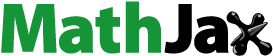
ABSTRACT
The inferior mechanical and water absorption properties of natural fibers are massive challenges to utilizing them for industrial applications. The study aims to enhance tensile strength, impact strength, water absorption, and degradation of sisal/polyester composites using glass and carbon fillers. Four fillers proportions (2.5 wt.%, 5 wt.%, 7.5 wt.%, 10 wt.%) and two sisal fiber proportions (20 wt.% and 30 wt.%) were taken to fabricate the composites. Experimentations were performed according to the ASTM standards. The fillers improved tensile and impact strength, water absorption, and degradation of the composites. Carbon-filled composites displayed better results than the corresponding glass-filled composites. Carbon fillers showed a maximum increment of 24.2% in tensile and 78.5% in impact strength for the dry 20/80 composites, and 14.7% in tensile and 57.3% in impact strength for the dry 30/70 composites. Moreover, carbon fillers reduced water absorption by 55.4% for the 20/80 composites and by 53.6% for the 30/70 composites. The highest values of tensile and impact strengths were obtained for the 5 wt.% carbon-filled 30/70 composite; whereas, the lowest water absorption was for the 10 wt.% carbon-filled 20/80 composite. The lowest degradation of 2% in tensile and impact strengths was exhibited for the 7.5 wt.% carbon-filled 20/80 composites.
摘要
天然纤维的低劣机械性能和吸水财产对其工业应用带来了巨大挑战. 该研究旨在提高使用玻璃和碳填料的剑麻/聚酯复合材料的拉伸强度、冲击强度、吸水性和降解性. 采用四种填料比例(2.5重量%、5重量%、7.5重量%、10重量%)和两种剑麻纤维比例(20重量%、30重量%)来制备复合材料. 根据ASTM标准进行实验. 填料提高了复合材料的拉伸和冲击强度、吸水性和降解性. 碳填充复合材料比相应的玻璃填充复合材料显示出更好的结果. 对于干燥的20/80复合材料,碳填料的拉伸强度和冲击强度分别增加了24.2%和78.5%,对于干燥的30/70复合材料,拉伸强度和抗冲击强度分别提高了14.7%和57.3%. 此外,对于20/80复合材料,碳填料降低了55.4%的吸水率,对于30/70复合材料,降低了53.6%的吸水率. 对于5 wt.%碳填充的30/70复合材料,获得了最高的拉伸强度和冲击强度值; 而对于10 wt.%碳填充的20/80复合材料,吸水率最低. 对于7.5 wt.%的碳填充20/80复合材料,在拉伸和冲击强度方面表现出2%的最低降解.
Introduction
Currently, natural fibers are gaining the attention of researchers and are already being used as reinforcements in some applications. The biodegradability, renewability, low densities, reduced energy consumption, less health risk, and low processing costs make natural fibers preferable reinforcements over synthetic fibers in the composite industry (Gupta and Deep Citation2019; Tesfay, Kahsay, and Senthil Kumar Citation2020). However, the application of these fibers remained limited to parts where the mechanical performance is not very demanding. Sisal fibers are among the natural fibers being traditionally used as bags, baskets, ropes, and rugs but they are barely used as reinforcements in the composite industry. That is due to their low strength, incompatibility with the polymer matrix, high water absorption properties, and degradation due to water absorption. Researches have been performed to solve the problems through treatments, hybridizations, and alternations on the stacking sequences and orientations of the hybrid fibers (Fiore, Bella, and Valenza Citation2015; Jarukumjorn and Suppakarn Citation2009; Melkamu, Kahsay, and Tesfay Citation2019; Premnath Citation2019; Tesfay, Kahsay, and Senthil Kumar Citation2020, Citation2022; Yahaya et al. Citation2015).
The objective of the research is to widen the application of the unique sisal plants grown in the northern part of Ethiopia in abundant amounts. The unique growth and development of the plants are due to the suitable climate and the chemical and physical properties of the soil (Asfaw Citation2011). The plants are drought resistant and easy to cultivate which could serve as a source of income for the peasants, who are suffering from a shortage of rain in the arid region of the northern part of Ethiopia, by increasing the demand for the plant via continuous research. The study is aiming to use the sisal fibers for interior and exterior parts of automotive, water tankers, and structural applications where the weight, tensile strength, impact strength, water absorption, and degradation due to water absorption properties are vital for their better performance. However, the inferior tensile strength, impact strength, water absorption, and degradation properties of the sisal fiber reinforced composites have been great challenges. Tesfay, Kahsay, and Senthil Kumar (Citation2020, Citation2022) studied the effects of unidirectional glass and carbon fibers on the tensile strength, impact strength, water absorption, and degradation properties of the sisal/polyester composites and significant improvements were reported. Nevertheless, further research to minimize the cost of the composite material without compromising on the performance is required. Fillers are among the effective hybrid materials to enhance the properties of fiber-reinforced composites and reduce the cost of their final product (Das et al. Citation2020; Tesfay, Kahsay, and Senthil Kumar Citation2020; Xanthos Citation2010). Many researchers have tried to enhance the performance of fiber-reinforced polymeric composite materials using various types of natural and synthetic fillers such as wood dust flour, wood charcoal, carbon nanotube fillers, graphene powders, etc. (Dinesh et al. Citation2020; Ganapathy et al. Citation2021; Saiteja, Jayakumar, and Bharathiraja Citation2019). Over the past years, the biodegradability, cheaper cost, sustainability, and environmental friendliness of natural fibers created interest among researchers in developing composites with the addition of nano-fillers on the natural fibers as a substitute for synthetic fibers (Borba, Tedesco, and Lenz Citation2014; Chaudhary, Rajput, and Bajpai Citation2017; Devnani and Sinha Citation2019; Ganapathy et al. Citation2021; Mohan and Rajmohan Citation2018; Nayak, Nayak, and Panigrahi Citation2021; Reddy et al. Citation2019; Saiteja, Jayakumar, and Bharathiraja Citation2019; Sarker et al. Citation2018). Ganapathy et al. (Citation2021) studied the effect of graphene powder on banyan aerial root fibers reinforced epoxy composites. A maximum increment of 45.4% in tensile and 5% in flexural strength were attained for a 4 wt.% of graphene powder-filled composite; however, further increase in the graphene content decreased the mechanical properties. Saiteja, Jayakumar, and Bharathiraja (Citation2019) reported the highest tensile and impact strengths in a jute-reinforced hybrid polymer composite filled with a 6% volume of carbon nanotube fillers. However, the flexural strength increased up to the addition of 8% volume of nanotube fillers and then decreased due to the agglomeration of nanotube fillers in the composite. Further analysis of various research findings related to the present study is given in . In this study, glass and carbon fillers are the hybrid materials used in the sisal/polyester hybrid composites mainly because of their high strength and low water absorption properties. Moreover, polyester is used as a matrix for its low cost and availability (Chandgude and Salunkhe Citation2021). Researchers reported good embedment between the polyester matrix and the reinforcements used in this research: sisal, glass, and carbon (Altay Citation2019; Biswas et al. Citation2019; Gupta and Deep Citation2019). The novelty of this research is the improvement of tensile strength, impact strength, water absorption, and degradation properties of local sisal fiber/polyester composites to the level of requirements of the proposed applications through the addition of the appropriate amount of carbon and glass fillers. A comparative study on the effect of carbon and glass fillers on the tensile strength, impact strength, water absorption, and degradation properties of the composites is also a new work. The effect of the content of fillers, which remained not widely explored before, is also studied.
Table 1. Effect of fillers on the mechanical and water absorption properties of hybrid composites.
Materials and methods
Materials
Glass and carbon fillers of size 0.1 mm were purchased from Faserverbundwerkstoffe composite technology, Germany. Unsaturated polyester resin and butanox hardener were procured from a local composite material manufacturer. Sisal fibers were collected from the outskirts of Mekelle, Tigray, Ethiopia. Commercially available sodium hydroxide was used to treat the sisal fibers.
Fiber treatment and ply preparation
Sisal fibers were manually extracted from locally collected sisal leaves. As recommended by Yahaya et al. (Citation2015), the sisal fibers were treated with a 6 wt.% diluted solution of sodium hydroxide to enhance their interfacial bonding properties. The treated fibers were cut in a length of 270 mm out of which unidirectional plies, size 270 150 mm2, were made by stitching them together using a sewing machine. Some of the unidirectional sisal plies are given in .
Matrix preparation
The matrix was prepared by mixing the polyester resin with the butanox hardener as per the manufacturer’s recommended mix ratio. Moreover, a modified matrix was prepared by adding glass and carbon fillers at 2.5%, 5%, 7.5%, and 10% by weight. The carbon and glass fillers were made below 10 wt.% as maximum results are reported at filler loading less than 10% (Ganapathy et al. Citation2021; Saiteja, Jayakumar, and Bharathiraja Citation2019). A measured amount of fillers and polyester were mixed in a container and a mechanical stirrer for 10 minutes at a speed of 300 rpm was used to ensure uniform distribution of the fillers in the modified matrix. After that, the desired amount of hardener was added to the matrix and mixed for 2 minutes again.
Composite preparation
The composites were prepared using the hand layup technique followed by compression molding. A releasing agent was used for easy removal of the composites from the mold after curing. The amounts of sisal fibers used to fabricate the composites were 20 wt.% and 30 wt.% due to Jenish et al. (Citation2022) reported maximum results at the optimum level of 30% hybrid fibers and 3% nano-silica. Moreover, Reddy et al. (Citation2020) added graphite fillers to 20% fiber-reinforced composites and recorded a 28% increase in tensile strength and 146% in impact strength for 15 wt.% granite filler content when compared with the 0 wt.% granite filler. Thus, the carbon and glass fillers are added in both 20 and 30 wt.% fiber-loaded composites to compare the results and utilize the best ones. The polyester resin alone and a modified polyester resin with fillers were used as a matrix. Two laminates (T1 and T2) were fabricated using the matrix without fillers and the sisal plies. The remaining sixteen (T3 to T18) composites were prepared using the modified matrix and the sisal plies. The unidirectional sisal plies were placed in a mold and impregnated with the corresponding matrix by pouring them onto the mat. A roller was used to squeeze the impregnated laminates for a uniform distribution of the matrix throughout the sisal ply and to release any trapped air bubbles. The composites were kept in compression at a constant load of 2 kN for 24 hours for curing. The size of the composites was 270 × 150 mm2. The nomenclature and content of the sisal fibers, glass fillers, carbon fillers, and polyester resin in the composites are given in . Sisal fibers and plies are presented in .
Table 2. The nomenclature and content of the sisal fibers, glass fillers, carbon fillers, and polyester resin in the composite.
Testing methods
Water absorption
The water absorption specimens were prepared according to ASTM D 570–98 (Citation1998). The dimension of the specimens was 76.2 25.4
4 mm3. Pre-weighed specimens were submerged in water at room temperature. At every 24 hours intervals, the samples were taken out of the water and weighed immediately after any water at the surface of the samples is removed using clean cotton. At the pre-determined time intervals, the specimens were weighed and placed into the water again until saturation had taken place after 15 days of immersion time. A digital balance with a precision of 0.01 g was used to measure the weight. The water absorption behavior of the specimens is evaluated using EquationEquation 1
(1)
(1) .
Where -is the percentage of water absorption of the specimen,
-is the weight of the wet specimen, and
-is the weight of the dry specimen.
Tensile test
Tensile test specimens were prepared according to ASTM D3039/D3039M (Citation2014). For every test, five samples, size 250 × 15 × 3 mm3, were prepared by cutting from each composite. The cut ends were polished using file and emery paper to avoid any rough and cracked edges. To study the effect of water absorption and hybridization on the degradation of tensile strength of the composites, wet samples were prepared by soaking specimens in a water bath for 15 days. A universal tensile testing machine (Microcomputer Controlled Electro-hydraulic Servo Universal Testing Machine, Model: SI-1000KN) was used to perform the tensile test at a crosshead speed of 2 mm/minute. The experimentations were performed under ambient conditions.
Impact test
The charpy impact test was performed according to ISO 179–1 (Citation2010). For every test, at least five un-notched samples with a size of 80 10
4 mm3 were prepared. To study the effect of water absorption and hybridization on the degradation of impact strength of the composites, wet samples were prepared by soaking specimens in a water bath for 15 days. The impact test was conducted on a charpy impact tester (Pendulum Impact Testing Machine, Model: SI-42, Impact Energy 150 J). The samples were hammered in their flatwise and normal direction. The experimentations were performed under ambient conditions.
Results and discussions
Water absorption
The percentages of water absorption of all composites are shown in , and . The maximum water absorption is observed for the pure sisal/polyester 20/80 and 30/70 composites (T1 and T2). That is because of the hydrophilic nature of sisal fibers. The percentage of water absorption showed an increment with the rise of sisal fibers amount in the composites.
Figure 2. Effect of glass fillers on the water absorption rate: (a) 20/80 composites and (b) 30/70 composites.

Figure 3. Effect of carbon fillers on the water absorption rate: (a) 20/80 composites and (b) 30/70 composites.

Table 3. Percentage of water absorption at saturation of the composites.
Effect of glass filler
As can be observed in , the addition of glass fillers significantly reduced the percentage of water absorption of both the 20/80 and 30/70 sisal/polyester composites. That is due to the hydrophobic behavior of glass decrease the affinity of sisal/polyester composites for water (Tesfay, Kahsay, and Senthil Kumar Citation2022). Moreover, the glass fillers may fill the voids and reduce their content in the composites, thereby hindering the penetration of water particles into the composites. The hybrid 20/80 sisal/polyester composites showed lower water absorption than the hybrid 30/70 sisal/polyester composites filled with the same amount of glass fillers. The lowest water absorption among the glass-filled composites is recorded in the 10 G 20/80 composite (3.4%), which represents a decrease of 47.7% when compared to the laminates T1. Laminates T10 (10 G 30/70 composite) and laminates T5 (7.5 G 20/80 composite) showed the second-lowest water absorption among the glass-filled composites (3.9%). The lowest water absorption among the glass-filled 20/80 composites (T6) is about 12.8% lower than the lowest water absorption among the glass-filled 30/70 composites (T10).
Effect of carbon filler
The effect of carbon filler content on the water absorption behavior of the composite laminates is given in . The water absorption is observed to reduce with the rise of carbon fillers content in the composite laminates. That is due to the hydrophobic nature of the carbon fillers and their possibility to reduce the void content in the composite (Ganapathy et al. Citation2021). The lowest percentage of water absorption (2.9%), which represents a reduction of about 55.4%, was observed in the 10 C 20/80 composite (T14). All carbon powder-filled 20/80 composites showed a lower percentage of water absorption than the 30/70 composites with the same amount of carbon fillers. The second-lowest percentage of water absorption (3.2%) was observed in the 10 C 30/70 composite (T18). As can be seen in , the carbon powder-filled composites presented a lower percentage of water absorption than the glass powder-filled composites. For instance, laminate T14, which exhibited the lowest percentage of water absorption among the carbon-filled composites, showed about 14.7% lower percentage of water absorption than laminate T6, which displayed the lowest percentage of water absorption among the glass-filled composites.
Tensile strength
The tensile strengths of eighteen laminates are illustrated in and 5.
Figure 4. Effect of glass fillers on tensile strength: (a) 20/80 composites and (b) 30/70 composites.

Effect of glass filler
The effect of glass fillers, at various mass fractions, on the tensile strength is shown in . The addition of glass fillers in the composites increased the tensile strength of the composites. The maximum tensile strength among the 20/80 composites was obtained for the composite with 7.5 wt.% glass fillers (T5); whereas, for the 30/70 composites, the maximum value was attained for the composite with 5 wt.% glass fillers (T8). That is due to the volume of polyester for the 20/80 composites is higher than the 30/70 composites which allowed the 20/80 composites to add glass fillers up to 7.5 wt.% before the tensile strength started to decline due to improper bonding. On the other hand, the tensile strength for the 30/70 composites was observed to decrease after the glass powder exceeds 5 wt.%. The reason for decreasing the tensile strength with further increment in the glass filler is the agglomeration of glass fillers in the polyester material and improper bonding between the matrix and reinforcement (Ganapathy et al. Citation2021; Reddy et al. Citation2019; Saiteja, Jayakumar, and Bharathiraja Citation2019). The maximum increment in tensile strength by the addition of glass fillers is 21.4% for the dry 20/80 composites and 12.7% for the dry 30/70 composites. The highest tensile strength was attained for the dry 5 G 30/70 composite (T8) which is about 15% higher than the maximum tensile strength among the 20/80 composites (T5). Thus, the addition of glass fillers in the 30/70 composites is preferable to the 20/80 composites for better tensile strength. It was observed that dry samples displayed better tensile strength than the corresponding wet samples. The reason is water absorption may lead to rapid debonding, delamination, and loss of structural integrity, thereby plasticizing the system and causing a reduction in the tensile strength (Tesfay, Kahsay, and Senthil Kumar Citation2022). Wet composites exhibit a similar tensile strength trend as that of their dry counterparts as shown in .
Effect of carbon filler
The effect of carbon fillers with varying weight fractions on the tensile strength of the composite is shown in . Like glass fillers, the addition of carbon fillers increased the tensile strength of the composites. Under the same weight fraction, the carbon powder-filled composites showed slightly higher tensile strength than the glass powder-filled composites as can be observed in . The effect of the content of carbon fillers on the tensile strength of the 20/80 and 30/70 composites followed the same trend as in the glass powder-filled composites. The highest tensile strength was observed in the dry 7.5 C 20/80 composite (T13) for the 20/80 composites and in the dry 5 C 30/70 composite (T16) for the 30/70 composites. The maximum increment in tensile strength is 24.2% for the dry 20/80 composites and 14.7% for the dry 30/70 composites. This is 2.8% higher for the 20/80 and 2% higher for the 30/70 composites than the corresponding glass powder-filled composites. Hence, carbon fillers are preferable hybrids to glass fillers for the higher tensile strength of the composites. Wet composites exhibit a similar tensile strength trend as that of their dry counterparts as shown in .
Impact strength
The impact strengths of eighteen laminates are presented in and 7.
Figure 6. Effect of glass fillers on impact strength: (a) 20/80 composites and (b) 30/70 composites.

Effect of glass filler
As shown in , glass fillers have increased the impact strength of the 20/80 and 30/70 composites. Like in the tensile strength, the maximum impact strength was attained in the composite with 7.5 wt.% filler composition (T5) for the 20/80 composites and in the composite with 5 wt.% filler composition (T8) for the 30/70 composites. Further increase in the glass fillers reduced the impact strength of the composites due to filler agglomeration in the polyester creates poor fiber – matrix interaction resulting in lower resistance to debonding (Biswas et al. Citation2019). However, none of the glass-filled composites have lower impact strength than the corresponding composite laminates without the glass fillers (T1 and T2). The impact strength exhibited a maximum improvement of 62.3% for the dry 20/80 composites and 46.2% for the dry 30/70 composites through the inclusion of glass fillers. The maximum impact strength among the glass-filled composites was presented for the dry 5 G 30/70 composite (T8) which is 13.4% higher than the maximum value among the glass-filled 20/80 composites (T5). Thus, the addition of glass fillers to the 30/70 composites is better than the 20/80 composites for better impact strength. Wet composites exhibit a similar impact strength trend as their dry counterparts as shown in .
Effect of carbon filler
The effects of carbon fillers content on the impact strength of the 20/80 and 30/70 composites are demonstrated in . The impact strength increased up to the addition of 7.5 wt.% of carbon fillers for the 20/80 composites and up to 5 wt.% carbon fillers for the 30/70 composites. Further increase of fillers decreased the impact strength due to the agglomeration of carbon fillers in the composite (Biswas et al. Citation2019). A maximum impact strength improvement of 78.5% for the dry 20/80 composites and 57.3% for the dry 30/70 composites was achieved through the inclusion of carbon fillers in the composites. Similar to the glass-filled composites, the maximum impact strength among the carbon-filled composites was presented for the dry 5 C 30/70 composite (T16) which is 11% higher than the maximum value among the carbon-filled 20/80 composites (T13). That indicates the addition of carbon fillers in the 30/70 composites is preferable to the 20/80 composites for better impact strength. Moreover, the maximum impact strength among the carbon-filled composites is 7.6% higher than the maximum value among the glass-filled composites. Wet composites exhibit a similar impact strength trend as their dry counterparts as shown in . Surprisingly, all carbon-filled wet specimens showed higher impact strength than the corresponding glass-filled dry specimens. Thus, carbon fillers are preferable hybrids to glass fillers for higher impact strength in dry and wet conditions of the composites.
Degradation of composites
Water absorption by the composites caused degradation in the tensile and impact strengths of the composites as shown in . That is due to water absorption cause rapid debonding, delamination, and loss of structural integrity, thereby plasticizing the system and cause a reduction in the tensile and impact strengths of the composites (Tesfay, Kahsay, and Senthil Kumar Citation2022). The percentage of degradation was highest in the laminates without fillers (T1 and T2) as shown in . The highest percentage of degradation in tensile and impact strengths were 13.5% and 18.9% respectively for the 30/70 composites (T2). The second-highest degradation was displayed for the 20/80 composites (T1). Laminates T2 has shown higher degradation than T1 due to the presence of a higher amount of hydrophilic sisal fibers in laminates T2 increased their water absorption rate which is the cause for degradation of the tensile and impact strengths of the composites. The addition of glass and carbon fillers decreased the degradation of the composites. The degradation of the tensile and impact strengths kept decreasing up to the addition of 10 wt.% of glass fillers for both the 20/80 and 30/70 composites. Whereas, the reduction in degradation of the carbon-filled composites was up to the addition of 7.5 wt.% of carbon fillers and a further increase in the fillers content lead to an increment of the degradation of the tensile and impact strengths for both the 20/80 and 30/70 composites. That is due to the volume of carbon fillers is higher than the same weight fraction of glass fillers and require more resin to wet. Thus, the matrix is insufficient to create proper bonding between the matrix and reinforcements. The lowest degradation in tensile strength (2%), which represents about 82.1% reduction compared to T1 laminates, is displayed for the 7.5 wt.% carbon-filled 20/80 composites (T13). The second lowest degradation (2.1%), which represents about 81.3% reduction compared to T1 laminates, is exhibited for the 10 wt.% glass-filled 20/80 composites (T6). Similarly, the lowest degradation in impact strength (2%), which represents about 88.2% reduction compared to T1 laminates, is presented for both the 7.5 wt.% and 10 wt.% carbon-filled 20/80 composites (T13 and T14). The third lowest degradation (2.2%), which represents about 87% reduction compared to T1 laminates, is exhibited for the 10 wt.% glass-filled 20/80 composites (T6). The 20/80 hybrid composites have shown lower degradation than the corresponding 30/70 hybrid composites as shown in . Nevertheless, the values of the tensile strength of the wet 30/70 hybrid composites are higher than all the corresponding wet and dry 20/80 hybrid composites as shown in . Moreover, the impact strength of the wet 30/70 hybrid composites is higher than all the wet and most of the dry corresponding 20/80 hybrid composites as shown in . For instance, the highest tensile strength among the wet 30/70 hybrid composites (T16) is about 11.4% higher than the highest tensile strength among the dry and wet 20/80 hybrid composites (T13). Furthermore, the highest impact strength among the wet 30/70 hybrid composites (T16) is about 6.3% higher than the highest impact strength among the dry and wet 20/80 hybrid composites (T13). Therefore, like in the dry conditions, the addition of fillers to the 30/70 composites is preferable to the 20/80 composites for a better performance of the composites in wet conditions. Carbon-filled composites have shown lower degradation than the corresponding glass-filled composites. For instance, the lowest degradation in tensile and impact strength of the carbon-filled composites (T13) is about 5% and 9%, respectively, lower than the lowest degradation in the glass-filled composites (T6).
Conclusion
The hybridization of sisal/polyester composite with carbon and glass fillers at an appropriate mass fraction showed enhanced mechanical and water absorption that can be utilized in various engineering applications like automotive, water tankers, and structural applications. Overall, the following conclusions are drawn:
The impact strength, water absorption, and degradation properties were highly affected by the addition of fillers. Whereas, the tensile strength is moderately affected in comparison.
Carbon-filled composites showed better results for water absorption, tensile strength, impact strength, and degradation properties than glass-filled composites.
The 20/80 composites added more fillers than the 30/70 composites before the tensile and impact strength started to decline.
The addition of fillers in the 30/70 composites is preferable to the 20/80 composites for better tensile and impact strengths. The 20/80 composites are preferable for lower water absorption and degradation properties.
Highlights
The highlights of this research include:
A significant enhancement on tensile strength, impact strength, water absorption, and degradation properties of sisal fiber/polyester composites is achieved through the addition of carbon and glass fillers.
Comparative study between the effect of carbon and glass fillers on the water absorption, tensile strength, impact strength, and degradation properties showed better results for the carbon filled composites than the glass filled composites.
Investigation on the influence of carbon and glass fillers’ amount on the impact strength, tensile strength, water absorption, and degradation properties of the composites presented highest tensile and impact strengths for the 5 wt.% carbon filled 30/70 composite, lowest water absorption for the 10 wt.% carbon filled 20/80 composite, and lowest degradation of 2% in tensile and impact strengths for the 7.5 wt.% carbon filled 20/80 composites.
Addition of carbon and glass fillers in the 30/70 composites is preferable to the 20/80 composites for better tensile and impact strengths; whereas, adding the powders in the 20/80 composites are preferable for lower water absorption and degradation properties.
Acknowledgements
The authors would like to appreciate the School of Mechanical and Industrial Engineering and School of Civil Engineering at Ethiopian Institute of Technology Mekelle (EIT-M), Mekelle University for providing us with materials, laboratory service, and research funding.
Disclosure statement
No potential conflict of interest was reported by the authors.
References
- Abhishek, S., M. R. Sanjay, R. George, S. Siengchin, J. Parameswaranpillai, and C. I. Pruncu. 2018. Development of new hybrid Phoenix pusilla/carbon/fish bone filler reinforced polymer composites. Journal of the Chinese Advanced Materials Society 6 (4):553–14. doi:10.1080/22243682.2018.1522599.
- Altay, L. 2019. The effect of hybrid carbon fillers on mechanical, thermal, and electrical properties of polyester composites. Emerging Materials Research 8:1–6. doi:10.1680/jemmr.18.00128.
- Asfaw, K. G. 2011. Investigation of the reasons for the unique growth and development of agave species (Agave sisalana and Agave americana) crop plants at the southern, central, north western and eastern parts of Tigray, Ethiopia. Current Research Journal of Biological Sciences 3 (4):273–81.
- ASTM D3039/D3039M. 2014. Standard test method for tensile properties of polymer matrix composite materials. West Conshohocken, PA: ASTM International.
- ASTM D 570–98. 1998. Standard test method for water absorption of plastics. PA, USA: ASTM International.
- Biswas, B., B. Hazra, A. Sarkar, N. R. Bandyopadhyay, B. C. Mitra, and A. Sinha. 2019. Influence of ZrO2 incorporation on sisal fiber reinforced unsaturated polyester composites. Polymer Composites 40:2790–801. doi:10.1002/pc.25087.
- Borba, P. M., A. Tedesco, and D. M. Lenz. 2014. Effect of reinforcement nanoparticles addition on mechanical properties of SBS/Curauá fiber composites. Materials Research 17:412–19. doi:10.1590/S1516-14392013005000203.
- Chandgude, S., and S. Salunkhe. 2021. In state of art: Mechanical behavior of natural fiber-based hybrid polymeric composites for application of automobile components. Polymer Composites 42:2678–703. doi:10.1002/pc.26045.26045.
- Chaudhary, V., A. K. Rajput, and P. K. Bajpai. 2017. Effect of particulate filler on mechanical properties of polyester based composites. Materials Today: Proceedings 4:9893–97. doi:10.1016/j.matpr.2017.06.289.
- Das, S. C., A. -E.K. Sheikh, M. A. Sayeed, Suruzzaman, D. Paul, S. A. Dhar, S. A. Grammatikos. 2020. On the use of wood charcoal filler to improve the properties of natural fiber reinforced polymer composites. Materials Today 44:926–29. doi:10.1016/j.matpr.2020.10.808.
- Devnani, G. L., and S. Sinha. 2019. Effect of nanofillers on the properties of natural fiber reinforced polymer composites. Materials Today: Proceedings 18:647–54. doi:10.1016/j.matpr.2019.06.460.
- Dinesh, S., P. Kumaran, S. Mohanamurugan, R. Vijay, D. L. Singaravelu, A. Vinod, M. R. Sanjay, S. Siengchin, and K. S. Bhat. 2020. Influence of wood dust fillers on the mechanical, thermal, water absorption and biodegradation characteristics of jute fiber epoxy composites. Journal of Polymer Research 27:1–13. doi:10.1007/s10965-019-1975-2.
- Fiore, V., G. D. Bella, and A. Valenza. 2015. The effect of alkaline treatment on mechanical properties of kenaf fibers and their epoxy composites. Composites Part B: Engineering 68:14–21. doi:10.1016/j.compositesb.2014.08.025.
- Ganapathy, T., R. Sathiskumar, M. R. Sanjay, P. Senthamaraikannan, S. S. Saravanakumar, J. Parameswaranpillai, and S. Siengchin. 2021. Effect of graphene powder on banyan aerial root fibers reinforced epoxy composites. Journal of Natural Fibers 18 (7):1029–1036.
- Gupta, M. K., and V. Deep. 2019. Effect of water absorption and stacking sequences on the properties of hybrid sisal/glass fiber reinforced polyester composite. Journal of Materials: Design and Applications 233 (10):2045–56. doi:10.1177/1464420718811867.
- ISO 179-1. 2010. Plastics – Determination of charpy impact properties – Part 1: Non-instrumented impact test. Geneva, Switzerland: International Organization for Standardization.
- Jarukumjorn, K., and N. Suppakarn. 2009. Effect of glass fiber hybridization on properties of sisal fiber–polypropylene composites. Composites Part B: Engineering 40 (7):623–27. doi:10.1016/j.compositesb.2009.04.007.
- Jenish, I., A. F. Sahayaraj, V. Suresh, J. M. Raj, M. Appadurai, E. F. I. Raj, O. Nasif, S. Alfarraj, and A. K. Kumaravel. 2022. Analysis of the hybrid of mudar/snake grass fiber-reinforced epoxy with nano-silica filler composite for structural application. Advances in Materials Science and Engineering 2022:1–10. doi:10.1155/2022/7805146.
- Melkamu, A., M. B. Kahsay, and A. G. Tesfay. 2019. Mechanical and water-absorption properties of sisal fiber (Agave sisalana)-reinforced polyester composite. Journal of Natural Fibers 16 (6):877–85. doi:10.1080/15440478.2018.1441088.
- Mohan, K., and T. Rajmohan. 2018. Effects of MWCNT on mechanical properties of glass-flax fiber reinforced nano composites. Materials Today: Proceedings 5:11628–35. doi:10.1016/j.matpr.2018.02.133.
- Nayak, S., R. K. Nayak, and I. Panigrahi. 2021. Effect of nano-fillers on low-velocity impact properties of synthetic and natural fibre reinforced polymer composites- a review. Advances in Materials and Processing Technologies 8:2963–86. doi:10.1080/2374068X.2021.1945293.
- Prasob, P. A., and M. Sasikumar. 2018. Static and dynamic behavior of jute/epoxy composites with ZnO and TiO2 fillers at different temperature conditions. Polymer Testing 69:52–62. doi:10.1016/j.polymertesting.2018.04.040.
- Premnath, A. A. 2019. Impact of surface treatment on the mechanical properties of sisal and jute reinforced with epoxy resin natural fiber hybrid composites. Journal of Natural Fibers 16 (5):718–28. doi:10.1080/15440478.2018.1432002.
- Reddy, P. V., P. R. Prasad, D. M. Krishnudu, and P. Hussain. 2019. Influence of fillers on mechanical properties of Prosopis juliflora fiber reinforced hybrid composites. Materials Today: Proceedings xxx: xxx-xxx. doi:10.1016/j.matpr.2019.07.618.
- Reddy, B. M., R. M. Reddy, B. C. M. Reddy, P. V. Reddy, H. R. Rao, and Y. V. M. Reddy. 2020. The effect of granite powder on mechanical, structural and water absorption characteristics of alkali treated cordia dichotoma fiber reinforced polyester composite. Polymer Testing 91:1–14. doi:10.1016/j.polymertesting.2020.106782.
- Saiteja, J., V. Jayakumar, and G. Bharathiraja. 2019. Evaluation of mechanical properties of jute fiber/carbon nano tube filler reinforced hybrid polymer composite. Materials Today: Proceedings xxx: xxx-xxx. doi:10.1016/j.matpr.2019.10.110.
- Sarker, F., N. Karim, S. Afroj, V. Koncherry, K. S. Novoselov, and P. Potluri. 2018. High-performance graphene-based natural fiber composites. ACS Applied Materials & Interfaces 10: xxx-xxx. doi: 10.1021/acsami.8b13018.
- Tesfay, A. G., M. B. Kahsay, and P. S. Senthil Kumar. 2020. Effects of chemical treatment, hybridization, and hybrid fiber stacking sequence and orientation on tensile and impact strength of continuous sisal fiber reinforced polyester composites. Journal of Natural Fibers 19 (7):2619–2631 . doi:10.1080/15440478.2020.1821283.
- Tesfay, A. G., M. B. Kahsay, and P. S. Senthil Kumar. 2022. Improvement of the degradation of tensile and impact strength of water-aged sisal fiber-reinforced polyester composites: A comparative study on the effects of hybridizations, hybrid layering sequences, and chemical treatments. Journal of Natural Fibers 19 (15): 11597–11609.
- Xanthos, M. 2010. Functional fillers for plastics. Hoboken: John Wiley & Sons.
- Yahaya, R., S. M. Sapuan, M. Jawaid, Z. Leman, and E. S. Zainudin. 2015. Effect of layering sequence and chemical treatment on the mechanical properties of woven kenaf–aramid hybrid laminated composites. Materials & Design 67:173–79.