ABSTRACT
Background
The 23-valent pneumococcal polysaccharide vaccine (PPSV23) is used in the Japanese National Immunization Program for older adults and adults with increased risk for pneumococcal disease, however, disease incidence and associated burden remain high. We evaluated the cost-effectiveness of pneumococcal conjugate vaccines (PCVs) for adults aged 65 years and high-risk adults aged 60–64 years in Japan.
Research design and methods
Using a Markov model, we evaluated lifetime costs using societal and healthcare payer perspectives and estimated quality-adjusted life-years (QALYs), and number of prevented cases and deaths caused by invasive pneumococcal disease (IPD) and non-IPD. The base case analysis used a societal perspective.
Results
In comparison with PPSV23, the 20-valent PCV (PCV20) prevented 127 IPD cases 10,813 non-IPD cases (inpatients: 2,461, outpatients: 8,352) and 226 deaths, and gained more QALYs (+0.0015 per person) with less cost (-JPY22,513 per person). All sensitivity and scenario analyses including a payer perspective analysis indicated that the incremental cost-effectiveness ratios (ICERs) were below the cost-effectiveness threshold value in Japan (JPY5 million/QALY).
Conclusions
PCV20 is both cost saving and more effective than PPSV23 for adults aged 65 years and high-risk adults aged 60–64 years in Japan.
1. Introduction
Pneumococcal disease (PD) is a common illness caused by infection with the bacteria Streptococcus pneumoniae (S. pneumoniae), and is a significant source of morbidity and mortality in adults aged ≥50 years [Citation1]. Two classifications of PD exist: invasive PD (IPD) and non-IPD (including non-bacteremic pneumococcal pneumonia (NBP)) based on the site of infection. The National Institute of Infectious Disease (NIID) reported IPD incidence rates in a Japanese population aged ≥65 years as 5.57 per 100,000 population with associated mortality rates of 8.1% in 2019 [Citation2]. Miyazaki et al. reported an annual incidence rate of pneumococcal community-acquired pneumonia (CAP, it was assumed in this study that CAP and NBP refer to the same disease) as 89, 260, and 645 per 100,000 population in adults aged 18 to 64 years, 65 to 79 years, and ≥80 years, respectively, in their prospective, population-based study in Goto city from 2015 to 2020 [Citation3]. Given this, prevention of PD is especially important in Japan, a country with a considerable elderly population, where 29% of the population are aged ≥65 years [Citation4].
As of January 2024, 3 vaccines, the 23-valent pneumococcal polysaccharide vaccine (PPSV23) and the 13- and 15-valent pneumococcal conjugate vaccines (PCV13 and PCV15) are available in Japan. Only PPSV23 is included in the routine national immunization program (NIP) since October 2014 for all adults aged 65, 70, 75, 80, 85, 90, 95, and 100 years, as well as adults aged 60 to 64 years with increased risk such as impairment of heart, kidney, or respiratory function, or impairment of immune function due to human immunodeficiency virus (HIV) [Citation5]. From April 2024, only adults aged 65 years and adults aged 60 to 64 years with increased risk will be eligible for routine vaccination of PPSV23 under the NIP [Citation6]. Despite the introduction of the PPSV23 vaccination in the NIP, the percentage of IPD caused by PPSV23-serotypes out of total IPD cases has remained high in adults (67% and 47% in 2014 and 2022) [Citation7]. One of the reasons for this may be the low pneumococcal vaccination rates in adults [Citation8,Citation9]. This contrasts with the change in IPD since the introduction of pediatric PCV13. Indeed, the percentage of IPD caused by PCV13-serotypes was dramatically decreased after the introduction of PCV13 in the NIP system in November 2013 (53.2% and 2.3% in 2013 and 2022) [Citation7] with nearly a 100% pneumococcal vaccination rate [Citation10].
Unlike the immune response to plain polysaccharide vaccines such as PPSV23, the immune response to conjugate vaccines such as PCV13 and PCV15 provides long-term immunity through the production of new memory B cells [Citation11]. The efficacy of PPSV23 is known to decline from year 1, while no decline of PCV13 efficacy is observed in the first 5 years [Citation12–15]. Additionally, a new vaccine, the 20-valent pneumococcal conjugate vaccine (PCV20) has been licensed for the prevention of PD in the United States and has replaced PCV13 in prescribing practice. In Japan, an application to approve the use of PCV20 has been submitted to the Pharmaceuticals and Medical Devices Agency (PMDA) [Citation16]. PCV20 was investigated in a phase II study in adults aged 60 to 64 years and in two phase III randomized controlled trials in adults aged ≥18 years [Citation17–20]. The results of these studies demonstrate that PCV20 is associated with an improved immune response compared with PPSV23 and is anticipated to provide more robust protection compared with PPSV23 for the serotypes both vaccines have in common. The introduction of PCV20 could therefore prevent more IPD and NBP cases; it could also reduce deaths related to those diseases among the elderly and immunocompromised persons.
In advance of PCV20 approval in Japan, an economic evaluation of PCV20 and PCV15 compared with PPSV23 in an elderly population aged 65 years was conducted by Hoshi et al. [Citation21]. The study found both PCV20 and PCV15 were cost-effective compared with PPSV23, with PCV20 being the most cost-effective. However, since PCV20 and PCV15 were not available in Japan when they conducted the analysis, the US Centers for Disease Control and Prevention (CDC)’s adult vaccine prices were used. Furthermore, the authors considered a restricted population of adults aged 65 years. Given this, the findings may not be fully reflective of a Japanese population eligible for the NIP. To inform potential gaps in knowledge about the impact of PCV20 in a Japanese healthcare setting, this paper evaluates the cost-effectiveness of PCV20 versus PPSV23 in adults aged 65 years and high-risk adults aged 60 to 64 years from both payer and societal perspectives using the latest available data from Japanese sources. Additionally, an assessment of the cost-effectiveness of PCV15 versus PPSV23 and PCV20 versus PCV15 in the same population are explored as secondary objectives.
2. Patients and methods
2.1. Model description
The cost effectiveness of a single dose of the various pneumococcal vaccines was assessed using a decision-analytic Markov model. The model was adapted from a model previously published by Mendes et al., calibrated to reflect Japanese healthcare settings [Citation22]. The model utilized a deterministic framework and a Markov-type process to depict the risks and costs of IPD and all-cause NBP over remaining years of life in a hypothetical population of Japanese adults. The model population was stratified by age and risk profile (i.e. low, medium, or high-risk) at model entry.
Expected clinical outcomes and economic costs were calculated on an annual basis, using age, risk profile, vaccination status, vaccine type, and time since vaccination. IPD was assumed to include bacteremia and meningitis; all-cause NBP was stratified by setting of care (inpatient or outpatient). People vaccinated at model entry had a differential risk of future IPD and all-cause NBP. The magnitude of vaccine-associated risk reduction was dependent on clinical presentation (i.e. IPD or all-cause NBP), in addition to the type of vaccine used, time since vaccination, and risk profile. Risk of death from IPD, all-cause NBP requiring inpatient care, and other causes (i.e. other than IPD and all-cause NBP) were dependent on age and risk profile.
At each model cycle, individuals were modeled to remain in their current health state or transition to another health state, based on health state transition probabilities. In the original model published by Mendes et al., individuals were permitted to transition to a higher risk group (i.e. from low risk to medium risk or medium risk to high risk) but not to a lower risk group [Citation22]. However, due to a lack of Japanese specific evidence to inform this transition, individuals were not permitted to transition to lower or higher risk groups in the base case analysis. General population mortality risk was applied to all individuals, with additional mortality risk applied for IPD or inpatient NBP. Death was an absorbing health state. A model schematic is presented in .
Figure 1. Model structure.
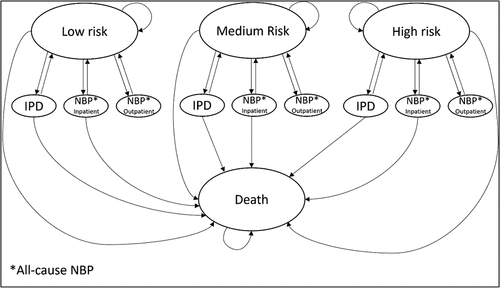
Expected costs of medical treatment for IPD and all-cause NBP were generated based on event rates and unit costs for the setting of care (i.e. inpatient or outpatient), age, and risk profile. Costs of vaccination (including vaccine and administration costs) were applied at model entry. The value of morbidity- and mortality-related work loss was included in the model. Clinical outcomes and economic costs were projected over the model lifetime horizon (up to 100 years) for each vaccination strategy. As with previous studies in this field, the model used a one-year cycle length and applied a half-cycle correction [Citation21,Citation23]. Cost-effectiveness was calculated in terms of cost per quality-adjusted life-year (QALY) gained and cost per life-year (LY) gained. Costs and outcomes were discounted at a rate of 2% per year [Citation24]. A cost-effectiveness threshold of JPY5,000,000 per QALY gained was used in the analysis to assess cost-effectiveness [Citation25]. The incremental net monetary benefit (INMB) was calculated as follows:
INMB = incremental QALYs gained with the intervention strategy (e.g. PCV20) compared to the comparator strategy (e.g. PPSV23) × threshold – incremental costs associated with the intervention strategy compared to the comparator strategy
The base case analysis was conducted from a societal perspective (inclusive of medical, productivity loss, and vaccination costs) as it was important to consider productivity loss, given the high burden of the disease [Citation24]. Results from a payer perspective are presented as a scenario.
2.2. Model estimation
The main model parameters are presented in and further presented in the supplemental online material. Additional details on the targeted literature review (TLR) are provided in the supplemental online material. If data from Japan were not available, other appropriate data were considered.
Table 1. Base-case model inputs.
2.2.1. Population
In the base case, the target population was adults aged 65 years and high-risk adults aged 60 to 64 years. Total population numbers and age distribution were informed by the official age structure of Japan (as of 2022) [Citation26]. The proportion of adults with high-risk (1.7%) was informed by Imai et al. [Citation27]. Further information on risk categories is provided below. Other scenarios were tested for the modeled population: adults aged ≥65 years and high-risk adults aged 60 to 64 years (scenario A), adults aged 65 years only (scenario B), adults aged 65 years and moderate and high-risk adults aged 60 to 64 years (scenario C), and adults aged 66 to 84 years only (scenario D).
2.2.2. Risk definition
In the base case, the risk definition from Imai et al. was used as this study considered guidelines and recommendations from the United States, England, and Japan [Citation27–31]. Medium-risk conditions included chronic heart disease, chronic lung disease, diabetes mellitus, chronic liver disease, and alcoholism. High-risk conditions included chronic renal disease, cancer, HIV/AIDS, functional or anatomic asplenia, organ transplant, and cerebrospinal fluid leakage. The same source was used to inform the proportion of at-risk adults and risk ratio for disease incidence. Imai et al. sourced the proportion of at risk adults from 2 Japanese claims databases, the database of JMDC Inc. (JMDC database) for adults aged <65 years and Medical Data Vision Co., Ltd. (MDV database) for adults aged ≥65 years [Citation27,Citation32,Citation33]. The JMDC database consists of approximately 16 million beneficiaries of health insurance associations and the MDV database contains Diagnosis Procedure Combination (DPC) data from approximately 27% facilities out of the nationwide DPC target hospitals.
2.2.3. Disease incidence and serotype distribution
Disease incidence and serotype distribution before the COVID-19 pandemic (2017–19) were used for the base-case analysis as PD incidence is expected to return to pre-pandemic levels. Incidence data during the COVID-19 pandemic (2020–22) were used for scenario analysis to consider the impact of COVID-19. Incidence rates for IPD and NBP were calculated based on surveillance data from NIID and the JMDC database, respectively [Citation32,Citation34]. Detailed information on the methods employed are described in the supplemental online material.
The proportion of NBP due to S. pneumoniae of any serotype was assumed to be 16.5% in the base case, based on data from Miyazaki et al. [Citation3]. In scenario analysis, a value of 20% was applied, sourced from a study by Kobayashi et al. [Citation35]. Percentages of vaccine serotypes for IPD were obtained from the latest data reported by the Epidemiological Information on Invasive Pneumococcal Infections in Adults and Children Research Group [Citation7]. Values were assumed to remain constant throughout the time horizon. Vaccine serotypes for NBP were assumed to be the same as IPD in the base-case and scenario analyses.
2.2.4. Vaccine coverage and history
Vaccine coverage was assumed to be 40.4% of those eligible for vaccination; consistent with the value used in a previously published economic evaluation, sourced from the Japanese Infectious Agents Surveillance Report (IASR) from 2018 [Citation9,Citation21]. This value was applied to the modeled population. Two additional scenarios for vaccine coverage were explored: 52%, consistent with data available from the IASR 2023 and 20%, which is an assumption to test the effects of poor uptake [Citation2].
In the base case, it was assumed that the studied population is vaccine-naïve, as there were limited data to inform this parameter. Data on vaccine history for persons with CAP were available from a study by Nakashima et al., who reported the proportion of persons aged ≥65 years who had been vaccinated within the previous 5 years (55.6% with only PPSV23 and 1.4% with both PPSV23 and PCV13) in Japan. These values were used in scenario analysis [Citation36].
2.2.5. Effectiveness of PCVs
Methods to derive the effectiveness of PCVs against vaccine type invasive pneumococcal disease (VT-IPD) and vaccine type non-bacteremic pneumonia (VT-NBP) were described in Mendes et al. [Citation22]. Values used for vaccine effectiveness are summarized in Supplementary Table S1. The same serotype-specific effectiveness was assumed for all PCVs and for all included serotypes.
Efficacy inputs for the initial period of effectiveness of PCVs against VT-IPD for low- and medium-risk people were based on data for adults aged ≥65 years in the Community-Acquired Pneumonia Immunization Trial in Adults (CAPiTA) per-protocol population and from post hoc analyses for people aged 60 to 64 years [Citation37,Citation38]. For people aged ≥65 years with low or medium risk, initial vaccine effectiveness was assumed to be 75% [Citation37]. For people aged 60 to 64 years, low- and medium-risk effectiveness was derived using age-specific relative changes in vaccine effectiveness against VT-IPD [Citation37]. High-risk people (all ages) were assumed to have effectiveness equal to 80% of the corresponding values for low- and medium-risk persons. This assumption was based on a study by Klugman et al., in which PCV9 vaccine efficacy against VT-IPD among HIV-positive children was 65%, versus 83% among HIV-negative children (relative efficacy, 78%) [Citation39,Citation40].
Efficacy inputs for the initial period of effectiveness of PCV vaccines against VT-NBP for low- and medium-risk people were based on data for adults aged ≥65 years in the CAPiTA per-protocol population and from post hoc analyses of CAPiTA data for adults aged <65 years [Citation37]. For people aged ≥65 years with low or medium risk, initial vaccine effectiveness was assumed to be 45%, based on the overall study findings. For people aged 60 to 64 years with low or medium risk, initial vaccine effectiveness estimates were derived using age-specific relative changes in effectiveness against VT-NBP (versus age 65 years) [Citation37]. Initial vaccine effectiveness against VT-NBP for people of all ages with high risk was assumed equal to 80% of the corresponding values for low- and medium-risk persons, using a similar approach as for VT-IPD in the high-risk category [Citation39,Citation40].
2.2.6. Effectiveness of PPSV23
Methods to derive the effectiveness of PPSV23 against VT-IPD and VT-NBP were also described in the publication of Mendes et al. [Citation22]. Efficacy inputs for the initial period of effectiveness of PPSV23 vaccine against VT-IPD for low-, medium-, and high-risk persons was estimated using data from Djennad et al. [Citation41]. Initial effectiveness was derived for all ages by fitting a logarithmic curve to values for people aged 65 to 74, 75 to 84, and 85 to 99 years, respectively, from data reported in Djennad et al., and then allocating estimated age-specific values across groups using relative risks and corresponding population weights [Citation26,Citation41].
For the base case, efficacy inputs of PPSV23 against VT-NBP was assumed to be zero, based on various published sources and consistent with base-case assumptions used in several similar economic evaluation studies [Citation42–57]. A scenario analysis using data reported by Suzuki et al. was performed to test moderate effectiveness of PPSV23 against VT-NBP [Citation15].
2.2.7. Vaccine waning
For all PCV vaccines, initial vaccine effectiveness was assumed to persist for the first 5 years of the modeling time horizon for all ages and risk categories, which is consistent with CAPiTA, in which no waning of vaccine efficacy was observed in the 5-year follow-up period [Citation37]. Beyond year 5, vaccine effectiveness was assumed to wane across all age and risk groups by 5% annual decline during years 6 to 10, 10% annual decline during years 11 to 15, and an assumption of no efficacy from year 16 onwards [Citation38].
For PPSV23, vaccine effectiveness was assumed to wane across all age and risk groups, based on data reported by Djennad et al. as follows: linear decline to 76.2% of initial vaccine effectiveness by year 5, then linear decline to no efficacy by year 10 and through to the end of the modeling time horizon [Citation41].
2.2.8. Herd effects from pediatric PCV15 and PCV20 use
The percentage reduction in total disease due to indirect effects (herd effects) from pediatric pneumococcal vaccines was not considered in the base case. In scenario analyses, two scenarios testing herd effects from pediatric PCV15 and PCV20 use were explored using data from Mendes et al. and Japanese epidemiology data [Citation7,Citation22]. The method used to estimate herd effect from Japanese epidemiology data is described in the supplemental online material and values used in this scenario are summarized in Supplementary Table S2.
2.2.9. Long-term consequences
Long-term consequences due to all-cause NBP were not considered in the base-case analysis. Previous Japanese studies and cost-effectiveness analyses of PCV20 in other countries did not consider long-term consequences due to all-cause NBP [Citation21–23,Citation58]. Furthermore, it was concluded that it was difficult to set the rate of long-term consequences due to all-cause NBP, which would be influenced by antibiotic use and age. Including long-term consequences due to all-cause NBP, would lead to better cost-effectiveness results as PCV20 is anticipated to reduce the number of NBP cases and associated sequelae. As a conservative approach, we have not included this parameter due to lack of evidence and expected positive impact on the results [Citation22,Citation59,Citation60].
2.2.10. Mortality
Age-specific general population mortality rates were estimated using the number of Japanese population in 2022 from Japan statistics data (e-stat) and mortality rates by age and sex from Ministry of Health, Labour and Welfare (MHLW) [Citation26,Citation61].
Case fatality for IPD (bacteremia and meningitis) was based on a publication from Chang et al. [Citation62]. Case fatality for NBP (hospitalized) for adults aged 50 to 64 years and ≥65 years was sourced from Miyazaki et al. [Citation3]. Alternative values for IPD case fatality were available from Yanagihara et al. and explored in scenario analysis [Citation63]. One scenario for NBP case fatality was explored using values from two published papers (Konomura et al. and Tashiro et al.) [Citation64,Citation65].
2.2.11. Utilities
Age- and sex-specific EQ-5D-5L values to inform baseline utilities for a Japanese population were sourced from Shiroiwa et al. [Citation66]. Utility values in adults aged 50 to 64, 65 to 74, 75 to 84 and ≥85 years of age were calculated by weighting the Japanese population per age group [Citation26]. Annual utility decrements of 0.1741, 0.0434, and 0.0289 were applied for IPD, inpatient NBP, and outpatient NBP, respectively [Citation67,Citation68].
2.2.12. Vaccination and medical care costs
Vaccine acquisition costs were JPY4,735 and JPY7,200 for PPSV23 and PCV15, respectively [Citation69,Citation70]. As of January 2024, PCV20 has not been approved in Japan. Therefore, in the base-case analysis, we assumed a cost of JPY8,102, which was calculated by multiplying the cost of PCV13 by 1.13. In the absence of other data, a value of 1.13 was used, calculated based on the ratio of pediatric PCV20 cost (USD 178.00) and PCV13 cost (USD 158.18), with vaccination costs sourced from the US Centers for Disease Control [Citation71]. In scenario analysis, the vaccine cost for PCV20 was also assumed to be the same as PCV13 (JPY7,200), as the vaccine cost for PCV20 is anticipated to be similar to the cost of PCV13 or higher [Citation71]. A vaccine administration cost of JPY3,250 including initial consultation, injection, and biological preparations was applied, based on the MHLW published list [Citation72].
Medication costs per episode of each disease condition were derived using the JMDC Japanese claims database for adults aged <65 years and the MDV database for ages ≥65 years [Citation32,Citation33]. Details on costing methods are described in the supplemental online material and in Supplementary Table S3. Alternative costs reported in the studies by Fukuda et al. and Konomura et al. were explored in scenario analysis [Citation64,Citation73].
2.2.13. Costs related to productivity loss
Productivity losses were assumed to be incurred by all patients aged 60 to 64 years and by caregivers of patients aged ≥65 years in the base-case analysis. In scenario analysis, working rate and caregiver utilization rate were considered based on Japanese statistics (Population Estimates Annual Report 2022 and Labour Force Survey 2022) and MHLW data [Citation26,Citation74,Citation75]. Costs related to productivity loss were derived by multiplying the number of working days missed due to PDs and vaccination. The average daily wage of a patient and caregivers was calculated based on the monthly wage (20.4 working days per month assumed) and included an annual bonus compensation, both sourced from the Wage Structure Basic Statistical Survey [Citation76]. The number of days lost due to hospitalization, stratified by age and risk category, were obtained from Konomura et al. and JMDC data [Citation32,Citation64]. An assumption was made to add 3 additional days to work loss for hospitalization. The number of days lost by age and risk category due to outpatient care for all-cause NBP were obtained from Konomura et al., corresponding to the median number of prescription days of antibiotics [Citation64]. Two scenarios for the number of days lost due to outpatient care for all-cause NBP in adults aged 60 to 64 years and ≥65 years were explored: 5 days and 10 days. The number of days lost due to vaccination was assumed at 0.5 day.
2.3. Sensitivity and scenario analyses
Deterministic sensitivity analyses (DSA) were performed to evaluate the robustness of base case results to changes in key model parameters, including disease incidence, utility, mortality, vaccine efficacy, and costs. Probabilistic sensitivity analysis (PSA) was performed to estimate the level of uncertainty surrounding the model’s incremental cost and effectiveness results. These analyses are described in detail in the supplemental online material and PSA input parameters are summarized in Supplementary Table S4. The generated DSA results are presented in the form of a tornado diagram. The PSA results are presented on a cost-effectiveness plane. In addition to sensitivity analyses, scenario analyses were also conducted (described in Supplementary Table S5). The input parameters used in scenario analyses are presented in Supplementary Table S6.
3. Results
3.1. Base-case results
The base-case results using societal and payer perspectives are presented in and . For the comparison of PCV20 with PPSV23, assuming the PCV20 price of JPY8,102, there was an incremental QALY gain of 0.0015 per person, with reduced costs of JPY22,513 per person and JPY477 per person from a societal and payer perspective respectively ().
Table 2. Results of base case in 65-year-old adults and adults at high risk aged 60 to 64 years.
Table 3. Breakdown of health outcomes and costs in base case and scenario analysis 1 in 65-year-old adults and adults at high risk aged 60 to 64 years.
The calculated ICERs for the probabilistic analysis were all located the south-east quadrant of the cost-effectiveness plane, which indicates PCV20 was dominant (more effective and cost saving) compared with PPSV23. The INMB at a cost-effectiveness threshold of JPY5,000,000 per QALY was JPY30,205 and JPY8,170 using a societal and payer perspective respectively. The positive INMBs indicate that PCV20 was cost-effective compared with PPSV23 at this threshold using both analysis perspectives.
Compared with PPSV23 for the base-case population, PCV20 resulted in reduced numbers of bacteremia, meningitis, hospitalized NBP and outpatient NBP cases of 117, 10, 2,461 and 8,352 respectively (). Mortality for PCV20 was reduced by 226 cases compared to PPSV23. Medical costs for the population were reduced by JPY2.84 billion and productivity loss costs by JPY34.01 billion per population, offsetting the incremental vaccination costs (JPY2.10 billion per population). Breakdown of health outcomes and costs for the other populations tested in scenarios are shown in Supplementary Table S7.
At a PCV20 price of JPY7,200 (par with PCV13), PCV20 was dominant compared with PPSV23 (Supplementary Table S8) and more cost-saving than the base-case, as the incremental vaccination cost was JPY1.54 billion per population (). The cost-effectiveness results of scenarios examining the PCV20 price are provided in Supplementary Table S9. Furthermore, analyses comparing PCV20 with PCV15 and PCV15 with PPSV23 were found to be more effective and incurred less costs ( and Supplementary Table S8).
Threshold analysis was conducted to determine the price at which the PCV20 vaccine would return an ICER of JPY5,000,000 per QALY. From a societal perspective, a PCV20 unit cost of JPY82,867 was required (JPY74,765 higher than PCV20 cost used in the base case). From a payer perspective, a PCV20 unit cost of JPY28,324 was required (which is JPY20,222 higher than the cost of PCV20 used in the base-case analysis).
3.2. Scenarios and sensitivity analyses results (PCV20 vs PPSV23)
Detailed scenario analyses results are presented in and , and Supplementary Tables S10 to S13. Using a societal perspective, PCV20 was dominant (less costly and more effective) in all scenario analyses compared with PPSV23 at a cost-effectiveness threshold of JPY5,000,000 per QALY gained. PPSV23 NBP vaccine effectiveness (scenario 13), productivity loss (scenario 18), and vaccine coverage (scenario 6) had the highest impact on the analysis results. The INMB was JPY30,205 in the base case analysis versus JPY6,180 in scenario 13, JPY11,066 in scenario 18 and JPY14,953 in scenario 6. For the payer perspective, PCV20 was either dominant or cost-effective compared with PPSV23 at a cost-effectiveness threshold of JPY5,000,000 per QALY gained. PPSV23 NBP vaccine effectiveness (scenario 13), vaccine coverage (scenarios 6) and vaccine target population (scenario C) were the top three most impactful scenarios on the INMB.
Table 4. Scenario analysis (PCV20 vs PPSV23) with different vaccine target population.
Table 5. Scenario analysis (PCV20 vs PPSV23) in 65-year-old adults and adults at high risk aged 60 to 64 years.
The parameters with the greatest impact on INMB in the DSA when comparing PCV20 to PPSV23 were disease incidence of inpatient NBP; PCV vaccine effectiveness against NBP and inpatient NBP case fatality (see for detailed results).
Figure 2. Deterministic sensitivity analysis results: tornado diagram.
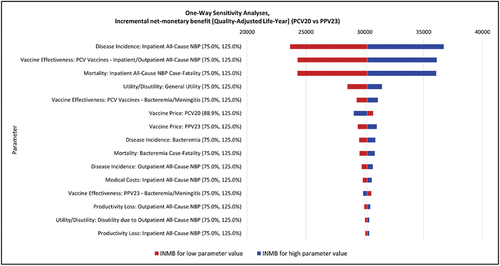
PCV20 was less costly and more effective (cost-saving) compared with PPSV23 in 100% of the 1,000 PSA simulations from a societal perspective (). The probability of PCV20 being cost-effective against PPSV23 was 100% at a cost-effectiveness threshold of JPY5,000,000 per QALY gained. PCV20 was less costly and more effective (cost-saving) compared with PPSV23 in 63% of the 1,000 PSA simulations using a payer perspective (). The remaining model simulations were in the northeast quadrant, indicating that PCV20 was more costly and more effective compared to PPSV23.
Figure 3. Probabilistic sensitivity analysis results for the cost-effectiveness plane (PCV20 vs PPSV23).
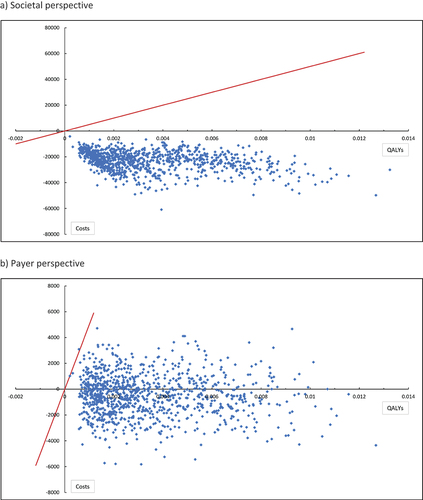
4. Discussion
We demonstrated that PCV20 would be a dominant strategy in comparison with PPSV23 (current NIP vaccine) from a societal perspective when the PCV20 price was set as JPY8,102 in adults aged 65 years and high-risk adults aged 60 to 64 years. Use of a payer perspective and other scenario analysis support this conclusion, where PCV20 was dominant or cost effective compared to PPSV23 at a cost-effectiveness threshold of JPY5,000,000 per QALY gained. PCV15 was also shown to be a dominant strategy in comparison with PPSV23, but when compared with PCV20, PCV20 was the dominant strategy because it provided greater health benefits at a lower cost than PCV15.
In Japan, PCV20 was submitted to PMDA in 2023 for approval. Therefore, the price of PCV20 has not yet been established as of January 2024. In this study, we used a cost of JPY8,102 for the base case and JPY7,200 (parity to PCV13) in scenario analysis. Sensitivity analysis revealed the price of PCV20 was one of the key drivers of the ICER. Therefore, threshold analysis was conducted and shows that using a higher PCV20 cost of JPY87,133 (societal perspective) or JPY28,324 (payer perspective) would still return an ICER below the JPY5,000,000 per QALY threshold. This result will be informative for discussions about PCV20 price and the NIP for PCV20.
During the COVID-19 pandemic, the incidence rate of PD reduced markedly due to several safety measures that were applied globally to curb the spread of coronavirus infection. For example, the incidence rate of IPD was 5.43 and 5.57 per 100,000 in 2018 and 2019 (before the COVID-19 pandemic), and 2.77 and 2.21 per 100,000 in 2020 and 2021 (during COVID-19 pandemic) in adults aged ≥65 years [Citation2]. Therefore, this study employed the incidence and serotype distribution data before the COVID-19 period (2017 to 2019) for the base-case analysis. In the scenario analysis that utilized a lower PD incidence and serotype distribution data during the COVID-19 era (2020 to 2022), the results showed that the base-case cost-effectiveness outcomes remained unchanged as a dominant strategy.
In addition to the adult PCV20 vaccine, the use of PCV20 for a pediatric population has been submitted for approval in Japan [Citation78]. Several reports suggest that pneumococcal vaccines exert an indirect effect (i.e. herd immunity) [Citation79]. Therefore, a scenario analysis examining herd immunity from use of pediatric PCV20 was considered by assuming that PCV20 would be widely used in the pediatric population (scenarios 14 and 15). These scenarios showed that PCV20 was still dominant compared with PPSV23. At present, only PCV13 is a NIP target for a pediatric population since 2013. In the model, we assumed that the proportion of IPD due to PCV13 serotypes should be assumed to have reached a steady state prior to model entry, and thus remain constant for the entirety of the modeling horizon. As the scenario we previously mentioned indicates, it is anticipated, however, that future pediatric use of PCV20 would result in a decline in the proportion of adult disease attributable to additional serotypes included in the novel vaccines.
In the base case, we assumed that PPSV23 had no effectiveness against NBP. A similar assumption was made in other studies evaluating PCV20 [Citation22,Citation58,Citation80,Citation81]. We used that assumption here because data to support the argument that PPSV23 affects NBP are inconsistent, and any inclusion of this effect would be uncertain and should be considered with caution [Citation15,Citation22,Citation50]. However, assuming effectiveness of PPSV23 against NBP does not change the conclusions of the analysis. Suzuki et al. reported that PPSV23 showed low to moderate effectiveness against vaccine serotype pneumococcal pneumonia in people aged 65 years or older [Citation11]. In the scenario analysis, we assumed that vaccine effectiveness of PPSV23 against NBP was 33.5% but PCV20 was still cost saving from a societal perspective and cost effective from a payer perspective at a cost-effectiveness threshold of JPY5,000,000 per QALY gained.
Our findings are consistent with a published study considering the use of PCV20 compared to PPSV23 in Japan. Hoshi et al. evaluated the cost-effectiveness of PCV20 and PCV15 compared with PPSV23 in an elderly Japanese population aged 65 years, using a payer perspective [Citation21]. Higher costs than those considered in the current study for PCV15 (JPY16,489) and PCV20 (JPY19,030) were used in that study, based on the US CDC vaccination costs [Citation71]. However, the results of that analysis found that the PCV20 vaccination was cost saving compared with PPSV23, supporting the findings of our analysis. Other key differences include different model structures (Hoshi et al. developed a decision tree linked to a Markov model, whereas our analysis used a Markov model structure) and use of a third-party payer perspective rather than a societal perspective [Citation21]. There was also a difference in key inputs, including the modeled population age (our analysis included adults aged 60 to 64 years who were at high risk), and Hoshi et al. did not stratify the model population by risk severity [Citation21]. The findings indicate that the introduction of PCV20 in adult NIP would be meaningful in Japan.
PCV20 has been approved in other jurisdictions, including the United Kingdom, Denmark, and Germany. Several publications evaluated PCV20 compared with the current standard of care, PPSV23, in these countries, using the same model structure as our analysis [Citation22,Citation58,Citation80,Citation81]. Despite differences in perspective, population age, and model inputs, each study found that PCV20 would be a cost saving and effective strategy compared with PPSV23. The findings of these studies support the argument for the introduction of PCV20 as a cost-saving alternative treatment in comparison to PPSV23.
The model analysis has several limitations, including data limitations. First, a comparison of PCV20 with a ‘no vaccine’ scenario was not considered in this analysis because several vaccines are already available, such as PPSV23, which is the current standard vaccine in the NIP. We considered this comparison would be non-informative given there are vaccines already used in practice.
Secondly, we made our best effort to use robust inputs estimates for Japan collected via targeted literature reviews. However, there were limited available data to inform several inputs, including vaccine history, caregiver work loss, percentage of NBP due to vaccine serotypes, and reduction in total disease, which was assumed to be zero. To test the uncertainty associated with these parameters, we ran scenario analyses; PCV20 remained the dominant strategy, suggesting it is likely to be cost saving in a Japanese healthcare setting.
Thirdly, we applied the risk distribution to the age groups without any adjustment to distribution and without taking real-world observations into account. We also used assumptions where data on several parameters, such as IPD case fatality, case fatality for hospitalized NBP, hospitalization cost data, productivity loss, and utility data, were available but incomplete for our target population because they were not specified by age group or risk stratification. To address data gaps, we assumed the same inputs were applicable across age groups and risk groups. However, we expect more granular data would have had little impact on the results, given the robustness of the analysis findings.
Additionally, there is uncertainty regarding vaccine effectiveness. Vaccine effectiveness estimates of all PCVs were based on studies showing non-inferior immunogenicity comparing PCV13 or PPSV23 [Citation17,Citation82]. Therefore, PCV20 and PCV15 effectiveness against PD was assumed to be the same as PCV13 effectiveness against PD in the CAPiTA trial [Citation37]. In clinical practice, PCV20 and PCV15 effectiveness may differ from PCV13 effectiveness. To examine this, we tested several scenarios, which had minimal impact on results.
Finally, given that economic models are a simplification of reality, we could not add all relevant inputs, and we did not include long-term clinical costs and consequences of PD, although evidence shows that these sequelae are common and costly [Citation83–87]. Likewise, we did not consider adverse events, given that these are expected to be rare and similar across all vaccines. Additionally, because the price for PCV20 in Japan is unknown, we assumed that the cost of PCV20 in Japan would be JPY3,367 higher than that of PPSV23. The analysis showed that even at the assumed higher cost, PCV20 was a cost-saving strategy compared with PPSV23.
5. Conclusions
Our analysis supports the case for the introduction of PCV20 in Japan, with the findings demonstrating that PCV20 is both cost saving and more effective than currently available vaccines for all adults aged 65 years and those aged 60 to 64 years at high risk. Given the prolonged duration of protection and greater effectiveness associated with PCV20, the introduction of PCV20 has the potential to substantially reduce healthcare burden and positively affect morbidity and mortality related to PD in Japan.
Declaration of interest
S Nakamura declares that he receives honoraria from Pfizer Japan Inc., for delivering promotional lectures and a consultation fee. M Mikami, T Hayamizu, N Yonemoto, and K Kamei are employees of Pfizer Japan Inc., and J Vietri is an employee of Pfizer Inc. C Crossan, C Moyon, M Gouldson, Y Onishi, M Nomoto, and F Khrouf are employees of Putnam. Putnam was paid by Pfizer Japan Inc. for conducting the study and medical writing support. The authors have no other relevant affiliations or financial involvement with any organization or entity with a financial interest in or financial conflict with the subject matter or material discussed in the manuscript apart from those disclosed.
Reviewer disclosures
Peer reviewers on this manuscript have no relevant financial or other relationships to disclose.
Author contributions
All authors have substantially contributed to the conception, design, and execution of this study. In addition, all authors have substantially contributed to the analysis and interpretation of the study findings. Finally, all authors have read and agreed to the published version of the manuscript.
Supplemental Material
Download MS Word (385.9 KB)Acknowledgments
The authors would like to thank Ataru Igarashi of Department of Public Health, Yokohama City University School of Medicine, and Department of Health Economics and Outcomes Research, Graduate School of Pharmaceutical Sciences, The University of Tokyo for providing valuable scientific advice. The authors also thank Yoshie Onishi, Mariko Nomoto, and Fatma Khrouf of Putnam, who conducted targeted literature reviews and desk research to inform model input parameters for Japanese healthcare setting.
Supplementary material
Supplemental data for this article can be accessed online at https://doi.org/10.1080/14760584.2024.2350246
Additional information
Funding
References
- World Health Organization. Weekly Epidemiological Record - considerations for pneumococcal vaccination in older adults. Geneva: World Health Organization; 2021 [2023 Aug 7]. Available from: https://apps.who.int/iris/bitstream/handle/10665/341721/WER9623-eng-fre.pdf
- National Institute of Infectious Diseases. Infectious Agents Surveillance Report (IASR) - pathogenic microorganism detection information 2023. [2023 Nov 9]. Available from: https://www.niid.go.jp/niid/images/idsc/iasr/44/515.pdf
- Miyazaki T, Hirano K, Ichihara K, et al. Community-acquired pneumonia incidence in adults 18 years of age and older in Goto City, Japan: a prospective, population-based study. CHEST Pulmonary. 2023;1(2):100007. doi: 10.1016/j.chpulm.2023.100007
- Portal sSte of Official Statistics of Japan. Current population estimates. 2022 [2023 Nov 9]. Available from: https://www.stat.go.jp/english/data/jinsui/2022np/index.html#:~:text=The%20population%20under%2015%20years,percent%20of%20the%20total%20population
- Ministry of Health Labour and Welfare website. About pneumococcal vaccine PPSV23. 2018 [2023 Aug 8]. Available from: https://www.mhlw.go.jp/content/10601000/000342164.pdf
- Ministry of Health Labour and Welfare website. 58th health sciences council immunisation - basic Immunisation policy subcommittee of the subcommittee on vaccines document. 2020 [2024 Jan]. Available from: https://www.mhlw.go.jp/stf/newpage_36952.html
- Epidemiological information on invasive pneumococcal infections in children and adults. Current serotype distribution of adult IPD-derived pneumococci 2022. [2023 Aug 14]. Available from: https://ipd-information.com/?page_id=49
- Kawakami K, Nakamura A, Wakana A, et al. A Japanese nationwide survey of 23-valent pneumococcal capsular polysaccharide vaccine (PPSV23) coverage among patients with chronic medical condition aged 50 and older. Hum Vaccin Immunother. 2020 Jul 2;16(7):1521–1528. doi: 10.1080/21645515.2019.1690332
- National Institute of Infectious Diseases. Infectious Agents Surveillance Report - pathogenic microorganism detection information 2018. [2023 Aug 16]. Available from: https://www.niid.go.jp/niid/images/idsc/iasr/39/461.pdf
- Ministry of Health Labour and Welfare - Health Insurance Bureau. Number of people receiving regular vaccinations 2021. [2024 Jan 5]. Available from: https://www.mhlw.go.jp/topics/bcg/other/5.html
- Pollard AJ, Perrett KP, Beverley PC. Maintaining protection against invasive bacteria with protein-polysaccharide conjugate vaccines. Nat Rev Immunol. 2009 Mar;9(3):213–220. doi: 10.1038/nri2494
- Leidner AJ. Summary of three economic models evaluating pneumococcal vaccines in adults in the United States. In: ACIP Meeting: Centers for Disease Control and Prevention; 2021 [2024 Jan 4]. Available from: https://www.cdc.gov/vaccines/acip/meetings/downloads/slides-2021-09-29/02-Pneumococcal-Leidner-508.pdf
- National Institute of Infectious Diseases. 23-valent pneumococcal polysaccharide vaccine fact sheet. 2018 [2023 Aug 14]. Available from: https://www.mhlw.go.jp/file/05-Shingikai-10601000-Daijinkanboukouseikagakuka-Kouseikagakuka/0000184910_1.pdf
- Patterson S, Webber C, Patton M, et al. A post hoc assessment of duration of protection in CAPiTA (Community Acquired Pneumonia immunization Trial in Adults). Trials Vaccinol. 2016;5:92–96. doi: 10.1016/j.trivac.2016.04.004
- Suzuki M, Dhoubhadel BG, Ishifuji T, et al. Serotype-specific effectiveness of 23-valent pneumococcal polysaccharide vaccine against pneumococcal pneumonia in adults aged 65 years or older: a multicentre, prospective, test-negative design study. Lancet Infect Dis. 2017;17(3):313–321. doi: 10.1016/S1473-3099(17)30049-X
- Application for manufacturing and sales approval for precipitated 20-valent pneumococcal conjugate vaccine. Pfizer Inc.; 2023. Available from: https://www.pfizer.co.jp/pfizer/company//press/2023/2023-09-13-02.
- Essink B, Sabharwal C, Cannon K, et al. Pivotal Phase 3 Randomized Clinical Trial of the Safety, Tolerability, and Immunogenicity of 20-Valent Pneumococcal Conjugate Vaccine in Adults Aged ≥18 Years. Clin Infect Dis. [2022 Aug 31];75(3):390–398. doi: 10.1093/cid/ciab990
- Haranaka M, Young Song J, Huang KC, et al. A phase 3 randomized trial of the safety and immunogenicity of 20-valent pneumococcal conjugate vaccine in adults ≥ 60 years of age in Japan, South Korea, and Taiwan. Vaccine. 2024 Jan 23;42(5):1071–1077. doi: 10.1016/j.vaccine.2024.01.004
- Hurley D, Griffin C, Young M, et al. Safety, Tolerability, and Immunogenicity of a 20-Valent Pneumococcal Conjugate Vaccine (PCV20) in Adults 60 to 64 Years of Age. Clin Infect Dis. 2021 Oct 5;73(7):e1489–e1497. doi: 10.1093/cid/ciaa1045
- Klein NP, Peyrani P, Yacisin K, et al. A phase 3, randomized, double-blind study to evaluate the immunogenicity and safety of 3 lots of 20-valent pneumococcal conjugate vaccine in pneumococcal vaccine-naive adults 18 through 49 years of age. Vaccine. 2021 Sep 7;39(38):5428–5435. doi: 10.1016/j.vaccine.2021.07.004
- Hoshi SL, Shono A, Seposo X, et al. Cost-effectiveness analyses of 15- and 20-valent pneumococcal conjugate vaccines for Japanese elderly. Vaccine. 2022 Nov 22;40(49):7057–7064. doi: 10.1016/j.vaccine.2022.10.010
- Mendes D, Averin A, Atwood M, et al. Cost-effectiveness of using a 20-valent pneumococcal conjugate vaccine to directly protect adults in England at elevated risk of pneumococcal disease. Expert Rev Pharmacoecon Outcomes Res. 2022 Dec;22(8):1285–1295. doi: 10.1080/14737167.2022.2134120
- Igarashi A, Hirose E, Kobayashi Y, et al. Cost-effectiveness analysis for PCV13 in adults 60 years and over with underlying medical conditions which put them at an elevated risk of pneumococcal disease in Japan. Expert Rev Vaccines. 2021 Sep;20(9):1153–1165. doi: 10.1080/14760584.2021.1952869
- Ikeda T. Research guidelines for evaluating the cost-effectiveness of vaccinations. Tokyo: Ministry of Health Labour and Welfare; 2017 [2024 Jan 4]. Available from: https://www.mhlw.go.jp/file/05-Shingikai-10601000-Daijinkanboukouseikagakuka-Kouseikagakuka/0000184902_1.pdf
- Ministry of Health Labour and Welfare - Health Insurance Bureau. Cost-effectiveness evaluation of drugs, medical devices and regenerative medicine products. 2019 [2023 Nov 23]. Available from: https://www.mhlw.go.jp/content/000497467.pdf
- Portal Site of Official Statistics of Japan. Population Estimates Annual Report 2022. [2023 Aug 16]. Available from: https://www.e-stat.go.jp/en/stat-search/files?page=1&layout=datalist&toukei=00200524&tstat=000000090001&cycle=7&tclass1=000001011679&tclass2val=0
- Imai K, Petigara T, Kohn MA, et al. Risk of pneumococcal diseases in adults with underlying medical conditions: a retrospective, cohort study using two Japanese healthcare databases. BMJ Open. 2018 Mar 2;8(3):e018553. doi: 10.1136/bmjopen-2017-018553
- The JRS guidelines for the management of community acquired pneumonia in adults. Nihon Kokyuki Gakkai Zasshi. 2007 Jan 15;Suppl:2–85. https://pubmed.ncbi.nlm.nih.gov/17290540/
- Bridges CB, Coyne-Beasley T, Advisory Committee on Immunization Practices. Advisory committee on immunization practices recommended immunization schedule for adults aged 19 years or older: United States, 2014. Ann Intern Med. 2014 Feb 4;160(3):190. doi: 10.7326/M13-2826
- Joint Committee on Vaccination and Immunisation. Statement on the wider use of pneumococcal conjugate vaccines in the UK. 2013 [cited 2023 Oct 11]. Available from: https://assets.publishing.service.gov.uk/government/uploads/system/uploads/attachment_data/file/224765/JCVI_statement_on_pneumococcal_vaccination_for_clinical_risk_groups_Final.pdf
- Ministry of Health Labour and Welfare website. Amendment of code of practice for immunization. 2014 [cited 2023 Dec 18]. Available from: https://www.mhlw.go.jp/file/06-Seisakujouhou-10900000-Kenkoukyoku/0000121144.pdf
- JMDC Inc. JMDC Claims Database. [cited 2023 Aug 11]. Available from: https://www.jmdc.co.jp/en/
- Medical Data Vision Co. MDV Database. [cited 2023 Nov 9]. Available from: https://en.mdv.co.jp/
- National Institute of Infectious Diseases. Surveillance data - summary table list 2021. [2023 Nov 9]. Available from: https://www.niid.go.jp/niid/ja/allarticles/surveillance/2270-idwr/nenpou/11638-syulist2021.html
- Kobayashi K, Jo T, Mimura W, et al. Interrupted time-series analyses of routine vaccination program for elderly pneumonia patients in Japan; an ecological study using aggregated nationwide inpatient data. Hum Vaccin Immunother. 2021;17(8):2661–2669. doi: 10.1080/21645515.2021.1875760
- Nakashima K, Suzuki K, Aoshima M, et al. Effectiveness of the 23-valent pneumococcal polysaccharide vaccine against community-acquired pneumonia in older individuals after the introduction of childhood 13-valent pneumococcal conjugate vaccine: A multicenter hospital-based case-control study in Japan. Vaccine. 2022 Nov 2;40(46):6589–6598. doi: 10.1016/j.vaccine.2022.09.055
- Bonten MJ, Huijts SM, Bolkenbaas M, et al. Polysaccharide conjugate vaccine against pneumococcal pneumonia in adults. N Engl J Med. 2015 Mar 19;372(12):1114–1125. doi: 10.1056/NEJMoa1408544
- Mangen MJ, Rozenbaum MH, Huijts SM, et al. Cost-effectiveness of adult pneumococcal conjugate vaccination in the Netherlands. Eur Respir J. 2015 Nov;46(5):1407–1416. doi: 10.1183/13993003.00325-2015
- French N, Gordon SB, Mwalukomo T, et al. A trial of a 7-valent pneumococcal conjugate vaccine in HIV-infected adults. N Engl J Med. 2010 Mar 4;362(9):812–822. doi: 10.1056/NEJMoa0903029
- Klugman KP, Madhi SA, Huebner RE, et al. A trial of a 9-valent pneumococcal conjugate vaccine in children with and those without HIV infection. N Engl J Med. 2003;349(14):1341–1348. doi: 10.1056/NEJMoa035060
- Djennad A, Ramsay ME, Pebody R, et al. Effectiveness of 23-Valent Polysaccharide Pneumococcal Vaccine and Changes in Invasive Pneumococcal Disease Incidence from 2000 to 2017 in Those Aged 65 and Over in England and Wales. EClinicalMedicine. 2018 Dec;6:42–50. doi: 10.1016/j.eclinm.2018.12.007
- Boccalini S, Bechini A, Levi M, et al. Cost-effectiveness of new adult pneumococcal vaccination strategies in Italy. Hum Vaccin Immunother. 2013 Mar;9(3):699–706. doi: 10.4161/hv.23268
- Cho BH, Stoecker C, Link-Gelles R, et al. Cost-effectiveness of administering 13-valent pneumococcal conjugate vaccine in addition to 23-valent pneumococcal polysaccharide vaccine to adults with immunocompromising conditions. Vaccine. 2013 Dec 5;31(50):6011–6021. doi: 10.1016/j.vaccine.2013.10.024
- Evers SM, Ament AJ, Colombo GL, et al. Cost-effectiveness of pneumococcal vaccination for prevention of invasive pneumococcal disease in the elderly: an update for 10 Western European countries. Eur J Clin Microbiol Infect Dis. 2007 Aug;26(8):531–540. doi: 10.1007/s10096-007-0327-z
- Fry AM, Zell ER, Schuchat A, et al. Comparing potential benefits of new pneumococcal vaccines with the current polysaccharide vaccine in the elderly. Vaccine. 2002 Dec 13;21(3–4):303–311. doi: 10.1016/S0264-410X(02)00451-6
- Heo JY, Seo YB, Choi WS, et al. Cost-effectiveness of pneumococcal vaccination strategies for the elderly in Korea. PLOS ONE. 2017;12(5):e0177342. doi: 10.1371/journal.pone.0177342
- Hoshi SL, Kondo M, Okubo I, et al. Economic Evaluation of Immunisation Programme of 23-Valent Pneumococcal Polysaccharide Vaccine and the Inclusion of 13-Valent Pneumococcal Conjugate Vaccine in the List for Single-Dose Subsidy to the Elderly in Japan. PLOS ONE. 2015;10(10):e0139140. doi: 10.1371/journal.pone.0139140
- Johnstone J, Marrie TJ, Eurich DT, et al. Effect of pneumococcal vaccination in hospitalized adults with community-acquired pneumonia. Arch Intern Med. 2007 Oct 8;167(18):1938–1943. doi: 10.1001/archinte.167.18.1938
- Kuhlmann A, Theidel U, Pletz MW, et al. Potential cost-effectiveness and benefit-cost ratios of adult pneumococcal vaccination in Germany. Health Econ Rev. 2012 Mar 30;2(1):4. doi: 10.1186/2191-1991-2-4
- Lawrence H, Pick H, Baskaran V, et al. Effectiveness of the 23-valent pneumococcal polysaccharide vaccine against vaccine serotype pneumococcal pneumonia in adults: A case-control test-negative design study. PLOS Med. 2020;17(10):e1003326. doi: 10.1371/journal.pmed.1003326
- Moberley S, Holden J, Tatham DP, et al. Vaccines for preventing pneumococcal infection in adults. Cochrane Database Syst Rev. 2013 Jan 31;2013(1):CD000422. doi: 10.1002/14651858.CD000422.pub3
- Ortqvist A, Hedlund J, Burman LA, et al. Randomised trial of 23-valent pneumococcal capsular polysaccharide vaccine in prevention of pneumonia in middle-aged and elderly people. Swedish Pneumococcal Vaccination Study Group. Lancet. 1998 Feb 7;351(9100):399–403. doi: 10.1016/S0140-6736(97)07358-3
- Simberkoff MS, Cross AP, Al-Ibrahim M, et al. Efficacy of pneumococcal vaccine in high-risk patients. Results of a Veterans Administration Cooperative Study. N Engl J Med. 1986 Nov 20;315(21):1318–1327. doi: 10.1056/NEJM198611203152104
- Sisk JE, Whang W, Butler JC, et al. Cost-effectiveness of vaccination against invasive pneumococcal disease among people 50 through 64 years of age: role of comorbid conditions and race. Ann Intern Med. 2003 Jun 17];138(12):960–968. doi: 10.7326/0003-4819-138-12-200306170-00007
- Smith KJ, Wateska AR, Nowalk MP, et al. Modeling of Cost Effectiveness of Pneumococcal Conjugate Vaccination Strategies in U.S. Older Adults. Am J Prev Med. 2013 Apr;44(4):373–381. doi: 10.1016/j.amepre.2012.11.035
- Stoecker C, Kim L, Gierke R, et al. Incremental Cost-Effectiveness of 13-valent Pneumococcal Conjugate Vaccine for Adults Age 50 Years and Older in the United States. J Gen Intern Med. 2016 Aug;31(8):901–908. doi: 10.1007/s11606-016-3651-0
- Stoecker C, Kobayashi M, Matanock A, et al. Cost-effectiveness of continuing pneumococcal conjugate vaccination at age 65 in the context of indirect effects from the childhood immunization program. Vaccine. 2020 Feb 11;38(7):1770–1777. doi: 10.1016/j.vaccine.2019.12.029
- Kuhne F, Achtert K, Puschner F, et al. Cost-effectiveness of use of 20-valent pneumococcal conjugate vaccine among adults in Germany. Expert Rev Vaccines. 2023 Jan;22(1):921–932. doi: 10.1080/14760584.2023.2262575
- Cantarero D, Ocana D, Onieva-Garcia MA, et al. Cost-utility analysis of the use of the 20-valent anti-pneumococcal vaccine (PCV20) in adults older than 60 years in Spain. Vaccine. 2023 Aug 14;41(36):5342–5349. doi: 10.1016/j.vaccine.2023.07.016
- Marbaix S, Mignon A, Taelman A, et al. Cost-utility of 20-valent pneumococcal conjugate vaccine compared to no vaccination and recommended alternative vaccines among Belgian adults. Expert Rev Vaccines. 2023 Jan;22(1):1008–1021. doi: 10.1080/14760584.2023.2273892
- Ministry of Health Labour and Welfare website. Overview of the 2020 simplified life table 2020. [2023 Sep]. Available from: https://www.mhlw.go.jp/toukei/saikin/hw/life/life22/index.html
- Chang B, Tamura K, Fujikura H, et al. Pneumococcal meningitis in adults in 2014-2018 after introduction of pediatric 13-valent pneumococcal conjugate vaccine in Japan. Sci Rep. 2022 Feb 23;12(1):3066. doi: 10.1038/s41598-022-06950-w
- Yanagihara K, Kosai K, Mikamo H, et al. Serotype distribution and antimicrobial susceptibility of streptococcus pneumoniae associated with invasive pneumococcal disease among adults in Japan. Int J Infect Dis. 2021 Jan;102:260–268. doi: 10.1016/j.ijid.2020.10.017
- Konomura K, Nagai H, Akazawa M. Economic burden of community-acquired pneumonia among elderly patients: a Japanese perspective. Pneumonia (Nathan). 2017;9(1):19. doi: 10.1186/s41479-017-0042-1
- Tashiro M, Fushimi K, Takazono T, et al. A mortality prediction rule for non-elderly patients with community-acquired pneumonia. BMC Pulm Med. 2016 Mar 8;16(1):39. doi: 10.1186/s12890-016-0199-z
- Shiroiwa T, Fukuda T, Ikeda S, et al. Japanese population norms for preference-based measures: EQ-5D-3L, EQ-5D-5L, and SF-6D. Qual Life Res. 2016 Mar;25(3):707–719. doi: 10.1007/s11136-015-1108-2
- Blommaert A, Hanquet G, Willem L, et al. Use of pneumococcal vaccines in the elderly: an economic evaluation (short report). KCE; 2016 [cited 2023 Dec 18]. Available from: https://kce.fgov.be/sites/default/files/2021-11/KCE_274C_Short-Report_Pneumococcal.pdf
- Glick HA, Miyazaki T, Hirano K, et al. One-Year Quality of Life Post-Pneumonia Diagnosis in Japanese Adults. Clin Infect Dis. [2021 Jul 15];73(2):283–290. doi: 10.1093/cid/ciaa595
- Ministry of Health Labour and Welfare. About pneumococcal vaccines for children 2023. [2024 Jan 4]. Available from: https://www.mhlw.go.jp/content/10900000/001139474.pdf
- Ministry of Health Labour and Welfare website. NHI price revisions - partial revision of drug prices (medicine price standards) for drugs used. 2023 Oct 11. Available from: https://www.mhlw.go.jp/content/12404000/001065988.pdf
- Centers for Disease Control and Prevention website. Vaccines for Children Program (VFC). CDC Vaccine Price List. 2023 [2023 Nov 9]. Available from: https://www.cdc.gov/vaccines/programs/vfc/awardees/vaccine-management/price-list/index.html
- Ministry of Health Labour and Welfare website. Methods for cost-effectiveness estimation for vaccination - medical fees 2022. [2023 Aug 8]. Available from: https://www.mhlw.go.jp/content/12404000/000907834.pdf
- Fukuda H, Onizuka H, Nishimura N, et al. Risk factors for pneumococcal disease in persons with chronic medical conditions: Results from the LIFE Study. Int J Infect Dis. 2022 Mar;116:216–222. doi: 10.1016/j.ijid.2021.12.365
- Portal Site of Official Statistics of Japan. Labour force survey 2022. [2023 Aug 16]. Available from: https://www.stat.go.jp/data/roudou/sokuhou/nen/ft/pdf/index1.pdf
- Ministry of Health Labour and Welfare website. Number of people certified for long-term Care/Support need. 2021 [2023 Sep]. Available from: https://www.mhlw.go.jp/topics/kaigo/osirase/jigyo/20/dl/r02_gaiyou.pdf
- Portal Site of Official Statistics of Japan. Wage Structure Basic Statistical Survey (general worker/Industrial classification) 2022. [2023 Oct 11]. Available from: https://www.e-stat.go.jp/stat-search/files?page=1&layout=datalist&toukei=00450091&tstat=000001011429&cycle=0&tclass1=000001202310&tclass2=000001202312&tclass3=000001202328&tclass4val=0
- Morimoto K, Suzuki M, Ishifuji T, et al. The burden and etiology of community-onset pneumonia in the aging Japanese population: a multicenter prospective study. PLOS ONE. 2015;10(3):e0122247. doi: 10.1371/journal.pone.0122247
- Pfizer Inc. Application for manufacturing and sales approval for precipitated 20-valent pneumococcal conjugate vaccine. 2023 [2023 Dec 19]. Available from: https://www.pfizer.co.jp//pfizer/company/press/2023/2023-09-13-02
- National Institute of Infectious Diseases. Fact sheet on pneumococcal conjugate vaccine for children. Ministry of Health Labour and Welfare. 2010 [2023 Aug 16]. Available from: https://www.mhlw.go.jp/file/05-Shingikai-10601000-Daijinkanboukouseikagakuka-Kouseikagakuka/0000207087_1.pdf
- Malene BM, Oyvind H, Tor M, et al. Cost-effectiveness of 20-valent pneumococcal conjugate vaccine compared with 23-valent pneumococcal polysaccharide vaccine among adults in a Norwegian setting. Cost Eff Resour Alloc. 2023 Aug 9;21(1):52. doi: 10.1186/s12962-023-00458-4
- Olsen J, Schnack H, Skovdal M, et al. Cost-effectiveness of 20-valent pneumococcal conjugate vaccine in Denmark compared with PPV23. J Med Econ. 2022 Jan;25(1):1240–1254. doi: 10.1080/13696998.2022.2152235
- Mohapi L, Pinedo Y, Osiyemi O, et al. Safety and immunogenicity of V114, a 15-valent pneumococcal conjugate vaccine, in adults living with HIV. AIDS. 2022 Mar 1;36(3):373–382. doi: 10.1097/QAD.0000000000003126
- Bornheimer R, Shea KM, Sato R, et al. Risk of exacerbation following pneumonia in adults with heart failure or chronic obstructive pulmonary disease. PLOS ONE. 2017;12(10):e0184877. doi: 10.1371/journal.pone.0184877
- Campling J, Jones D, Chalmers J, et al. Clinical and financial burden of hospitalised community-acquired pneumonia in patients with selected underlying comorbidities in England. BMJ Open Respir Res. 2020 Oct;7(1):e000703. doi: 10.1136/bmjresp-2020-000703
- Corrales-Medina VF, Alvarez KN, Weissfeld LA, et al. Association between hospitalization for pneumonia and subsequent risk of cardiovascular disease. JAMA. 2015 Jan 20;313(3):264–274. doi: 10.1001/jama.2014.18229
- Hughes GJ, Wright LB, Chapman KE, et al. Serotype-specific differences in short- and longer-term mortality following invasive pneumococcal disease. Epidemiol Infect. 2016 Sep;144(12):2654–2669. doi: 10.1017/S0950268816000856
- Myint PK, Hawkins KR, Clark AB, et al. Long-term mortality of hospitalized pneumonia in the EPIC-Norfolk cohort. Epidemiol Infect. 2016 Mar;144(4):803–809. doi: 10.1017/S0950268815001971