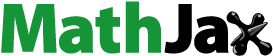
ABSTRACT
Plant-derived natural fibers have recently been used as a promising reinforcement in polymer matrix composites. Fibers from the inner sponge of the luffa cylindrica fruit have been the subject of several studies as reinforcement in various polymer resins. However, no research has been done on the Sponge Gourd Outer Skin Fiber (SGOSF). The present work is aimed to extract and characterize the chemical and physical properties of novel natural lignocellulosic fiber obtained from the outer skin (waste material) of the matured Luffa Cylindrica plant fruit. Characteristic studies on chemical composition, chemical structure, chemical compounds, thermal stability, and morphology of luffa cylindrica outer skin fibers (SGOSF) were investigated. The results revealed that SGOSF had cellulose (81.69 wt. %), lignin (10.14 wt. %), wax (0.22 wt.%), and ash content (1.18 wt. %). The density of the SGOSF was reported as 1.394 g/cm3. Thermal study results indicate SGOSF were withstanding the temperature up to 320°C. Fourier transform infrared analysis (FTIR) was done to determine the molecular structure of SGOSFs. The SGOSFs show tensile strength of 438.5 MPa. These results revealed that SGOSFs could be a sound reinforcement in the polymer matrix composites for various applications.
摘要
植物来源的天然纤维最近被用作聚合物基复合材料中一种很有前途的增强材料. 丝瓜果实内部海绵中的纤维作为各种聚合物树脂的增强材料,已经成为多项研究的主题. 然而,目前还没有对海绵葫芦外皮纤维(SGOSF)进行研究. 本工作旨在从成熟的丝瓜(Luffa Cylindrica)植物果实的外皮(废料)中提取并表征新型天然木质纤维素纤维的化学和物理性质. 对丝瓜外皮纤维(SGOSF)的化学成分、化学结构、化学成分、热稳定性和形态进行了表征研究. 结果表明,SGOSF具有纤维素(81.69 wt%)、木质素(10.14 wt%)、蜡(0.22 wt%)和灰分(1.18 wt%). 据报道,SGOSF的密度为1.394 g/cm3. 热研究结果表明,SGOSF能够承受高达320°C的温度. 采用傅立叶变换红外光谱分析(FTIR)方法测定了SGOSFs的分子结构. SGOSF的抗拉强度为438.5MPa. 这些结果表明,SGOSFs可以在各种应用的聚合物基复合材料中起到声音增强作用.
Introduction
Natural fiber composite materials have been the focus of research in recent days as a replacement for synthetic fiber composites due to increasing demand and environmental awareness among people globally (Sathish et al. Citation2021; Vigneshwaran, Jenish, and Sivasubramanian Citation2014). In the past decades, synthetic fibers (glass, carbon, and aramid) have been used as reinforcement in various polymer resin-based composites (Jenish et al. Citation2020; Sathish Gandhi et al. Citation2022). These synthetic fibers have several drawbacks, such as being non-degradable and difficult to disposable after end of the use. Natural fibers are a potential alternative for synthetic fibers because of their superior properties, which include low density, economic, environmental friendliness, and non-corrosiveness. In addition to that, natural fibers are renewable, biodegradable, easily available, and inexpensive to process. Natural fiber-reinforced laminates are increasingly used in numerous engineering industries, including automobiles, building materials, aircraft, marine, and household products.
Luffa cylindrica (LC) is a tropical plant that comes under the cucurbitaceous family that produces cylindrically shaped fruit. Luffa fruit is naturally made of a fibrous vascular system, which is mostly unavailable in other natural fibers (Saeed and Iqbal Citation2013). The fruit consists of the following parts such as inner core and outer skin. Sponge lengths range from 150 to 250 mm. shows the matured luffa cylindrica fruit of the Cucurbitaceae plant luffa cylindrica with the inner core and outer skin (Tanobe et al. Citation2005). Many studies have used the inner sponge of the luffa cylindrica fruit as a reinforcing material in various polymer matrices (Kocak et al. Citation2015). However, no research has characterized the outer skin fiber of the luffa cylindrica fruit. Luffa inner sponge processing industries threw this outer cover material as waste. The recently acknowledged Luffa Cylindrica (LC) outer skin fiber characterization was not done so far. Luffa cylindrica plant fruit fibers are attractive because they can grow in drylands and can be grown up quickly. Fibers extracted from luffa fruits have a higher specific strength and are renewable and biodegradable. (Nagaraja and Muralikannan Citation2016) reported that luffa cylindrica inner sponge fiber consists of 63% of cellulose, 19.4% of hemicellulose, 11.2% of lignin, 3.2% of wax, and 0.4% of ash. These fibers are also used as a filter in the diesel engine and as insulation material in biological compounds (Bal, Bal, and Lallam Citation2016). Luffa fruits are also used in the medicinal field to cure fever, enteritis, and diabetes. From this, it was concluded that luffa fruit fibers have wide applications in the engineering and biotechnology sectors (Shendge and Belemkar Citation2018). The sponge gourd has better properties when it is subjected to chemical treatments such as NaOH, silane, Ca (OH)2, and methacrylamide, which increases the tensile strength, cellulose content, and bonding strength and decreases the fiber density of the fiber since the enormous amount of wax and lignin has been removed from the fiber (Kalusuraman et al. Citation2019; Tanobe et al. Citation2014). Recently, epoxy was reinforced with sponge fibers. The composite has better properties at 40 wt.% of fiber reinforcement (Felix Sahayaraj and Muthukrishnan Citation2022).
Figure 1. (a) Luffa cylindrica flower, (b) luffa cylindrica young fruit, (c) matured luffa cylindrica fruit, (d) fibers in the outer shell of the sponge gourd.

A detailed characterization of fiber is extremely beneficial when looking for new applications. Many interfacial phenomena, such as adsorption, wetting, and adhesion, are caused by the surface composition of elements in polymer composites. Therefore, it is necessary to define and evaluate the properties and quality of sponge gourd outer skin fiber in order to use this as reinforcement. Various analytic techniques were used to characterize SGOSFs in this study. Chemical analysis, physical properties, XRD, FTIR spectroscopy, tensile testing, TGA, water absorption test, and SEM were used to report the physicochemical properties of SGOSFs for the first time. This research aims to address essential fiber properties in order to aid their increased use in current and future applications such as the automotive, structural, and packaging industries.
Materials and methods
Materials
Luffa Cylindrica (LC) is a lignocellulose plant that comes under the Cucurbitaceae family. Fibers were collected from the outer shell of matured Luffa Cylindrica plant fruit from Kannampalyam village, Coimbatore district of Tamilnadu, India. Extracted sponge gourd outer skin fiber (SGOSF) is shown in . The fibers were peeled out manually from the waste outer shell. The extracted outer shell fibers were rinsed with fresh tap water to remove the unwanted impurities attached to the fiber. The cleaned fibers were dried in the micro-oven for 72 hours and then underwent several tests for characterization. The fiber extraction process is shown in .
Chemical analysis of SGOSFs
Extracted SGOSFs were dried using the oven at 110°C and then placed in a desiccator to prevent moisture. The chemical composition of SGOSFs was determined by standard testing methods. The cellulose amount was measured by Kurshner and Hoffer’s method, and the Klason lignin Method determined the lignin amount. SGOSF ash amount was calculated as per standard (ASTM E1755–01) (Fagerstedt et al. Citation2015). An electronic moisture analyzer was employed to find out moisture content. Conrad method was used to measure the wax content of SGOSF.
Physical analysis of SGOSF
The SGOSF samples were prepared according to the ASTM D792 standard to measure the density using a pycnometer (Kader and Deeb Citation2020). Kerosene was taken as immersion fluid, and density was measured according to EquationEquation 1(1)
(1) .
where ρSGOSFs represents the density of SGOSF; ρK represents the density of kerosene; m1- mass of empty pycnometer; m2- mass of pycnometer filled with SGOSF; m3- the mass of pycnometer filled with kerosene; m4- the mass of pycnometer with SGOSF and kerosene (Béakou et al. Citation2008).
XRD analysis
The XRD analysis was primarily used to identify the chemical structure and crystallinity index (CI) of the SGOSF. Xpert PRO-PANalytical system (Cu Ka Radiation = 0.154 nm; step size = 0.0130; Temperature = 25°C; Generator Settings = 30 mA, 45 kV) was employed to find out the crystallinity of SGOSF. The sample was taken in powder form. The following equation (EquationEquation 2(2)
(2) ) was used to determine the crystallinity index (CI).
The intensities of the graph at 2θ = 22.60° and 18.77° were denoted as H22.60 and H18.77, respectively. Scherrer’s formula (Muvvala et al. Citation2019) was used to determine the crystalline size (CS) in EquationEquation 3(3)
(3) .
Where K- Scherrer’s constant (0.89), λ represents the wavelength of the radiation, β represents the intensities full width, and θ-Bragg angle.
FTIR analysis
FITR Spectroscopy was used to identify the functional groups of SGOSF. FTIR apparatus (Miracle 10 Shimadzu) with a DTGs detector was used to record the spectrum. SGOSF was chopped into small fibers and converted to micro-level particles through a ball mill. This powdered fiber sample was combined with potassium bromide (2.5 mg sample per 250 mg KBr) and then pelletized using a mini hydraulic press. FTIR spectrum was noted from 4000 cm−1 to 500 cm−1 with 16 cm−1 resolution, and 14,000 scans were received for the analysis (Sudharsan et al. Citation2016).
TGA analysis
TGA analysis to determine the thermal stability of the SGOSF in order to use it as reinforcement in polymer composite. The thermal study was done using Thermogravimetric Analyzer (EXSTAR TG/DTA 6300). Finely powdered fiber samples were initially weighed and placed in an alumina crucible pan, then the temperature was raised up to 1000°C at a constant heat value of 20°C/min (Lee, Joo, and Radovic Citation2003). The setup was maintained in a nitrogen gas environment to avoid oxidation. Nitrogen gas was continuously supplied within the chamber with a flow rate of 20 ml/min. At constant intervals, weight loss and corresponding temperature were recorded.
Tensile properties
An INSTRON 3369 Universal Testing Machine was used to investigate the tensile properties (single fiber tensile test) of the sponge gourd outer skin fiber at standard atmospheric conditions. Fibers were prepared according to the ASTM D3379 standard. Twenty-five fiber samples (50 mm) were picked, and the average values were recorded. Microfibril angle was calculated by analyzing SEM images using Image J software.
SGOSF samples with a gauge length of 50 mm were fixed on thick paper to determine the tensile strength. The original gauge length of the fibers was confirmed with a vernier caliper then the fiber’s axis aligned with the UTM machine’s crosshead. The machine setup was loaded with 1N at the feed rate of 0.5 mm/min. The double faces of the paper frame were cut carefully without disturbing the whole setup. The entire setup was conducted at 24°C with 56% humidity (Ramesh et al. Citation2021).
Water absorption studies
SGOSFs were undergone water absorption studies to analyze the hydrophilic behavior of the samples. The predetermined weight of fiber samples was taken and dried using the oven at 60°C for about 3 hours. Weighed fiber samples were immersed in a freshwater sample taken from a nearby lake (Kannampalayam village, Coimbatore District, Tamilnadu, India) at room temperature. The fiber samples were taken out and cleaned with the dry cotton cloth at a constant interval, and a corresponding weight change was recorded. The water absorption percentage of SGOSF was calculated using EquationEquation 4(4)
(4) .
Where, Wo- the weight of the sample before immersion, and Wt- the weight of the sample after immersion
Surface morphology
The surface morphology of SGOSF was analyzed using a SEM in the model of MIRA3 TESCAN. The setup was running at 5–10 kV operating voltage. The surface and diameter of SGOSFs were studied at various zoom levels. Before SEM inspection, SGOSF samples were sputter-coated with gold in order to make the sample inhibit charging, reduce thermal damage and enhance the quality of SEM images.
The diameter of the SGOSF depends upon various factors such as plant age, cultivation environment, soil condition, and extraction method. It is very difficult to calculate the diameter of non-uniformly shaped cellulosic fiber. SGOSFs are nearly cylindrically shaped, so the diameter was taken in the fibers’ upper, center, and lower parts, and the average values were recorded.
EDX analysis
SGOSFs elements (C-Carbon, O-oxygen, Mg-Magnesium, Na-sodium, etc.) were confirmed using SEM with EDX setup. The presented elements are tabulated in terms of atomic and weight percentage.
Results and discussions
SGOSFs chemical composition analysis
The chemical composition (cellulose, hemicellulose, lignin, wax, moisture, and ash content) of the extracted SGOSFs was reported and compared with the conventional fibers shown in . Soil conditions, plant age, and growing places influence the fiber chemical composition. Chemical composition of the fiber from the inner core of the sponge gourd to be used as reinforcement in polymer resins. According to research done by Yoldas et al. 2011 inner core consists of cellulose (63.00 wt.%), hemicellulose (14.40 wt.%), lignin (1.60 wt.%), ash (0.9 wt.%), and other elements (20 wt.%). In this study, SGOSF had cellulose (81.69 wt. %), lignin (10.14 wt. %), wax (0.22 wt. %), and ash content (1.18 wt. %). The tensile strength, thermal stability, crystallinity index, non-flammability and biological decomposability of the SGOSF are influenced by the chemical elements present in the fiber. The results showed that the SGOSF has higher cellulose content (81.69 wt. %), enhancing the fiber strength and stiffness. The lignin content (10.14 wt. %) of SGOSF is higher than the luffa inner core fiber (1.60 wt. %). This amorphous lignin matrix bound the SGOSF microfibrils and act as protection agent against fungal attack (Manimaran et al. Citation2019). Higher lignin content in the fiber improves the biocompatible nature of the polymer composites. Simultaneously, the wax amount was lower than the sponge gourd inner core fiber. Generally, higher wax content reduces the interfacial bonding between matrix and fiber when fabricating the composites. Additionally, SGOSFs show lower moisture content, which assists in matrix-fiber adhesion. Higher amount of ash content was found, which shows that the fiber content has been increased due to the lower amount of the amorphous phase, such as hemicelluloses and wax content. The results revealed that SGOSF may be a suitable reinforcement in polymer resins.
Table 1. SGOSF properties compared with other natural fibers.
Physical properties
The density of SGOSF reported as 1.394 g/cm3 is comparatively lower than conventional natural fibers such as jute, hemp, and sisal. The low-density fiber was helpful in the fabrication of eco-friendly and lightweight applications. Density of the natural fiber plays the vital role in the weight of the composite while reinforcing with polymer resins.
XRD analysis
XRD spectrogram of SGOSF is shown in . From the graph, two valleys were identified clearly. The crystalline intensities are observed at 2θ = 22.60° which represent the (0 0 2) crystalline plane (I002) and amorphous intensities is observed at 2θ = 18.77° which represent (Iam). The crystalline index is the parameter used to calculate the crystalline content in cellulose.
SGOSF crystalline index was reported as 41.72%, which is higher than the other conventional fibers such as jute (34.3%) and kenaf (34.9%) (Ramamoorthy et al. Citation2017). The increased CI value of SGOSF is due to the absence of hemicellulose and lower amorphous phase content, such as lignin and pectin content. These high CI values also contribute to the mechanical and thermal properties. This is because, at higher CI value, cellulosic chains are aligned regularly, which helps to improve the tensile behavior of the reinforcement. As a result, fiber with matrix produces high-strength composites, and thermal degradation temperature also increased significantly. The crystalline size of the SGOSF was reported as 18.36 nm. The higher crystalline size lower the water-absorbing ability and chemical reactivity (Ramesh et al. Citation2021).
FTIR analysis
Chemical groups present in the extracted SGOSF can be identified using FTIR analysis. shows the recorded FTIR spectrum of SGOSF. The functional groups of SGOSF are reported in . FTIR results confirm the presence of major chemical compounds (cellulose, lignin, and wax) present in SGOSF, similar to the other conventional fibers such as jute, hemp, and sisal.
Table 2. Functional groups present in the SGOSF.
TGA analysis
TGA was employed on the SGOSFs in order to calculate the thermal stability. Assessing the thermal behavior of SGOSF is essential in order to find the temperature range for fabrication of composite and trace out when the fiber loses its properties. The graph plotted weight loss with the corresponding temperature at a constant time interval. The chemical composition (cellulose, hemicellulose, and lignin) of the fibers majorly affects thermal stability. The mass loss graph is given in . The Thermogram of extracted SGOSF is shown in . Thermograms were used to analyze the thermal behavior of the fibers at the temperature range higher than room temperature. The first mass loss was observed around 100°C due to the evaporation of moisture and other volatile content from SGOSF. Secondary mass loss due to the thermal degradation of hemicellulose and lesser amount of lignin and cellulose content at the temperature of 303°C with 28.6 wt. % of mass loss. The third step peak represents the thermal degradation of cellulose at 362°C. The fourth fall is due to the degradation of wax and ash formation observed at 656°C with an overall mass loss wt. % of 81.1% in SGOSF (Ramesh et al. Citation2020).
Tensile testing
The mechanical behavior of natural fibers mainly depends on the chemical structure and composition. Cellulose-based natural fibers show distinct mechanical properties, confirmed by various literature studies. The variation in mechanical properties is mainly due to plant characteristics, test methods, extraction technique, harvesting age, defects in fiber structure, etc.
The tensile test reports show that SGOSF has a tensile strength of 438.5 MPa is higher than other conventional fibers. The main reason for this increased tensile strength is rich cellulose content and low fibril angle because cellulose contents in the fiber have linear orientation and a high degree of polymerization. The SGOSFs calculated microfibril angle (9.46°–12.18°) provided excellent tensile strength. SGOSF consists of the cell wall that is twisted from cellulose microfibrils embedded in hemicellulose and lignin matrix.
Water absorption behavior of SGOSF
The water absorption behavior of SGOSF is shown in . According to (Melo, Lia Fook, and Lima Citation2021), it follows non-Fickian behavior. Initially, the water absorption rate of the fiber increases linearly with time. The main reason for this increasing trend is that SGOSF has voids, cracks, and rough surfaces through which water penetrates and swelling of fiber results in reduced mechanical properties. Saturation may have formed after a while, as depicted by the graph’s steady upward trend.
SEM analysis
The surface morphology of SGOSF is shown in at various magnification levels of 100 and 200 ×. SEM images showed that the fiber has a rough surface. The rough surface of the SGOSF indicates that the fibers might have excellent bonding strength while making the polymer composites. It is observed that some dust and waste particle were attached to the fiber because the fiber does not undergo any chemical treatments. Voids and microcracks available on the SGOSF surface are indicated in .
EDX analysis
EDX analysis of SGOSFs is shown in Figure 10. The elements present in the SGOSF were reported in terms of atomic and weight percentage. The EDX results show that the fiber has a higher percentage of carbon and oxygen. provides information on the percentages of a number of elements. The table shows the extracted SGOSF consists of 39.4 wt.% of carbon and 49.6% of oxygen. The analysis shows that the SGOSF consists of less carbon content due to less amount of non-cellulose elements in the fiber. It is also noticed that the amount of oxygen is higher in the fiber due to more cellulose content. The presence of other elements, such as magnesium, silicon, calcium, and sulfur, is due to raw SGOSF. These elements will have vanished when the fiber is subjected to chemical treatments.
Table 3. Elements present in the surface of SGOSF.
Conclusions
This study examined the extraction and characterization of novel SGOSFs, including chemical composition, crystalline index (CI), crystalline size (CS), chemical groups, thermal stability, and tensile properties. Due to its high cellulose content (81.69 wt.%) SGOSF shows higher tensile strength (438.5 MPa). SGOSFs have a lower density (1.394 g/cm3) and are suitable for lightweight composite fabrications. In addition, TGA analysis confirms that the fiber can be suitable for composite application and withstand heat up to 320°C. SGOSF has a low density, a superior chemical composition, a medium crystallinity index, and good thermal characteristics, according to the physicochemical characterization data. FTIR studies confirm the existence of cellulose, hemicellulose, and lignin components. XRD analysis gives a higher crystallinity index (41.72%) and crystalline size (18.36 nm) of the SGOSFs. Based on the findings, SGOSFs might be used as a reinforcing material in composites for various applications, including automobile interior panels, door panels, and packaging materials. It may be concluded that Luffa cylindrica fibers are adaptable and have technical potential to be used as reinforcement polymer matrices with enhanced properties.
Highlights
It is a novel work of Sponge Gourd Outer Skin Fiber characterization, which is obtained from the plant of luffa cylindrica fruit outer skin.
Fiber has been characterized for the application of polymer composite materials since the requirements of fiber for the polymer composite are essential.
Characteristic studies on chemical composition, chemical structure, chemical compounds, thermal stability, and morphology of luffa cylindrica outer skin fibers (SGOSF) have been investigated.
Data availability
The data used to support the findings of this study are included within the article.
Acknowledgements
The authors thank to the KIT-Kalaignarkarunanidhi Institute of Technology for giving all the facilities to complete this work. The authors appreciate the support from Seenu Atoll School, Addu City, Maldives, for the research and preparation of the manuscript.
Disclosure statement
No potential conflict of interest was reported by the authors.
Additional information
Funding
References
- Bal, K. E., Y. Bal, and A. Lallam. 2016. Gross morphology and absorption capacity of cell-fibers from the fibrous vascular system of loofah (luffa cylindrica). Textile Research Journal 74 (3):241–14.
- Béakou, A., R. Ntenga, J. Lepetit, J. A. Atéba, and L. O. Ayina. 2008. Physico-chemical and microstructural characterization of “rhectophyllum camerunense” plant fiber. Composites Part A, Applied Science and Manufacturing 39 (1):67–74. doi:10.1016/j.compositesa.2007.09.002.
- Fagerstedt, K. V., P. Saranpää, T. Tapanila, J. Immanen, J. A. A. Serra, and K. Nieminen. 2015. Determining the composition of lignins in different tissues of silver birch. Plants 4 (2):183–95. doi:10.3390/plants4020183.
- Felix Sahayaraj, A., and M. Muthukrishnan. 2022. Experimental investigation on the effect of fiber volume fraction of sponge gourd outer skin fiber reinforced epoxy composites. doi:10.1002/pc.26754.
- Fu, S., P. Song, and X. Liu. 2017. Thermal and flame retardancy properties of thermoplastics/natural fiber biocomposites. Advanced High Strength Natural Fibre Composites in Construction. doi:10.1016/B978-0-08-100411-1.00019-4.
- Jenish, I., V. C. Sathish Gandhi, S. Basavarajappa, S. Indran, D. Divya, Y. Liu, M. R. Sanjay, and S. Siengchin. 2020. Tribo-mechanical characterization of carbonized coconut shell micro particle reinforced with cissus quadrangularis stem fiber/epoxy novel composite for structural application. Journal of Natural Fibers. doi:10.1080/15440478.2020.1838988.
- Kader, M. M. A., and A. S. Deeb. 2020. Preparation and characterization of low-cost water proofing sheets from NR-loaded clay. HBRC Journal 16 (1):1–15. doi:10.1080/16874048.2019.1688073.
- Kalusuraman, G., I. Siva, S. Mustafa Aslan, S. Anandkumar, and K. G. Satyanarayana. 2019. Evaluation of surface pre-treatments on the surface characteristics modifications in sponge gourd (luffa cylindrica) fibers. Materials Research Express 6 (9):095–106.
- Kocak, D., S. I. Mistik, M. Akalin, and N. Merdan. 2015. The use of luffa cylindrica fibres as reinforcements in composites. Biofiber Reinforcements in Composite Materials. doi:10.1533/9781782421276.5.689.
- Lee, Y. J., H. J. Joo, and L. R. Radovic. 2003. Preferential distribution and oxidation inhibiting/catalytic effects of boron in carbon fiber reinforced carbon (CFRC) composites. Carbon 41 (13):2591–600.
- Malkapuram, R., V. Kumar, and Y. Singh. 2009. Recent development in natural fiber reinforced polypropylene composites. Journal of Reinforced Plastics and Composites 28 (10):1169–89. doi:10.1177/0731684407087759.
- Manimaran, P., S. P. Saravanan, M. R. Sanjay, S. Siengchin, M. Jawaid, and A. Khan. 2019. Characterization of new cellulosic fiber: Dracaena reflexa as a reinforcement for polymer composite structures. Journal of Materials Research and Technology 8 (2):1952–63. doi:10.1016/j.jmrt.2018.12.015.
- Melo, R. Q. C., M. V. Lia Fook, and A. G. B. Lima. 2021. Non-fickian moisture absorption in vegetable fiber reinforced polymer composites: The effect of the mass diffusivity. Polymers 13 (5):761.
- Milanese, A. C., M. O. H. Cioffi, and H. J. C. Voorwald. 2012. Thermal and mechanical behaviour of sisal/phenolic composites. Composites Part B: Engineering 43 (7):2843–50.
- Muvvala, K., R. Surendra, M. S. Dutta, and R. Rao. 2019. Estimation of particle size using the debye equation and the scherrer formula for polyphasic TiO2 powder. Journal of Physics Conference Series 1410 (1):012057.
- Nagaraja, G. B., and R. Muralikannan. 2016. Extraction and characterization of lignocellulosic fibers from luffa cylindrica fruit. International Journal of Polymer Analysis and Characterization 21 (3):259–66. doi:10.1080/1023666X.2016.1146849.
- Ramamoorthy, S. K., D. Åkesson, M. Skrifvars, and B. Baghaei. 2017. Preparation and characterization of biobased thermoset polymers from renewable resources and their use in composites. Handbook of Composites from Renewable Materials 1–8:425–57.
- Ramesh, M., C. Deepa, M. Tamil Selvan, L. Rajeshkumar, D. Balaji, and V. Bhuvaneswari. 2021. Mechanical and water absorption properties of calotropis gigantea plant fibers reinforced polymer composites. Materials Today: Proceedings 46:3367–72. doi:10.1016/j.matpr.2020.11.480.
- Ramesh, M., C. Deepa, M. Tamil Selvan, and K. H. Reddy. 2020. Effect of alkalization on characterization of ripe bulrush (typha domingensis) grass fibers reinforced epoxy composites. Journal of Natural Fibers 19 (3):931–42. doi:10.1080/15440478.2020.1764443.
- Ramesh, M., L. Rajeshkumar, C. Deepa, M. Tamil Selvan, V. Kushvaha, and M. Asrofi. 2021. Impact of silane treatment on characterization of ipomoea staphylina plant fiber reinforced epoxy composites. Journal of Natural Fibers 19 (13):5888–99. doi:10.1080/15440478.2021.1902896.
- Saeed, A., and M. Iqbal. 2013. Loofa (luffa cylindrica) sponge: Review of development of the biomatrix as a tool for biotechnological applications. Biotechnology Progress 29 (3):573–600. doi:10.1002/btpr.1702.
- Sathish Gandhi, V. C., I. Jenish, S. Indran, and D. Y. Rajan. 2022. Mechanical and thermal analysis of cissus quadrangularis stem fiber/epoxy composite with micro-red mud filler composite for structural application. Transactions of the Indian Institute of Metals 75 (3):737–47. doi:10.1007/s12666-021-02478-1.
- Sathishkumar, T., P. Navaneethakrishnan, S. Shankar, R. Rajasekar, and N. Rajini. 2013. Characterization of natural fiber and composites – a review. Journal of Reinforced Plastics and Composites 32 (19):1457–76. doi:10.1177/0731684413495322.
- Sathish, S., L. Prabhu, S. Gokulkumar, N. Karthi, D. Balaji, and N. Vigneshkumar. 2021. Extraction, treatment and applications of natural fibers for bio-composites - a critical review. International Polymer Processing 36 (2):114–30.
- Shendge, P. N., and S. Belemkar. 2018. Therapeutic potential of luffa acutangula: A review on its traditional uses, phytochemistry, pharmacology and toxicological aspects. Frontiers in Pharmacology 9 (OCT):1177. doi:10.3389/fphar.2018.01177.
- Sudharsan, K., C. Chandra Mohan, P. B. Azhagu Saravana, G. Archana, K. Sabina, M. Sivarajan, and M. Sukumar. 2016. Production and characterization of cellulose reinforced starch (CRT) films. International Journal of Biological Macromolecules 83:385–95. doi:10.1016/j.ijbiomac.2015.11.037.
- Tanobe, V. O. A., T. H. S. Flores-Sahagun, S. C. Amico, G. I. B. Muniz, and K. G. Satyanarayana. 2014. Sponge gourd (luffa cylindrica) reinforced polyester composites: Preparation and properties. Defence Science Journal 64 (3):273–80. doi:10.14429/dsj.64.7327.
- Tanobe, V. O. A., T. H. D. Sydenstricker, M. Munaro, and S. C. Amico. 2005. A comprehensive characterization of chemically treated Brazilian sponge-gourds (luffa cylindrica). Polymer Testing 24 (4):474–82.
- Vigneshwaran, G., I. Jenish, and R. Sivasubramanian. 2014. Design, fabrication and experimental analysis of pandanus fibre reinforced polyester composite. Advanced Materials Research 984:253–56.