5-dihydroxy-7,8-dimethoxyflavone glucoside.
Compound M9 (M9, tR = 7.684)
Compound M9 was detected at a retention time of 7.684 min. A protonated molecular ion [M + H]+ of m/z 351.2158 was observed in its MS chromatogram in positive ion mode. Due to the successive loss of three molecules of H2O, the resulting fragmentation pattern yielding ions of 333.2049, 315.1949, and 297.1837, respectively, was observed. A further fragmentation was detected at 257.1526/207.1736, which was predicted to be an andrographolide isomer.
Compound M10 (M10, tR = 8.572)
The MS chromatogram of compound M10 was eluted at a retention time of 8.572 min. A protonated ion [M + H]+ of m/z 333.2053 was deduced as an andrographolide isomer, with a molecular formula of C20H30O5. The consecutive loss of two molecules of H2O led to a fragmentation pattern of m/z 315.1948 and 297.1845, respectively.
Compound M11 (M11, tR = 8.853)
Compound M11, at a retention time of 8.853 min, was a molecular ion of a dimer [2M + H]+ detected at m/z 701.4259. The loss of an H2O molecule from a protonated ion [M + H]+ at 351.2165, led to a fragment ion at 333.2058. The consecutive loss of H2O molecules led to a fragment ion at m/z 315.1953, and this product ion was then further fragmented at 297.1844 due to the loss of another H2O molecule. Compound M11 was identified as andrographolide (molecular formula C20H30O5), which was comparable to the authentic compound.
Compound M12 (M12, tR = 9.633)
Compound M12, with a retention time of 9.633 min, was an adduct ion [M + NH4]+ observed at m/z 498.3055. The product ion of m/z 319.2263 was fragmented to m/z 301.2163, due to the loss of an H2O molecule. A further fragmentation was typically produced at m/z 289.2156, which tentatively indicated that M12 was a neoandrographolide (molecular formula C26H40O8).
Compound M13 (M13, tR = 11.602)
Compound M13 was observed at m/z 669.4357 as a [2M + H]+ protonated ion, which was based on a molecular formula of C20H30O4. The sequence of MS fragmentation was observed at m/z: 317.2111/299.2003/287.2003/229.1216/205.1218/179.1057/153.0906, leading to the tentative identification of M13 as dehydropinifolic acid, a natural diterpenoid substance.
Safety evaluation of the study products
This study included an evaluation of the safety of the products under the pharmacokinetics investigation (). The results of this evaluation indicated that co-administration of solubilizer and bioenhancer with A. paniculata powder were well-tolerated in beagle dogs. No significant changes in blood chemistry or hematological evaluations were observed throughout the study. None of the beagle dogs exhibited any abnormal physical characteristics after receiving A. paniculata orally co-administered with β-cyclodextrin, SDS, or piperine, whether as single or multiple doses.
Table 4. Tolerability profile after oral administration of A. paniculata preparations (equivalent to 3 mg/kg of andrographolide).
Comparative pharmacokinetics
In this pharmacokinetic study, a validated quantitative high-performance liquid chromatography coupled with triple quadrupole mass spectrometry (LCMS-QqQ) method (Songvut et al. Citation2023), which is a highly sensitive and selective analytical technique, was used to determine the levels of andrographolide in plasma, urine, and feces samples collected from the beagles.
shows the comparative mean plasma concentrations versus time profiles of andrographolide after single and multiple oral administration of A. paniculata across groups; that is, between oral administration of the capsule formulation containing A. paniculata powder alone (group 1), the combination formulation capsules with different solubilizers (groups 2 and 3), and the capsules with bioenhancer plus solubilizer (group 4). Pharmacokinetic parameters were calculated by dose-normalization, and are summarized in . Moreover, additional comparative pharmacokinetics between single and multiple doses are also illustrated in and .
Figure 2. (a–d). Comparative mean plasma concentration versus time profiles of andrographolide after single and multiple oral administration of A. paniculata alone and in different combination formulations (equivalent to 3 mg/kg of andrographolide) in beagle dogs. Data are presented as means ± SD (n = 4).
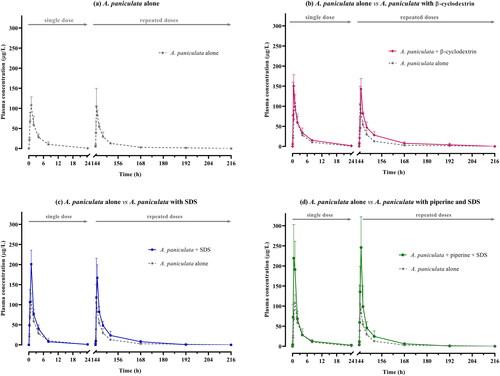
Table 5. Comparative pharmacokinetic parameters of andrographolide after single and multiple oral administration of A. paniculata capsules (equivalent to 3 mg/kg of andrographolide) in beagle dogs.
As shown in , the pharmacokinetic profiles indicated that the plasma concentration of andrographolide obtained after oral administration of A. paniculata alone (Group 1) was lower when compared to the concentrations obtained after oral administration of the other combination formula (group 2–4). The largest area under the curve was observed in group 4, which received the dual combination of bioenhancer plus solubilizer (piperine plus SDS). Considering the PK parameters as summarized in , the mean AUC(0-∞) of Group 4 following multiple oral administrations of the dual-combination, was nearly twice that of Group 1, which received A. paniculata alone. The maximum concentrations (Cmax) of groups 4 significantly increased, by more than double the corresponding values in group 1. These apparent differences correspond with the noticeable differences in relative oral bioavailability as seen in . The findings showed that the combination of piperine and SDS with A. paniculata in capsules resulted in a significant improvement in the relative oral bioavailability of andrographolide (148.89–196.05%), compared to the group that received A. paniculata alone, p = 0.036. The Tmax values of single and multiple doses were consistent across the groups. Tmax of groups 1–4 were observed within 0.5–1 h after oral dosing. Data on the other pharmacokinetic parameters indicate that there is a decrease in apparent clearance and a longer half-life, as observed after single and multiple doses of A. paniculata co-administered with solubilizer and bioenhancer.
This pharmacokinetic study also considered the elimination kinetics of A. paniculata, and found that andrographolide, a parent compound, was eliminated partly through the urine (, ) and feces () after oral administration. The highest excreted amounts of andrographolide in urine and feces were observed within 24 h after dosing. The percentage of recovery of andrographolide in urine ranged from 0.1 to 1.0%, while feces excretion of andrographolide was less than 0.4% of the administered dose in beagle dogs.
Figure 3. Cumulative amount of andrographolide in urine after multiple oral administration of A. paniculata formulations (equivalent to 3 mg/kg of andrographolide) in beagle dogs. Data are presented as means ± SD (n = 4).
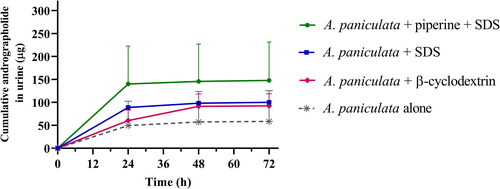
Table 6. Percent recovery of andrographolide elimination in urine and feces after single and multiple oral administration of A. paniculata capsules (equivalent to 3 mg/kg of andrographolide) in beagle dogs (data are expressed as means ± SD, n = 4).
Determination of glucuronide metabolites
The preliminary results of conjugated glucuronide metabolite levels in urine and feces following oral administration of the newly developed A. paniculata formulations, are illustrated in . The total amount of andrographolide released after glucuronide deconjugation via β-glucuronidase hydrolysis was significantly higher than the amount of free andrographolide found without hydrolysis. These data suggest the presence of a noticeable concentration of conjugated andrographolide-glucuronide in urine and feces samples. The preliminary results indicate that the combination of A. paniculata and solubilizers, with or without the use of a bioenhancer, increased the levels of conjugated andrographolide-glucuronide when compared to the group that received A. paniculata alone. However, no significant differences were observed in the levels of this conjugated glucuronide when the data taken from the groups receiving the combinations (group 2–4) were compared to each other.
Discussion
Pharmaceutical developments of A. paniculata formulations during COVID-19 outbreaks
Most pharmaceutical research of andrographolide usually focuses on drug delivery systems for pure andrographolide. Techniques such as solid dispersion, cyclodextrin inclusion complexes, and microemulsion have been investigated to enhance the water solubility and oral bioavailability of andrographolide. Additionally, advanced nanotechnologies including nanoemulsion, nanosuspension, nanoparticles, and nanomicelles have shown the potential to increase both water solubility and bioavailability of andrographolide by approximately 2–5 times (Loureiro Damasceno et al. Citation2022). Limited literature exists concerning the improvement of solubility and bioavailability of andrographolide in A. paniculata extract or the dried powder of ground A. paniculata herbal plant. The scarcity of reports may be attributed to the complex composition of the extract or coarse powder, which contains multiple compounds with varying solubilities. A shortage of the extract and pure andrographolide during the COVID-19 outbreak led to the exploration of formulations involving ground powder from dried aerial parts of A. paniculata, but the limited oral bioavailability of andrographolide in this powder remains a concern. According to the previous findings, andrographolide possesses poor solubility (log p = 2.632, Pandey and Rao Citation2018) and low oral bioavailability (Panossian et al. Citation2000), which may lead to its restricted disposition kinetics (Panossian et al. Citation2000; Yang et al. Citation2013; Bera et al. Citation2014; Xu et al. Citation2015; Pholphana et al. Citation2016; Songvut et al. Citation2022; Zeng et al. Citation2022). Therefore, this study formulated combinations for phytopharmaceutical applications incorporating a solubilizer and/or bioenhancer to increase the oral bioavailability of andrographolide and potentially mitigate the issue of pure compound and extract shortages during future outbreaks. This study indicated that common solubilizer (SDS) and bioenhancer (piperine) could enhance oral bioavailability by approximately 1.5–2.0 times. Notably, these enhancements were achieved without the complexities associated with formulations in drug delivery systems. This approach would be particularly beneficial for use in developing countries where access to antiviral drugs and conventional medicines is limited during COVID-19 outbreaks.
The drugability of andrographolide molecule and improvement in oral bioavailability
In accordance with Lipinski’s Rule of Five, andrographolide meets all the criteria required for the development of an oral drug, with a molecular weight below 500 g/mol, logP value lower than 5, fewer than 5 hydrogen bond donors, and less than 10 hydrogen bond acceptors (Lipinski et al. Citation2001). According to the Biopharmaceutics Classification System (BCS), andrographolide is categorized as a BCS class II compound, due to its poor water solubility and high permeability. To enhance the oral bioavailability of such BCS class II compounds, strategies including particle size reduction, solid dispersion, and the incorporation of cosolvents or surfactants should be implemented (Lee et al. Citation2019). In this present study, enhancing the water solubility of andrographolide through the use of common SDS or β-cyclodextrin could resolve the primary weakness of andrographolide absorption in the gut lumen. Additionally, SDS as a surfactant, could improve the permeability of andrographolide through enterocyte membrane disruption at the absorption site. A combination of common bioenhancers such as piperine could manage the issue of efflux transporters at the gut lumen and also mitigate the metabolic instability associated with first-pass metabolism after absorption. The observed sequence of oral bioavailability improvement in this study was as follows: piperine plus SDS > SDS > β-cyclodextrin > ground powder obtained from dried A. paniculata plant material. Additionally, the excretion of andrographolide as unchanged and possible glucuronide conjugates in urine also exhibited a similar tendency to systemic blood exposure. The combination of piperine and SDS had the highest amount of unchanged andrographolide and its glucuronide-conjugated metabolites, followed by the amounts reported in the group that received SDS, β-cyclodextrin, and A. paniculata alone.
Phytochemical profile of A. paniculata powder and bioanalytical method validation
The effectiveness of A. paniculata not only depends on its major components but also relates to its overall chemical profile. This study characterized the phytochemical profile of this plant material using a developed LCMS-QTOF technique, a sensitive analytical method for the identification of complex compounds. A total of 13 tentative plant metabolites, including 7 diterpenoids, 5 flavonoids, and 1 phenolic compound were identified in the profile of A. paniculata powder. Andrographolide was selected as a chemical marker for the pharmacokinetic investigation of its levels in biological samples of beagle dogs.
For quantitative determination of andrographolide in biological samples, the LCMS-QqQ method exhibited a linear detection range for andrographolide. The overall stability studies in plasma, urine, and feces inform appropriate sample handling for pharmacokinetic experiments, potentially supporting the reliability of pharmacokinetic data.
Comparative pharmacokinetics of andrographolide in A. paniculata formulations
The pharmacokinetics investigation showed that although the dosage of andrographolide given to each group was equivalent, the groups that received the combinations had a greater systemic exposure of andrographolide as compared to the group that received A. paniculata alone. This increase in the concentration of andrographolide in the plasma suggested improvements in its pharmacokinetics. The comparative pharmacokinetic parameters indicate an increased means of AUC and Cmax due to the effects of (1) SDS, a surfactant commonly used in pharmaceutical formulations to enhance the solubility of poorly soluble drugs. The potential mechanism of SDS involves the spontaneous self-assembly of SDS molecules into micelles. The hydrophobic portion of the drug compound becomes incorporated into the hydrophobic core of these micelles, consequently enhancing the drug’s solubility. It is an anionic surfactant that is widely used as a wetting agent in solid dosage forms (Bahr et al. Citation2019); (2) β-cyclodextrin, a solubilizer excipient frequently incorporated into pharmaceutical formulations, is a cyclic oligosaccharide composed of seven glucose units. It forms complexes that retain poorly soluble molecules within its hydrophobic interior, while the exterior of the complex remains hydrophilic, thereby enhancing solubility (Zou et al. Citation2022); and (3) piperine, a bioenhancer that increases bioavailability (Dudhatra et al. Citation2012), leading to increased pharmacological efficacy of the active compound. The evidence indicates that piperine may inhibit the efflux of P–glycoprotein (Han et al. Citation2008), possibly causing an increase in andrographolide systemic exposure in this way. The results of comparative pharmacokinetic studies indicate that single and multiple co-administration of piperine and SDS in A. paniculata capsules (group 4) resulted in the increase in the relative oral bioavailability of andrographolide (148.89% on day1 and 196.05% on day 7, respectively) when compared to a group that received A. paniculata alone.
According to the elimination kinetics results, less than 1.0% of the administered dose of andrographolide was recovered in the form of unchanged andrographolide excreted in urine and feces within the first 24 h. This result indicates that unchanged andrographolide (parent compound) is not the major clearance compound after oral administration of A. paniculata. This also corresponds with the observation that andrographolide was partly eliminated as glucuronide conjugated metabolites, which suggests that andrographolide is metabolized partly through a glucuronidation pathway, resulting in conjugated andrographolide glucuronide being one of the detectable metabolites in urine and feces. This biotransformation may be one of the causes of the limited amounts of andrographolide detected in the systemic circulation, leading to its restricted bioavailability. These findings on andrographolide’s metabolism and excretion strengthen the previously published reports which hypothesized that andrographolide’s poor oral bioavailability may be associated not only with its physicochemical properties, but also with its rapid biotransformation after oral administration (Ye et al. Citation2011). The interpretation of the data obtained from the enzymatic digestion of the glucuronide conjugates is limited by the indirect determination method used. The lack of a chemical standard for andrographolide glucuronide precludes the possibility of conducting a direct assay. Further research efforts should be focused on andrographolide glucuronide, not only to develop a selective analytical method but also to explore its biological and pharmacological activities.
Conclusions
The newly developed A. paniculata formulations, which were assessed through biochemical and hematological evaluations, were well-tolerated in beagle dogs. This study introduces a new approach for enhancing systemic exposure of andrographolide from A. paniculata through the use of solubilizers including β-cyclodextrin and sodium dodecyl sulfate individually or in combination with the bioenhancing agent (piperine). The developed combination formula demonstrated improved pharmacokinetics, as evidenced by the increase in the amount of andrographolide in the blood circulation, resulting in a relative oral bioavailability of 131.01–196.05%. After oral administrations of these combination formulas, an increase in the AUC was observed. Specifically, the apparent clearance of the group receiving the combinations decreased, while the half-life was extended. The small amounts of unchanged andrographolide in the excreta indicated that andrographolide administered was partly biotransformed into metabolites before elimination. Preliminary investigation into the elimination of metabolites suggests that glucuronidation via the phase II metabolic pathway may contribute to one of the possible pathways of biotransformation of andrographolide. The findings from this study on the improvement of the oral pharmacokinetics of A. paniculata encourage future product development for clinical therapeutic use in humans.
Author contributions
PK initiated the conception. PS, TB, TK, and PK developed the design. PS, TB, NN, TJ, TK, KS, and PK conducted the experiments. PS, TB, NN, and TJ analyzed the results. PS, TB, and TJ prepared the first draft of the manuscript. PS, TB, NN, TJ, TK, KS, and PK reviewed and edited the manuscript.
Acknowledgements
The authors would like to thank Miss Jittra Saehun for her contribution to the sample preparation in this study, and Dr. James M. Dubbs for proofreading. Lastly, we express our appreciation to the Food and Drug Quality Unit at Chulabhorn Research Institute for conducting the quantitative analysis of A. paniculata capsules.
Disclosure statement
No potential conflict of interest was reported by the author(s).
Data availability statement
The datasets generated and/or analyzed during the present study are not publicly available but are available from the corresponding author on reasonable request that needs a consensus from colleagues.
Additional information
Funding
References
- Bahr MN, Modi D, Patel S, Campbell G, Stockdale G. 2019. Understanding the role of sodium lauryl sulfate on the biorelevant solubility of a combination of poorly water-soluble drugs using high throughput experimentation and mechanistic absorption modeling. J Pharm Pharm Sci. 22(1):221–246. doi:10.18433/jpps30347.
- Bera R, Ahmed SK, Sarkar L, Sen T, Karmakar S. 2014. Pharmacokinetic analysis and tissue distribution of andrographolide in rat by a validated LC-MS/MS method. Pharm Biol. 52(3):321–329. doi:10.3109/13880209.2013.836544.
- Boonyarattanasoonthorn T, Kongratanapasert T, Maiuthed A, Hamlin R, Kijtawornrat A, Khemawoot P. 2022. Bioenhancing effects of piperine and curcumin on triterpenoid pharmacokinetics and neurodegenerative metabolomes from Centella asiatica extract in beagle dogs. Sci Rep. 12(1):20789. doi:10.1038/s41598-022-24935-7.
- Brewster ME, Loftsson T. 2007. Cyclodextrins as pharmaceutical solubilizers. Adv Drug Deliv Rev. 59(7):645–666. doi:10.1016/j.addr.2007.05.012.
- Chao WW, Lin BF. 2010. Isolation and identification of bioactive compounds in Andrographis paniculata (Chuanxinlian). Chin Med. 5(1):17. doi:10.1186/1749-8546-5-17.
- Cheung HY, Cheung CS, Kong CK. 2001. Determination of bioactive diterpenoids from Andrographis paniculata by micellar electrokinetic chromatography. J Chromatogr A. 930(1–2):171–176. doi:10.1016/s0021-9673(01)01160-8.
- Chokwarakul C. 2021. Local herbs against COVID-19 virus. In: Proceedings of RSU International Research Conference 2021. Pathum Thani, Thailand: Rangsit University.
- Dudhatra GB, Mody SK, Awale MM, Patel HB, Modi CM, Kumar A, Kamani DR, Chauhan BN. 2012. A comprehensive review on pharmacotherapeutics of herbal bioenhancers. Sci World J. 2012:637953–637933. doi:10.1100/2012/637953.
- Elder R. 1983. Final report on the safety assessment of sodium lauryl sulfate and ammonium lauryl sulfate. J Am Coll Toxicol. 2:127–181.
- [EMA] European Medicines Agency. 2017a. Cyclodextrins used as excipients report published in support of the ‘questions and answers on cyclodextrins used as excipients in medicinal products for human use’ (EMA/CHMP/495747/2013). London (UK): EMA. [accessed 2023 Mar 2]. https://www.ema.europa.eu/en/documents/scientific-guideline/questions-answers-cyclodextrins-used-excipients-medicinal-products-human-use_en.pdf.
- [EMA] European Medicines Agency. 2017b. Sodium laurilsulfate used as an excipient report published in support of the’questions and answers on sodium laurilsulfate used as an excipient in medicinal products for human use’ (EMA/CHMP/606830/2017). London (UK): EMA. [accessed 2023 Mar 2]. https://www.ema.europa.eu/en/documents/report/sodium-laurilsulfate-used-excipient-report-published-support-questions-answers-sodium-laurilsulfate_en.pdf.
- Han Y, Chin Tan TM, Lim LY. 2008. In vitro and in vivo evaluation of the effects of piperine on P-gp function and expression. Toxicol Appl Pharmacol. 230(3):283–289. doi:10.1016/j.taap.2008.02.026.
- [ICH] International Council for Harmonization. 2019. ICH guideline M10 on bioanalytical method validation. ICH. [accessed 2023 Mar 2]. https://database.ich.org/sites/default/files/M10_Guideline_Step4_2022_0524.pdf.
- Intharuksa A, Arunotayanun W, Yooin W, Sirisa-Ard P. 2022. A comprehensive review of Andrographis paniculata (Burm. f.) Nees and its constituents as potential lead compounds for COVID-19 drug discovery. Molecules. 27(14):4479. doi:10.3390/molecules27144479.
- Jarukamjorn K, Nemoto N. 2008. Pharmacological aspects of Andrographis paniculata on health and its major diterpenoid constituent andrographolide. J Health Sci. 54(4):370–381. doi:10.1248/jhs.54.370.
- Johnson JJ, Nihal M, Siddiqui IA, Scarlett CO, Bailey HH, Mukhtar H, Ahmad N. 2011. Enhancing the bioavailability of resveratrol by combining it with piperine. Mol Nutr Food Res. 55(8):1169–1176. doi:10.1002/mnfr.201100117.
- Junsaeng D, Anukunwithaya T, Songvut P, Sritularak B, Likhitwitayawuid K, Khemawoot P. 2019. Comparative pharmacokinetics of oxyresveratrol alone and in combination with piperine as a bioenhancer in rats. BMC Complement Altern Med. 19(1):235. doi:10.1186/s12906-019-2653-y.
- Lee SY, Abdullah LC, Rahman RA, Abas F, Chong GH. 2019. Role of polymers as crystal growth inhibitors in coprecipitation via solution-enhanced dispersion by supercritical fluids (SEDS) to improve andrographolide dissolution from standardized Andrographis paniculata extract. J Drug Deliv Sci Technol. 50:145–154. doi:10.1016/j.jddst.2019.01.026.
- Lipinski CA, Lombardo F, Dominy BW, Feeney PJ. 2001. Experimental and computational approaches to estimate solubility and permeability in drug discovery and development settings. Adv Drug Deliv Rev. 46(1–3):3–26. doi:10.1016/s0169-409x(00)00129-0.
- Loureiro Damasceno JP, Silva da Rosa H, Silva de Araújo L, Jacometti Cardoso Furtado NA. 2022. Andrographis paniculata formulations: impact on diterpene lactone oral bioavailability. Eur J Drug Metab Pharmacokinet. 47(1):19–30. doi:10.1007/s13318-021-00736-7.
- Mehta S, Sharma AK, Singh RK. 2021. Pharmacological activities and molecular mechanisms of pure and crude extract of Andrographis paniculata: an update. Phytomedicine Plus. 1(4):100085. 100085. doi:10.1016/j.phyplu.2021.100085.
- Naik SR, Hule A. 2009. Evaluation of immunomodulatory activity of an extract of andrographolides from Andographis paniculata. Planta Med. 75(8):785–791. doi:10.1055/s-0029-1185398.
- Pandey G, Rao C. 2018. Andrographolide: its pharmacology, natural bioavailability and current approaches to increase its content in Andrographis paniculata. Int J Complement Alt Med. 11:355–360.
- Panossian A, Hovhannisyan A, Mamikonyan G, Abrahamian H, Hambardzumyan E, Gabrielian E, Goukasova G, Wikman G, Wagner H. 2000. Pharmacokinetic and oral bioavailability of andrographolide from Andrographis paniculata fixed combination Kan Jang in rats and human. Phytomedicine. 7(5):351–364. doi:10.1016/S0944-7113(00)80054-9.
- Pholphana N, Panomvana D, Rangkadilok N, Suriyo T, Puranajoti P, Ungtrakul T, Pongpun W, Thaeopattha S, Songvut P, Satayavivad J. 2016. Andrographis paniculata: dissolution investigation and pharmacokinetic studies of four major active diterpenoids after multiple oral dose administration in healthy Thai volunteers. J Ethnopharmacol. 194:513–521. doi:10.1016/j.jep.2016.09.058.
- Pholphana N, Rangkadilok N, Saehun J, Ritruechai S, Satayavivad J. 2013. Changes in the contents of four active diterpenoids at different growth stages in Andrographis paniculata (Burm.f.) Nees (Chuanxinlian). Chin Med. 8(1):2. doi:10.1186/1749-8546-8-2.
- Rowe R, Sheskey P, Owen S. 2006. Handbook of pharmaceutical excipients. London (UK): Pharmaceutical Press.
- Sa-Ngiamsuntorn K, Suksatu A, Pewkliang Y, Thongsri P, Kanjanasirirat P, Manopwisedjaroen S, Charoensutthivarakul S, Wongtrakoongate P, Pitiporn S, Chaopreecha J, et al. 2021. Anti-SARS-CoV-2 activity of Andrographis paniculata extract and its major component andrographolide in human lung epithelial cells and cytotoxicity evaluation in major organ cell representatives. J Nat Prod. 84(4):1261–1270. doi:10.1021/acs.jnatprod.0c01324.
- Saokham P, Muankaew C, Jansook P, Loftsson T. 2018. Solubility of cyclodextrins and drug/cyclodextrin complexes. Molecules. 23(5):1161. doi:10.3390/molecules23051161.
- Song Y-X, Liu S-P, Jin Z, Qin J-F, Jiang Z-Y. 2013. Qualitative and quantitative analysis of Andrographis paniculata by rapid resolution liquid chromatography/time-of-flight mass spectrometry. Molecules. 18(10):12192–12207. 10.3390/molecules181012192.24084022.
- Songvut P, Pholphana N, Suriyo T, Rangkadilok N, Panomvana D, Puranajoti P, Satayavivad J. 2023. A validated LC–MS/MS method for clinical pharmacokinetics and presumptive phase II metabolic pathways following oral administration of Andrographis paniculata extract. Sci Rep. 13(1):2534. doi:10.1038/s41598-023-28612-1.
- Songvut P, Suriyo T, Panomvana D, Rangkadilok N, Satayavivad J. 2022. A comprehensive review on disposition kinetics and dosage of oral administration of Andrographis paniculata, an alternative herbal medicine, in co-treatment of coronavirus disease. Front Pharmacol. 13:952660. 952660. doi:10.3389/fphar.2022.952660.
- Tanwettiyanont J, Piriyachananusorn N, Sangsoi L, Boonsong B, Sunpapoa C, Tanamatayarat P, Na-Ek N, Kanchanasurakit S. 2022. Use of Andrographis paniculata (Burm.f.) Wall. ex Nees and risk of pneumonia in hospitalised patients with mild coronavirus disease 2019: a retrospective cohort study. Front Med (Lausanne). 9:947373. 947373. doi:10.3389/fmed.2022.947373.
- [USFDA] U.S. Food and Drug Administration. 2018. Guidance for industry: bioanalytical method validation. Maryland (MD): U.S. Department of Health and Human Services, Food and Drug Administration. [accessed 2023 Mar 2]. https://www.fda.gov/files/drugs/published/Bioanalytical-Method-Validation-Guidance-for-Industry.pdf.
- Wang Y, Jiao J, Yang Y, Yang M, Zheng Q. 2018. Screening and identification for immunological active components from Andrographis Herba using macrophage biospecific extraction coupled with UPLC/Q-TOF-MS. Molecules. 23(5):1047. doi:10.3390/molecules23051047.
- [WHO] World Health Organization. 2002. WHO monographs on selected medicinal plants. Geneva (Switzerland): World Health Organization. Vol. 2:12–24.
- Xu FF, Fu SJ, Gu SP, Wang ZM, Wang ZZ, He X, Xiao W. 2015. Simultaneous determination of andrographolide, dehydroandrographolide and neoandrographolide in dog plasma by LC-MS/MS and its application to a dog pharmacokinetic study of Andrographis paniculata tablet. J Chromatogr B Anal Technol Biomed Life Sci. 990:125–131. doi:10.1016/j.jchromb.2015.03.014.
- Yang T, Xu C, Wang ZT, Wang CH. 2013. Comparative pharmacokinetic studies of andrographolide and its metabolite of 14-deoxy-12-hydroxy-andrographolide in rat by ultra-performance liquid chromatography-mass spectrometry. Biomed Chromatogr. 27(7):931–937. doi:10.1002/bmc.2884.
- Ye L, Wang T, Tang L, Liu W, Yang Z, Zhou J, Zheng Z, Cai Z, Hu M, Liu Z. 2011. Poor oral bioavailability of a promising anticancer agent andrographolide is due to extensive metabolism and efflux by P‐glycoprotein. J Pharm Sci. 100(11):5007–5017. doi:10.1002/jps.22693.
- Zeng B, Wei A, Zhou Q, Yuan M, Lei K, Liu Y, Song J, Guo L, Ye Q. 2022. Andrographolide: a review of its pharmacology, pharmacokinetics, toxicity and clinical trials and pharmaceutical researches. Phytother Res. 36(1):336–364. doi:10.1002/ptr.7324.
- Zeng X, Cai D, Zeng Q, Chen Z, Zhong G, Zhuo J, Gan H, Huang X, Zhao Z, Yao N, et al. 2017. Selective reduction in the expression of UGTs and SULTs, a novel mechanism by which piperine enhances the bioavailability of curcumin in rat. Biopharm Drug Dispos. 38(1):3–19. doi:10.1002/bdd.2049.
- Zhao Y, Kao CP, Wu KC, Liao CR, Ho YL, Chang YS. 2014. Chemical compositions, chromatographic fingerprints and antioxidant activities of Andrographis Herba. Molecules. 19(11):18332–18350. doi:10.3390/molecules191118332.
- Zou L, Ding W, Huang Q, Yang X, Li J, Huang T, Li Z, Lin S, Feng J. 2022. Andrographolide/phospholipid/cyclodextrin complex-loaded nanoemulsion: preparation, optimization, in vitro and in vivo evaluation. Biol Pharm Bull. 45(8):1106–1115. doi:10.1248/bpb.b22-00154.