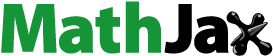
Abstract
To utilize the radical scavenging property of fullerene (C60), hydrophilic poly(ethylene glycol) (PEG) was chemically attached by Cu(I)-catalyzed azide-alkyne cycloaddition (CuAAC). The resulting C60-PEG derivatives were soluble in methanol when the molecular weight of PEG was 5,000. The antioxidative activity was evaluated for the alcohol-soluble C60 derivative. The C60-PEG has sufficient antioxidative activity with the β-carotene-attributed chromaticity of 76% for the C60-PEG derivative (9.96 mM). Further chemical modification will produce water-soluble fullerenes with higher antioxidative activities.
1. Introduction
The radical scavenging property of fullerene (C60) has attracted much attention with a view to commercialize the product by blending it into cosmetics.[Citation1] However, the hydrophobic feature of C60 makes it difficult to homogeneously distribute in an aqueous solution, which also hinders application studies of forming biopolymer complexes. In order to enhance the hydrophilicity and water solubility, chemical modification has been often adopted. For example, water-soluble fullerenes with numerous hydroxyl groups on their surface have been developed and given the trademark name “radical sponge,” and they have been proven to show excellent radical scavenging properties.[Citation2] Therefore, cosmetics containing hydroxylated fullerenes are already available on the market, and they promote anti-aging and whitening effects on the skin. It is generally said that water-soluble fullerenes have approximately 170 times the antioxidant effect of vitamin C. However, in the chemical structure of the “radical sponge,” a part of the C60 conjugated system disappeared due to the introduction of hydroxyl groups, and it is thought that the properties of fullerenes, represented by their antioxidant properties, accordingly decreased. To solve this problem, a more controlled chemical modification of fullerenes can be adopted.[Citation3] For example, it is known that the water solubility issue can be solved by substituting a giant water-soluble (high) molecular weight chain into a fullerene. However, a new problem arises: as the molecular weight of the substituent increases, the reactivity of the polymer terminal functional group generally decreases and the fullerene unit density in the resulting water-soluble fullerene derivative also decreases. In this study, we decided to control the solubility balance and selected the Cu(I)-catalyzed azide-alkyne cycloaddition (CuAAC) click chemistry reaction to introduce a single poly(ethylene glycol) (PEG) chain, a water-soluble polymer, to C60. The applicability of CuAAC to C60 was previously demonstrated.[Citation4] The resulting PEGylated C60 has the good solubility in methanol, depending on the length of the PEG chain. Finally, the antioxidant activity of the obtained PEG-fullerene derivative was evaluated. Since the antioxidants in natural products include not only water-soluble substances but also many lipophilic components, it is significant to evaluate the antioxidant activity of various synthetic materials.
2. Experimental section
2.1. General procedures
All chemicals were purchased from Kanto Chemical Co., TCI, and Aldrich and used without further purification. Nuclear magnetic resonance (NMR) spectra were recorded using a JEOL model AL300 (300 MHz) at room temperature. Deuterated chloroform was used as the solvent. Chemical shifts of NMR were reported in ppm (parts per million) relative to the residual solvent peak at 7.26 ppm for 1H NMR spectroscopy. Coupling constants (J) were given in Hz. The resonance multiplicity was described as s (singlet), br s (broad singlet), and m (multiplet). Fourier transform infrared (FT-IR) spectra were recorded by a JASCO FT/IR-4200 spectrometer in the range from 4000 to 600 cm−1. MALDI − TOF mass spectra were measured on a Shimadzu/Kratos AXIMACFR mass spectrometer equipped with a nitrogen laser (λ = 337 nm) and pulsed ion extraction, which was operated in a linear-positive ion mode at an accelerating potential of 20 kV. Tetrahydrofuran (THF) solutions containing 1 g L−1 of a sample, 10 g L−1 of dithranol, and 1 g L−1 of sodium trifluoroacetate were mixed to a ratio of 1:1:1; and then 1 μL aliquot of this mixture was deposited onto a sample target plate. UV–vis absorption spectra were recorded on a JASCO V-670 spectrophotometer.
The antioxidative property of the C60 derivative was evaluated according to a literature procedure.[Citation7,Citation8] First, a methanol solution of the C60 derivative was prepared at the concentration of 0.0023 M (solution A). Next, a chloroform solution of β-carotene (1 g L−1) (solution B), a chloroform solution of linoleic acid (0.1 g mL−1) (solution C), and a chloroform solution of Tween 40 (0.2 g mL−1) (solution D) were prepared. The solution B (0.25 mL), solution C (0.1 mL), and solution D (0.5 mL) were placed into a sample bottle, and chloroform was evaporated by spaying nitrogen gas. Pure water (50 mL) and 0.2 M phosphate buffer (4.45 mL) were added, and the mixture was stirred at room temperature for 30 min in the dark (solution E). To a UV cuvette, the solution E (2.85 mL) and a fixed amount of solution A were added. The absorbance of β-carotene at 470 nm was tracked over time with stirring at 50 °C. The relative intensity of 470 nm absorption was calculated according to the following EquationEquation (1)(1)
(1) :
(1)
(1)
2.2. Synthetic procedures
PEGylated C60 was prepared by CuAAC of the C60 derivative 15 and PEG-N3. To a 50 mL round bottle flask, 1 (24 mg, 0.022 mmol) and THF (7.2 mL) were added and the solution was stirred at 0 °C. After a THF solution of (nC4H9)4NF (1 M, 0.055 mL) was added, the solution was further stirred at 1.5 h at 0 °C. The solution was gradually heating to room temperature, and brine (200 mL) was added. Organic layers were extracted with CHCl3 (400 mL), washed with brine (200 mL × 2), and dried over Na2SO4. After filtration, the filtrate was concentrated to approximately 15 mL. To this solution, α-methoxy-ω-azide-poly(ethylene glycol) (Mn 5,000, 111 mg, 0.0222 mmol)) and Cu(PPh3)3Br (2.06 mg, 0.00221 mmol) were added and the mixture was stirred under N2 at 60 °C for 22 h. After cooling to room temperature, chloroform, and water were added. The organic layer was washed with water, dried over Na2SO4, and filtered. Evaporation and column chromatography (Bio-Beads S-X2, chloroform) afforded the target compound (89 mg, 68%). The resulting PEGylated C60 was soluble in methanol but limited solubility in water at 20–37 °C.
1H NMR (CDCl3, 300 MHz): δ = 7.68 ppm (br s, 1H), 4.29 (br s, 4H), 3.44–3.80 (m, 4(n + 1)H), 3.37 (s, 3H), 1.8–1.6 (m, 4H). IR (neat): 3243.7, 2884.0, 2130.0, 1717.3, 1591.0, 1483.0, 1466.6, 1451.2, 1358.6, 1342.2, 1280.5, 1241.0, 1144.6, 1105.0, 1060.7, 1006.7, 962.3, 879.4, 841.8, 717.4, 700.0, 664.4 cm−1.
3. Results and discussion
C60 derivative 1 was synthesized using the Bingel reaction.[Citation5] After deprotection of the trimethylsilyl groups of 1, one equivalent of methoxy-terminated polyethylene glycol (PEG) azide was added in the presence of a copper catalyst for cycloaddition reaction (the CuAAC click reaction) (Scheme 1). The unreacted starting material and bis-PEG adduct were removed by column chromatography on Bio-Beads SX-2, and the target product of mono-PEGylated fullerenes was isolated. The solubility of the fullerene derivatives varied greatly depending on the molecular weight of the PEG chain: C60-PEG2000 was soluble in organic solvents, such as chloroform and toluene, but insoluble in alcohols. On the other hand, C60-PEG5000 had a good solubility in methanol and chloroform but a limited solubility in water. Accordingly, the mono-PEGylated fullerenes can be further reacted with biomolecules in alcohol or aqueous solutions, although such application studies are out of the scope of this study. In this study, we describe the C60-PEG5000 in detail.
The structure of the obtained C60-PEG5000 derivative was confirmed by 1H NMR, IR, and MALDI-TOF MS spectra. The 1H NMR revealed new peaks for the PEG chain and triazole ring, although most peaks other than PEG were very broadened and the terminal alkyne peak was not detected (). IR showed H–C≡C and C≡C stretching vibrations of the terminal alkyne at 3243.7 and 2130.0 cm−1, respectively (). MALDI-TOF MS revealed that PEG5000 has a molecular weight distribution with a peak top molecular weight (Mass/Charge) of approximately 5000 (a), whereas the peak top molecular weight of the C60-PEG5000 derivative was slightly shifted toward the higher molecular weight side (b). In addition, the entire peak became broadened in the positive mode (b). This is characteristic of the MALDI-TOF MS spectra of C60 derivatives with n-type semiconductor properties. All these spectroscopic data indicated that the target mono-PEGylated product was indeed obtained.
The antioxidant activity of the C60-PEG5000 derivative was evaluated. It is known that when peroxide radicals generated by the oxidation of linoleic acid attack β-carotene, the absorption intensity of β-carotene at 470 nm decreases.[Citation6] The addition of an antioxidant to the mixture of linoleic acid and β-carotene prevents the attack to β-carotene. Here the change in the absorption intensity at 470 nm was assumed to be directly related to the antioxidant activity. A rapid decrease in the 470 nm absorption intensity started about 10 min after the addition of linoleic acid to the β-carotene solution (see the plots of the control sample in ). However, the decrease was milder when 4.79 mM of the C60-PEG5000 derivative was present (see the plots of 4.79 mM in ). Furthermore, when the concentration of the C60-PEG5000 derivative was increased to 9.96 mM, the decrease in absorbance intensity was further mitigated, suggesting that the PEGylated fullerene derivative has a clear antioxidant effect. The β-carotene-attributed chromaticity at the time of 30 min was 55% for C60-PEG5000 (4.79 m) and 76% for C60-PEG5000 (9.96 m). Although experiments at higher concentrations have not been conducted due to the limited solubility of the developed fullerene derivatives in methanol and water, it is thought that the antioxidant activity of the C60-PEG5000 derivative is similar to those of the previously reported sugar-appended fullerenes.[Citation7]
4. Conclusions
In conclusion, we have introduced hydrophilic PEG substituents without compromising the π-electron system of fullerenes. Although azide groups are known to react with fullerenes, the CuAAC reaction proceeded much faster and the target compound was selectively obtained. It was revealed that the solubilities of the fullerene derivatives greatly changed by the molecular weight of the attached PEG chain. The C60-PEG5000 derivative was soluble in methanol. It was thought that a longer PEG chain was necessary to make it completely water-soluble. However, the antioxidant activity of the C60-PEG5000 derivative could be successfully evaluated. The addition of small amounts (4.79–9.96 mM) of the C60-PEG5000 derivative explicitly inhibited the attack of peroxide radicals to β-carotene, indicating the potent antioxidant properties. In addition, the C60-PEG derivatives synthesized in this study have one more unreacted terminal alkyne. Since the solubility in alcohols is ensured by substituting PEG with a molecular weight of more than 5000, we believe that these fullerene derivatives can be covalently linked to azidated biomolecules by using the second CuAAC reaction. This will create novel bio-nanostructures, such as biomaterials decorated by PEGylated C60 derivatives.
Disclosure statement
No potential conflict of interest was reported by the authors.
Additional information
Funding
References
- (a) Choe, E.; Min, D. B. Mechanisms of Antioxidants in the Oxidation of Foods. Comp. Rev. Food Sci. Food Safe 2009, 8, 345–358. DOI: 10.1111/j.1541-4337.2009.00085.x.; (b) Cataldo, F. Interaction of C60 Fullerene with Lipids. Chem. Phys. Lipids 2010, 163, 524–529.; (c) Cataldo, F.; Rocchi, S.; Ursini, O. Antioxidant Effect of C 60 and C 70 Fullerene in the Autoxidation of Ethyl Oleate. Fuller. Nanotub. Carbon Nanostructures 2013, 21, 624–633.; (d) Sabirov, D. S. H.; Garipova, R. R.; Bulgakov, R. G. What Fullerene is More Reactive toward Peroxyl Radicals? A Comparative DFT Study on ROO • Addition to C 60 and C 70 Fullerenes. Fuller. Nanotub. Carbon Nanostructures 2015, 23, 1051–1057.; (e) Czochara, R.; Kusio, J.; Symonowicz, M.; Litwinienko, G. Fullerene C 60 Derivatives as High-Temperature Inhibitors of Oxidative Degradation of Saturated Hydrocarbons. Ind. Eng. Chem. Res. 2016, 55, 9887–9894.; (f) Galimov, D. I.; Gazeeva, D. R.; Sabirov, D. S.; Bulgakov, R. G. Estimation of the Efficiency of the C 60 and C 70 Fullerenes as Inhibitors of the Radical Chain Oxidation of Сumene. Fuller. Nanotub. Carbon Nanostructures 2023, 31, 176–181.
- Xiao, L.; Takada, H.; Gan, X. H.; Miwa, N. The Water-Soluble Fullerene Derivative “Radical Sponge” Exerts Cytoprotective Action against UVA Irradiation but Not Visible-Light-Catalyzed Cytotoxicity in Human Skin Keratinocytes. Bioorg. Med. Chem. Lett. 2006, 16, 1590–1595. DOI: 10.1016/j.bmcl.2005.12.011.
- Yao, Z.; Tam, K. C. Stimuli-Responsive Water-Soluble Fullerene (C60) Polymeric Systems. Macromol. Rapid Commun. 2011, 32, 1863–1885. DOI: 10.1002/marc.201100426. 21997830
- (a) Nierengarten, J.-F. Copper-Catalyzed Alkyne-Azide Cycloaddition for the Functionalization of Fullerene Building Blocks. Pure Appl. Chem. 2011, 84, 1027–1037. DOI: 10.1351/PAC-CON-11-08-21.; (b) Ho, K.-H. L.; Hijazi, I.; Rivier, L.; Gautier, C.; Jousselme, B.; de Miguel, G.; Romero-Nieto, C.; Guldi, D. M.; Heinrich, B.; Donnio, B.; Campidelli, S. Host–Guest Complexation of [60]Fullerenes and Porphyrins Enabled by “Click Chemistry”. Chem. A Eur. J. 2013, 19, 11374–11381.; (c) Ko, Y.-G.; Hahm, S. G.; Murata, K.; Kim, Y. Y.; Ree, B. J.; Song, S.; Michinobu, T.; Ree, M. New Fullerene-Based Polymers and Their Electrical Memory Characteristics. Macromolecules 2014, 47, 8154–8163.; (d) Constant, C.; Albert, S.; Zivic, N.; Baczko, K.; Fensterbank, H.; Allard, E. Orthogonal Functionalization of a Fullerene Building Block through Copper-Catalyzed Alkyne–Azide and Thiol–Maleimide Click Reactions. Tetrahedron 2014, 70, 3023–3029.; (e) Jevric, M.; Petersen, A. U.; Mansø, M.; Madsen, A. Ø.; Nielsen, M. B. Bismuth(III)‐Promoted Acetylation of ThioEthers into Thioacetates. Eur. J. Org. Chem. 2015, 2015, 4675–4688.; (f) Fensterbank, H.; Baczko, K.; Constant, C.; Idttalbe, N.; Bourdreux, F.; Vallée, A.; Goncalves, A.-M.; Méallet-Renault, R.; Clavier, G.; Wright, K.; Allard, E. Sequential Copper-Catalyzed Alkyne–Azide Cycloaddition and Thiol-Maleimide Addition for the Synthesis of Photo- and/or Electroactive Fullerodendrimers and Cysteine-Functionalized Fullerene Derivatives. J. Org. Chem. 2016, 81, 8222–8233.; (g) Hahn, U.; Nierengarten, J.-F. The Copper–Catalyzed Alkyne-Azide Cycloaddition for the Construction of Fullerene–Porphyrin Conjugates. J. Porphyrins Phthalocyanines 2016, 20, 918–934.; (h) Muñoz, A.; Illescas, B. M.; Luczkowiak, J.; Lasala, F.; Ribeiro-Viana, R.; Rojo, J.; Delgado, R.; Martín, N. Antiviral Activity of Self-Assembled Glycodendro[60]Fullerene Monoadducts. J. Mater. Chem. B 2017, 5, 6566–6571.; (i) Salta, J.; Arp, F. F.; Kühne, C.; Reissig, H.-U. Multivalent 1,2,3‐Triazole‐Linked Carbohydrate Mimetics by Huisgen–Meldal‐Sharpless Cycloadditions of an Azidopyran. Eur. J. Org. Chem. 2020, 2020, 7333–7342.; (j) Rabah, J.; Yonkeu, L.; Wright, K.; Vallée, A.; Méallet-Renault, R.; Ha-Thi, M.-H.; Fatima, A.; Clavier, G.; Fensterbank, H.; Allard, E. Synthesis of a Dual Clickable Fullerene Platform and Construction of a Dissymmetric BODIPY-[60]Fullerene-DistyrylBODIPY Triad. Tetrahedron 2021, 100, 132467.
- Iehl, J.; Nierengarten, J.-F. A Click-Click Approach for the Preparation of Functionalized [5:1]-Hexaadducts of C60. Chemistry 2009, 15, 7306–7309. DOI: 10.1002/chem.200901291.
- (a) Tsuchihashi, H.; Kigoshi, M.; Iwatsuki, M.; Niki, E. Action of Beta-Carotene as an Antioxidant against Lipid Peroxidation. Arch. Biochem. Biophys. 1995, 323, 137–147. DOI: 10.1006/abbi.1995.0019.[PMC]; (b) Farhoosh, R. Antioxidant Activity and Mechanism of Action of Butein in Linoleic Acid. Food Chem. 2005, 93, 633–639.
- Horie, M.; Fukuhara, A.; Saito, Y.; Yoshida, Y.; Sato, H.; Ohi, H.; Obata, M.; Mikata, Y.; Yano, S.; Niki, E. Antioxidant Action of Sugar-Pendant C60 Fullerenes. Bioorg. Med. Chem. Lett. 2009, 19, 5902–5904. DOI: 10.1016/j.bmcl.2009.08.067.
- Kato, S.; Aoshima, H.; Saitoh, Y.; Miwa, H. Highly Hydroxylated or γ-Cyclodextrin-Bicapped Water-Soluble Derivative of Fullerene: The Antioxidant Ability Assessed by Electron Spin Resonance Method and β-Carotene Bleaching Assay. Bioorg. Med. Chem. Lett. 2009, 19, 5293–5296. DOI: 10.1016/j.bmcl.2009.07.149.