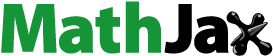
ABSTRACT
The use of coconut leaf stalk fibers in composites has rarely been investigated. This study focuses on the use of Malayan Dwarf coconut leaf stalk fibers as reinforcement in a polyester matrix. After extracting the fibers, the optimum concentration of sodium hydroxide for their mercerization was determined. This was, firstly, done through tensile, contact angle, FTIR, and SEM tests. They were supplemented by preliminary tensile tests which were performed on composites, with 0.5% fiber content (by weight). The selection of the optimum mercerization process led to the production of the composites for further investigations. Two types of plain weave arrangement (single layer and multiple layers) of the fiber reinforcements were considered, with increasing fiber loading. For the mercerization process, 5% sodium hydroxide treatment was found to produce composites with better mechanical properties. The composite, with the single layer arrangement and 1.5% fiber loading, was found to have the highest tensile (46 MPa) and flexural (149 MPa) strength. Two-way ANOVA was used to confirm that the means of the strengths, of the various types of composites tested were not the same. The results of this study are expected to lead to a more sustainable use of waste coconut leaves.
摘要
椰子叶茎纤维在复合材料中的应用很少被研究. 这项研究的重点是使用马来亚矮椰子叶茎纤维作为聚酯基体的增强材料. 提取纤维后,确定了用于纤维丝光的氢氧化钠的最佳浓度. 这首先是通过拉伸、接触角、FTIR和SEM测试来完成的. 对纤维含量为0.5%(按重量计)的复合材料进行的初步拉伸试验对其进行了补充. 最佳丝光工艺的选择导致了复合材料的生产,以供进一步研究. 随着纤维负载的增加,考虑了两种类型的平纹组织(单层和多层)纤维增强物. 对于丝光工艺,发现5%氢氧化钠处理可以产生具有更好机械财产的复合材料. 发现具有单层排列和1.5%纤维负载的复合材料具有最高的拉伸(46MPa)和弯曲(149MPa)强度. 使用双向方差分析来确认测试的各种类型的复合材料的强度平均值不相同. 这项研究的结果有望使废弃椰子叶得到更可持续的利用.
Introduction
The use of coconut coir fiber has been extensively studied as a reinforcement in polymer composites. The diameter and tensile strength (TS) of coir fibers were found to generally vary from 100 μm to 450 μm and from 106 MPa to 270 MPa, respectively (Biagiotti, Puglia, and Kenny Citation2004). However, the tensile strength of the coir fibers is quite low compared to other natural ones such as hemp, jute, flax, or pineapple (Biagiotti, Puglia, and Kenny Citation2004).
Though there have been many studies on the coir fibers, few studies have been performed on fibers from the coconut leaf stalks. Instead, studies have been performed on coconut sheaths and petioles. Maheswari et al. (Citation2012) extracted cellulose microfibrils with diameters in the range of 10–15 μm from the agricultural residue of coconut palm leaf sheath. Zhao et al. (Citation2015) and Xu et al. (Citation2015) have produced cellulose nanofibrils from coconut palm petioles for use as reinforcements in polymer composites. Srinivasababu (Citation2018) produced coconut epoxy composites from coconut sheaths and it was observed to exhibit TS of 50.68 MPa. On the other hand, investigations on the primary flower leaf stalk fiber revealed extremely high TS within the range of 2008–2653 MPa (Thirumurugan et al. Citation2021).
In Mauritius, there are three types of coconut trees, namely, Ceylan (local), Malayan Dwarf, and Pemba. The Malayan Dwarf is commonly grown in hotels and coastal areas for its aesthetic value. Its leaves, cut off during maintenance, lead to a serious waste disposal problem. Hence, this study aims to use the Malayan Dwarf leaf stalk fibers as reinforcement, in a woven configuration, in polymer composites. Polyester was considered as the matrix. A woven arrangement of the fibers was chosen because it generally improves the mechanical performance of composites (Bougueraa, Mouhri, and Ettaqi Citation2018; Kalagi, Patil, and Nayak Citation2016). However, coconut coir fiber composites in woven configuration have rarely been studied because of the shorter length of the coir fibers. The leaf stalk fibers are expected to be thicker and longer than the coir fibers and, therefore, they would be more appropriate for the woven configuration. Positive results, with respect to the mechanical properties of the composites, would lead to a new option for the manufacture of natural fiber composites. It will also result in a sustainable use of the waste coconut leaves in Mauritius with the potential of this material replacing products that are more harmful to the environment.
Methodology
To fulfill the aim of the study, a stepwise method was followed. The optimum sodium hydroxide (NaOH) concentration to be used for the treatment of the coconut fibers was first determined. 2%, 5% and 10% NaOH treated fibers were tested for tensile strength. NaOH has an adverse effect on fiber strength if its concentration is too high. Hence, if the 10% NaOH treatment decreased the fibers’ strength, it would not be considered for further tests.
The variation of the contact angle for the fibers, with the selected types of treatment, was then determined, and FTIR and SEM tests were also performed. For the SEM tests, prior to imaging, all samples were coated with a thin layer of an Au/Pd (60:40) alloy using a Quorum SC7620 sputter coater. The SEM images were then captured using a TESCAN Vega 3 scanning electron microscope operating at a voltage of 20 kV. The working distance (distance between tip of electron gun and sample) was set to 15 mm. Images were then captured.
Composites, with these fibers in a polyester matrix and 0.5% fiber loading (by weight), were used to perform preliminary tests. The results of the tests were used to select the optimum NaOH concentration for the treatment of the fibers. The composites with different fiber loadings were then manufactured and their mechanical properties were determined.
Extraction of the leaf stalks
Green leaf stalks, shown in , were cut from a Malayan Dwarf coconut tree. They were sectioned and the fibers were then removed in layers, as shown in . After extraction, they were soaked in water for 25 days so that they could be easily separated, as shown in . The fibers were then washed thoroughly under running water and then oven-dried at 60°C for 24 hours (Oushabi et al. Citation2017).
Treatment of fibres
After the extraction process, the fibers were treated with 2%, 5%, and 10% NaOH. A total treatment time of 1 hour was allowed for all concentrations of alkali (Oushabi et al. Citation2017). Untreated fibers (UCF) were used as control. The treated fibers (TCF) were then thoroughly washed with distilled water, until its pH remained neutral, and oven-dried at 60°C for 24 hours (Ramasawmy et al. Citation2021). Tensile tests, of all the batches of fibers were carried out. The diameter of the fibers was measured through micrometry (using an eyepiece with an internal reticle scale calibrated with a stage micrometer) at a magnification of x50 prior to the tensile tests. For each fiber the diameter was measured at 5 different points and the average was determined. The linear density of the fibers was also determined by measuring their mass over a total length of 10 m.
Tensile strength of untreated coconut fibres and treated coconut fibres
The tensile tests were carried out as per ASTM C1557–14 (ASTM International Citation2014a), using the Testometric M500-50AT Universal Testing Machine (UTM), as shown in . It was equipped with a 10 kgf load cell and a gauge length of 25.4 mm was used with a crosshead motion of 6.000 mm/min. For each type of treatment of the coconut fibers, 35 samples were randomly tested. The effect of the 10% NaOH treatment was observed. It would not be considered for further tests if a significant decrease in the TS was noted. One-way ANOVA was also carried out to confirm whether the TS means are the same or at least one mean is different from the other(s) at 5% significance level.
Contact Angle (CA) measurements
For the CA measurements, the procedure elaborated by Schellbach, Monteiro, and Drelich (Citation2016) was used. Two coconut fibers of the same type were aligned 1 mm apart and a droplet of water was deposited in between the fibers to create a water bridge, as shown in . After allowing the meniscus to stabilize, the advancing CA was observed. After 5–7 minutes, the liquid column shrank reflecting the receding CA. For both situations, the images were captured and analyzed using the ImageJ software.
Preliminary tests
Preliminary tests were performed with the TCF at 0.5% fiber loading (by weight) to select the NaOH concentration which would lead to the production of composites with the highest tensile strength.
Mold manufacturing process
Based on ASTM D638–14 (ASTM International Citation2014b), test specimens (Type I) of 7 mm thickness were produced. The required amount of fibers was weighed. They were woven within the mold, of size 18 × 18 cm, as shown in . Two plain woven (PW) arrangements of the woven fibers were considered: single layer (side by side) fiber arrangement (PW1) and layered arrangement (PW2). The grid sizes for the single layer fiber arrangement are shown in . Both warp and weft distances were kept constant for the single layer. For layered arrangement, the grid size of 18 × 18 mm was kept constant. shows a 3-layer arrangement of the fibers. It should be noted that the fibers were continuous and they were held at the extremities in the mold.
Table 1. Grid size values/Warp & Weft Distance.
TS of reinforced composite Specimens
The composite specimens were tested based on ASTM D638–14, as shown in . As per the standard, the speed of 1.500 mm/min was chosen so that the rupture occurs within 0.5 to 5 minutes. 5 samples were used for each type of composite.
Insert and (b) here.
Flexural strength of reinforced composites
For the flexural strength (FS), the ASTM D790–17 (ASTM International Citation2019) standard was considered. A specimen size of 120 mm x 13 mm x 5 mm was selected with a support span of 80 mm and a crosshead motion of 2.133 mm/min was chosen (Khan et al. Citation2021). A load cell of 5000 kgf was used. Five samples were selected for each type of composite.
For the analysis of the results, two-way ANOVA was performed to determine whether the difference in the means of the TS was due to the woven arrangements and/or the fiber loadings. The interaction between these two properties was also determined. A significance level of 5% was considered. The same procedure was adopted for the FS.
Results and discussions
TS and average diameter of fibers
shows the average diameter of the UCF and TCF. The 5% NaOH treated fibers resulted in the minimum diameter. The linear density was 131.3 g/km or Tex.
Table 2. Summary of fiber diameter and contact angle results.
The average diameter of the treated fibers was slightly higher than those of coir fibers (Mishra and Basu Citation2020; Sengupta and Basu Citation2016). It was, however, within the range of diameters generally obtained for coconut fibers which is between 100 and 795 μm (Sengupta and Basu Citation2016). As for the linear density, it was much higher than that of other natural fibers, such as sisal, jute, or hemp (Balakrishnan, Dharmasri Wickramasinghe, and Samudrika Wijayapala Citation2019; Mbise, Masombe, and Venance Citation2022). Hence, it can be deduced that the coconut leaf stalk fibers have higher flexural rigidity.
Tensile strength
The tensile strength of the fibers treated with various percentages of NaOH is shown in . The UCF yielded an average TS of 56.7 MPa which was very low when compared to the TCF. The 2% NaOH TCF yielded the highest TS (209.2 MPa) followed by the 5% NaOH. 10% NaOH TCF had the lowest TS due to the fact that the NaOH damaged the fibers. A similar trend was observed for the Young’s Modulus with mean values of 0.47 GPa, 2.42 GPa, 2.29 GPa, and 1.80 GPa for the UCF, 2% NaOH TCF, 5% NaOH TCF and 10% NaOH TCF respectively. Hence, the 10% NaOH was not considered for further tests. From the one-way ANOVA test, the F-value was found to be 20.0 while the critical F-value was 2.7. This supported the claim that at least one of the means is different. It should be noted that the percentage elongation at break was 11.3, 8,9, 6.8 and 7.4 for the UCF, 2% NaOH TCF, 5% NaOH TCF and 10% NaOH TCF respectively. It should be noted that eco-friendly wood ash solution may be used instead of NaOH, which is synthetic in nature.
With respect to the tensile strength of the fibers, 209.5 MPa obtained in this study is higher than that reported by Dharmaratne et al. (Citation2021), while similar results were observed by Verma and Gope (Citation2015). The stalk fibers was observed to have higher tensile strength than coir fibers. This can be due to several factors such as fiber diameter fiber species and origin, method of extraction of the fibers, porosity and pore size distribution (Madueke, Mbah, and Umunakwe Citation2022). On the other hand, they show lower Young’s Modulus as compared to those reported by Mishra and Basu (Citation2020) and Dharmaratne et al. (Citation2021) for coir fibers. Hence, the stalk fibers can also be considered less stiff than the coir fibers.
FTIR & SEM
shows the FTIR Spectra of the UCF and 2% and 5% NaOH TCF. It can be observed that the 2% TCF and UCF displayed similar peaks while they decreased significantly for the 5% NaOH TCF.
Referring to , the absorption band at 3329.96 and 3288.41
observed for the UCF and TCF were characterized by the vibration of the O-H stretching (Oushabi et al. Citation2017; Xu et al. Citation2015; Zhao et al. Citation2015). This is due to cellulose and hemicellulose in the fiber. The intensity of the peak decreased with increasing NaOH concentration. The 5% NaOH mercerization leads to a net decrease in the intensity of the peaks. This can be explained by the fact that during the mercerization process, Na+ ions widen the smallest pores in between the lattice planes and penetrate into them together with water molecules, causing swelling (Ouarhim, Zari, and Qaiss Citation2019). Subsequent rinsing with water removes the linked Na-ions and shrinks the fibers back to yield a new allomorph, cellulose-II, which is thermodynamically more stable than cellulose-I. The holistic effect is observed in with a significant decrease in the diameter of the fibers upon mercerization. The amplitude of the peaks at 2920.12
and 2852.44
were allocated to C-H bond stretching of cellulose and hemicellulose (Thirumurugan et al. 2019) which have been observed to decrease for both 2% and 5% TCF with the latter being the most significant compared to the UCF. This is in accordance with the fact that mercerization not only has an impact on the cellulose present in the fiber but also removes noncellulosic components (hemicellulose, lignin, and pectin). The peaks at 1593.88, and 1596.74 cm−1 can be attributed to in-plane C=C aromatic vibration, associated with lignin (Li et al. Citation2018). This explains the disappearance of the peaks with the NaOH treated fibers. The intensity of the peak at 1027.86
was allocated to C-O bond stretching of cellulose, lignin, and hemicellulose which was observed to decrease for the TCF.
The FTIR results show that the mercerization process was successfully performed. Moreover, the cellulose content (which refers to the peaks at 2920.12, 2852.44
and 1027.86 cm−1) decreased more significantly for the 5% NaOH TCF compared to the 2% NaOH TCF, which most probably led to the decrease in TS for the 5% NaOH TCF.
To supplement the FTIR results, the surface morphology of the TCF was observed through SEM, as shown in . Referring to , it was found that the mercerization treatment resulted in a rough fiber with a surface free from impurities. shows that impurities, such as pectin, hemicelluloses, hemicellulose, lignin, wax and/or oil, that cover the fiber surface were removed. The elliptical pits were exposed, leading to an increased surface roughness. Damage in the fiber due to high concentration or excessive use of NaOH is absent, suggesting that the use of NaOH has been optimally made.
Hydrogen bond intensity (HBI) was used to interpret qualitative changes in crystallinity in cellulose. For untreated fiber, it was equal to 2.64. For 2% and 5% NaOH TCF it was 3.07 and 3.98, respectively. As HBI increases crystallinity decreases. Hence, the increase in HBI represents an increase in the conversion of cellulose I to cellulose II, which confirms the observation and analysis of the absorption band at 3329.96 and 3288.41
.
CA test
The results obtained from the CA tests have been summarized in . The highest receding CA obtained was 53 ± 7 for the 5% TCF and the lowest was 37 ± 1
for the 2% TCF. Based on Schellbach, Monteiro, and Drelich (Citation2016), advancing contact angles of 40° to 50° were considered as weakly hydrophilic. The 5% NaOH TCF is expected to be the least hydrophilic of the other treated fibers. The 5% NaOH is also expected to result in the roughest fiber surface since it leads to the highest differences in the CA.
Preliminary tests
Preliminary tests were performed to determine which one of the 2% and 5% NaOH would be considered for further tests. Hence, both the 2% and 5% NaOH TCF were used to manufacture a composite plate of 0.5% fiber loading (by weight). The average TS for the UCF was 18.6 MPa. For the 2% NaOH TCF, it was 14.3 MPa and for the 5% NaOH TCF it was 24.6 MPa. Hence, for further tests, the fibers were treated with 5% NaOH.
Though there was a decrease in crystallinity in the fiber cellulose with the 5% NaOH treatment, the overall TS of the composite increased due to a greater loss in lignin and hemicellulose and the fiber was less hydrophilic. Moreover, the 5% TCF was expected to have better adhesion with the matrix since the presence of polar O-H groups was reduced (reduced peak between 3329–3288 cm−1) which made the fibers exhibit a non – polar behavior and, hence, led to a better adherence with the nonpolar resin. Thirdly, from the CA results, it was found that the 5% NaOH TCF consisted of a rougher surface which could have increased the adherence of the fiber in the matrix.
Further tests - mechanical properties
Tensile strength
The TS results for the composites are summarized in . There was no difference in the values of the TS and Young’s Modulus for the 0.5% fiber content for PW1 and PW2. The TS significantly decreased for PW2 for the 1.5% fiber loading. It can be observed that for both PW, the TS increased with increasing fiber loading except for the 1.0% fiber loading (Bakri, Jayamani, and Hamdan Citation2017). For the PW1 (side by side fiber arrangement), the maximum TS obtained was 44.6 MPa which showed an increase of 140% when compared to the plain polyester resin while for the PW2 (layered arrangement), the maximum TS obtained was 26.6 MPa showing a total increase of 44% only.
Table 3. Summary of tensile strength results.
Moreover, the strain of the PW1 samples increased slightly from 3 ± 1 to 3.25 ± 0.02 with the increase in fiber loading while for the PW2 samples, the strain decreased from 3 ± 1 to 1.9 ± 0.4 and then increased to 2.2 ± 0.6. Compared to the strain of the plain resin (3 ± 1), PW1 sample had similar elongations while the PW2 samples showed signs of brittleness. In both cases, the Young’s Modulus increased (by 94% for PW1 and by 28% for PW2) implying that the PW1 samples were stiffer than the PW2 samples.
From the Two-Way ANOVA test, the P-values that were obtained for the Plain Weave Arrangement, the Fibre Loading and the Interaction were 0.017, 0.00010 and 0.043 respectively. Hence, there is enough evidence to support the claim that there is a difference in means of the TS using different plain weave arrangements.
Flexural strength
The results for FS are summarized in . It can be observed that the 0.5% wt. fiber content did not reinforce the resin. This reduction in strength was due to insufficient fiber loading. Nevertheless, for both PW, the FS was seen to increase with higher fiber loading. For PW1, the maximum FS obtained was 148.7 MPa which showed a 100% increase when compared to the plain resin. Likewise, PW2 had a maximum FS of 89.3 MPa (20% increase only). Based on this percentage increase, it can easily be deduced that fiber loading was a dominant factor. The maximum force at break for the PW1 samples was 360 N and that for PW2 was 194 N, which are in agreement with the results obtained by Devaraju, Babu, and Gnanavelbabu (Citation2018).
Table 4. Summary of flexural strength results.
Moreover, for both PW, an overall decrease in strain was observed with an increase in fiber loading. The strain decreased from 4.70.3 to 2
1 and then peaked to 2.7
0.7 for PW1 samples while for the PW2, the strain decreased from 4.7
0.3 to 4
1. This showed that the PW1 samples had lower elongations (signs of brittleness) than the PW2 samples.
In terms of flexural modulus, an increase of 236% was observed for the PW1 samples while the PW2 samples showed only a 69% increase. This showed that the PW1 samples were stiffer than the PW2 samples.
From the Two-Way ANOVA test, the P-values that were obtained for the Plain Weave Arrangement, the Fibre Loading and the Interaction were 0.013, 1.32 × 10−8 and 0.0017 respectively. Hence, there is enough evidence to support the claim that there is a difference in means of the TS using different plain weave arrangements.
Comparing results obtained to those of other studies
The TS of the 2% NaOH TCF was the highest (209 MPa) in this study. It is also higher than those of the pandanus species (Rafidison et al. Citation2020), palm trees (Ramasawmy et al. Citation2021), and coir (Bui et al. Citation2020). It is, however, in the same range as those of the bagasse fibers and is much lower than those of sisal, kenaf, and hemp (Kumar et al. Citation2019). The cellulose content of the fibers is one of the main reasons for this behavior.
As for the TS of the composites, the results vary significantly. Generally, it has been observed that with a fiber content of above 10%, the tensile strength has a tendency to decrease (Júnior et al. Citation2010; Priya, Raju, and Naveen Citation2014). The highest TS of the composites obtained in this study was 45 MPa. It is very comparable to results obtained with composites manufactured using the hand lay-up technique, with other natural fibers and even with higher fiber content. Khan et al. (Citation2021), for example, studied jute and kenaf fiber composites and obtained similar results for the TS. The hand lay-up technique was used, with the fibers in a woven arrangement. The composite consisted of an epoxy matrix with a fiber loading of 30%. Hybrid composites of the two fibers were found to have a TS of 39 MPa to 43 MPa and the FS was in the range of 56 MPa to 76 MPa. Composites of polyester-coir fibers, on the other hand, show much lower TS ranging from 20 MPa to 31 MPa (Júnior et al. Citation2010; Priya, Raju, and Naveen Citation2014). Low mechanical properties are commonly obtained because of low adhesion and moisture resistance properties of the fibers and the porosity of the matrix. Silane treatments can produce fibers that are almost hydrophobic thus improving their adherence, and leading to better mechanical properties of the composites (Chand and Mohammed Citation2021). Composites manufactured from fibers, such as sisal, bamboo and date palm, especially through the use of heat and pressure, would exhibit much higher TS and FS (Biagiotti, Puglia, and Kenny Citation2004).
Conclusion
In this study, coconut leave stalk fibers were considered as reinforcement in a polyester matrix. The highest value of the average tensile strength of the fibers was found to be 209.5 MPa and the highest value for the average Young’s Modulus was 2.42 GPa. The optimum NaOH concentration for the treatment of the fibers which led to the highest TS and FS in the composites was 5% NaOH. Single layers and layered arrangements of the woven fibers as reinforcement were tested. The single layer arrangement was observed to lead to higher TS (45 MPa) and FS (149 MPa). The TS and FS obtained from the composites were also found to be much higher than those obtained with coir fibers (Bui et al. Citation2020).
The stalk fiber composites may be used to replace non-load bearing concrete products such as slabs, road and garden edges, and decorative bricks. Further studies can be performed on the use of the coconut leaf stalk fibers together with shorter coconut husk fibers or other stronger fibers to increase the fiber loading so as to improve the mechanical properties of the composites.
Highlights
The mechanical properties of coconut leaf stalk fibers were investigated.
The fibers were used as reinforcement in the manufacturing composites, with polyester as the matrix.
The fibers were arranged in a woven configuration.
Mercerization with 5% NaOH was found to lead to better mechanical properties of the coconut fiber-polyester composite.
5% NaOH treated coconut fiber in a single layer arrangement with 1.5% fiber loading (by weight) led to the highest tensile strength and flexural strength.
Disclosure statement
No potential conflict of interest was reported by the author(s).
References
- ASTM International. 2014a. ASTM C1557-14- standard test method for tensile strength and young’s modulus of fibers. PA, USA: ASTM.
- ASTM International. 2014b. ASTM D638-14- standard test method for tensile properties of plastics. PA, USA: ASTM.
- ASTM International. 2019. ASTM D790-17- standard test methods for flexural properties of unreinforced and reinforced plastics and electrical insulating materials. PA, USA: ASTM.
- Bakri, M., E. Jayamani, and S. Hamdan. 2017. Processing and characterization of banana fiber/epoxy composites: effect of Alkaline treatment. Materials Today: Proceedings 4 (2):2871–12. doi:10.1016/j.matpr.2017.02.167.
- Balakrishnan, S., G. L. Dharmasri Wickramasinghe, and U. G. Samudrika Wijayapala. 2019. Study on dyeing behavior of banana fiber with reactive dyes. Journal of Engineered Fibers and Fabrics 14 (1):1–12. doi:10.1177/1558925019884478.
- Biagiotti, J., D. Puglia, and J. M. Kenny. 2004. A review on natural fibre-based composites-part I. Journal of Natural Fibers 1 (2):37–68. doi:10.1300/J395v01n02_04.
- Bougueraa, F., S. Mouhri, and S. Ettaqi. 2018. Experimental analysis of biocomposite Raphia fiber/Chitosan influence of weaving process on mechanical properties. Procedia Manufacturing 22:180–85. doi:10.1016/j.promfg.2018.03.028.
- Bui, H., N. Sebaibi, M. Boutouil, and D. Levacher. 2020. Determination and review of physical and mechanical properties of raw and treated coconut fibers for their recycling in construction materials. Fibers 8 (6):37. doi:10.3390/fib8060037.
- Chand, N., and F. Mohammed. 2021. Sisal-reinforced polymer composites. In Woodhead publishing series in composites science and engineering, tribology of natural fiber polymer composites, N. Chand and F. Mohammed. 84-107: Woodhead Publishing. 10.1533/9781845695057.84
- Devaraju, A., K. Babu, and A. Gnanavelbabu. 2018. Investigation on the mechanical properties of coconut bunch fiber reinforced Epoxy with Al2O3 Nano particles composites for structural application. Materials Today: Proceedings 5 (6):14252–57. doi:10.1016/j.matpr.2018.03.006.
- Dharmaratne, P. D., H. Galabada, R. Jayasinghe, R. Nilmini, and R. U. Halwatura. 2021. Characterization of physical, chemical and mechanical properties of Sri Lankan Coir fibers. Journal of Ecological Engineering 22 (6):55–65. doi:10.12911/22998993/137364.
- Júnior, H. S., F. Lopes, L. Costa, and S. Monteiro. 2010. Mechanical properties of tensile tested coir fiber reinforced polyester composites. Review Material 15:113–18.
- Kalagi, G. R., D. R. Patil, and M. N. Nayak. 2016. Natural fibre reinforced polymer composite materials for wind turbine blade applications. International Journal of Scientific Development and Research 1 (9):28–37.
- Khan, T., M. T. H. Sultan, A. U. M. Shah, A. H. Ariffin, and M. Jawaid. 2021. The effects of stacking sequence on the tensile and flexural Properties of Kenaf/Jute Fibre Hybrid composites. Journal of Natural Fibers 18 (3):452–63. doi:10.1080/15440478.2019.1629148.
- Kumar, R., M. I. Ul Haq, A. Raina, and A. Anand. 2019. Industrial applications of natural fibre-reinforced polymer composites – challenges and opportunities. International Journal of Sustainable Engineering 12 (3):212–20. doi:10.1080/19397038.2018.1538267.
- Li, X., Y. Wei, J. Xu, N. Xu, and Y. He. 2018. Quantitative visualization of lignocellulose components in transverse sections of moso bamboo based on FTIR macro‑ and micro‑spectroscopy coupled with chemometrics. Biotechnology for Biofuels 11 (1):263. doi:10.1186/s13068-018-1251-4.
- Madueke, C. I., O. M. Mbah, and R. Umunakwe. 2022. A review on the limitations of natural fibres and natural fibre composites with emphasis on tensile strength using coir as a case study. Polymer Bulletin 80 (4):3489–506. doi:10.1007/s00289-022-04241-y.
- Maheswari, C. U., K. O. Reddy, E. Muzenda, B. R. Guduri, and A. V. Rajulu. 2012. Extraction and characterization of cellulose microfibrils from agricultural residue – Cocos nucifera L. Biomass & bioenergy 46:555–63. doi:10.1016/j.biombioe.2012.06.039.
- Mbise, E., M. Masombe, and T. Venance. 2022. Study of physical and mechanical properties of Sansevieria Ehrenbergii Fibres (SEF). Journal of Textile Engineering Fashion Technology 8 (2):38‒46. doi:10.15406/jteft.2022.08.00299.
- Mishra, L., and G. Basu. 2020. Coconut fibre: Its structure, properties and applications. In Handbook oF natural fibres volume 1: types, properties and factors affecting breeding and cultivation, ed. R. M. Kozłowski and M. Mackiewicz-Talarczyk, 2nd ed., 231–55. Cambridge, UK: Woodhead Publishing Series in Textiles.
- Ouarhim, W., N. Zari, and A. Qaiss. 2019. Mechanical performance of natural fibers–based thermosetting composites. In Woodhead publishing series in composites science and engineering, mechanical and physical testing of biocomposites, fibre-reinforced composites and Hybrid composites, ed. M. Jawaid, M. Thariq, and N. Saba, 43–60. Cambridge, UK: Woodhead Publishing.
- Oushabi, A., S. Sair, F. Oudrhiri Hassani, Y. Abboud, O. Tanane, and A. El Bouari. 2017. The effect of alkali treatment on mechanical, morphological and thermal properties of date palm fibers (DPFs): Study of the interface of DPF–Polyurethane composite. South African Journal of Chemical Engineering 23:116–23. doi:10.1016/j.sajce.2017.04.005.
- Priya, N. A. S., P. V. Raju, and P. Naveen. 2014. Experimental testing of polymer reinforced with coconut coir fiber composites. International Journal Emerging Technology Advence Engineering 4 (12):453–60.
- Rafidison, B. H., H. Ramasawmy, J. Chummun, and F. B. V. Florens. 2020. Using infrared spectrum analyses to predict tensile strength of fibres in a group of closely related plant species: Case of Mascarenes Pandanus spp. SN Applied Sciences 2 (11):1922. doi:10.1007/s42452-020-03667-1.
- Ramasawmy, H., J. Chummun, F. B. V. Florens, and H. Joyram. 2021. Palm leaves as potential natural-fibre source for a Tropical Island developing state. Journal of Natural Fibers 18 (4):607–19. doi:10.1080/15440478.2019.1642823.
- Schellbach, S. L., S. N. Monteiro, and J. W. Drelich. 2016. A novel method for contact angle measurements on natural fibers. Materials Letters 164:599–604. doi:10.1016/j.matlet.2015.11.039.
- Sengupta, S., and G. Basu. 2016. Properties of coconut fiber. In Encyclopedia of Renewable and Sustainable Materials, S. Hashmi and I. A. Choudhury, 263–81. Amsterdam: Elsevier.
- Srinivasababu, N. 2018. Fabrication of Chemically Treated Pure-Bred Coconut Leaf Sheath Fibre Reinforced Composites and Determination of Mechanical Properties. Macromolecular Symposia 382 (1):1800119. doi:10.1002/masy.201800119.
- Thirumurugan, R., M. Jayaraj, D. Shanmugam, and T. Ramkumar. 2021. Characterization of new natural cellulosic fiber from Coconut Tree Primary Flower Leaf Stalk Fiber(CPFLSF). Journal of Natural Fibers 18 (11):1844–56. doi:10.1080/15440478.2019.1701608.
- Verma, D., and P. Gope. 2015. The use of coir/coconut fibers in composites. In Biofiber reinforcements in composite materials, O. Faruk and M. Sain. 285-319: Woodhead Publishing. 10.1533/9781782421276.3.285
- Xu, C., S. Zhu, C. Xing, D. Li, N. Zhu, H. Zhou, and F. Zhao. 2015. Isolation and properties of cellulose Nanofibrils from coconut palm petioles by different mechanical process. Plos One 10 (4):e0122123. doi:10.1371/journal.pone.0122123.
- Zhao, Y., C. Xu, C. Xing, X. Shi, L. M. Matuana, H. Zhou, and X. Ma. 2015. Fabrication and characteristics of cellulose nanofibril films from coconut palm petiole prepared by different mechanical processing. Industrial Crops and Products 65:96–101. doi:10.1016/j.indcrop.2014.11.057.