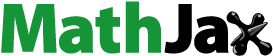
ABSTRACT
Natural fibers are available in low cost, easily renewable and they are rich in cellulose. As the natural fibers possess impurities over the surface, poor wettability and interfacial bonding are the main drawbacks encountered during fabrication process. In the current research work, hemp (H) and palmyra (P) fibers were treated with different sodium hydroxide (NaOH) concentrations (2%, 4%, 6%, and 8%) for 5 hrat room temperature. The influence of stacking sequence (HHPPHH, HPHPHP and PPHHPP) and surface modification and on physical, mechanical, and thermal properties of hemp and palmyra fiber-reinforced epoxy composites were investigated with a 30 wt.% of fiber loading. The attributes such as water absorption, density, tensile properties, flexural properties, impact energy, hardness, and thermal conductivity were examined. From the experimental results, the 6% NaOH-treated composites compared with untreated fiber composites disclosed an increase of 19.34% for tensile strength, 16.77% for flexural strength, and 12.35% for hardness with stacking sequence of PPHHPP. The experimental thermal conductivity results show that there is a significant decrease in values after NaOH treatment of hybrid composites. The surface morphology of the untreated, treated reinforcements, and fracture surface of the tensile-tested hybrid composite specimens were examined by scanning electronic microscope.
摘要
天然纤维成本低,易于可再生,并且富含纤维素. 由于天然纤维表面含有杂质,润湿性差和界面结合是制造过程中遇到的主要缺点. 在目前的研究工作中,大麻(H)和棕榈(P)纤维在室温下用不同浓度的氢氧化钠(2%、4%、6%和8%)处理5小时. 研究了堆叠顺序(HHPPHH、HPHPHP和PPHHPP)和表面改性对纤维负载量为30 wt.%的大麻和棕榈纤维增强环氧树脂复合材料的物理、机械和热性能的影响. 测试了吸水性、密度、拉伸性能、弯曲性能、冲击能、硬度和热导率等属性. 从实验结果来看,与未处理的纤维复合材料相比,6%NaOH处理的复合材料的拉伸强度增加了19.34%,弯曲强度增加了16.77%,硬度增加了12.35%. 实验热导率结果表明,经过NaOH处理后,杂化复合材料的热导率值显著降低. 通过扫描电子显微镜检查了未处理、处理的增强材料的表面形态和拉伸测试的混合复合材料试样的断裂表面.
Introduction
Due to the demand for eco-friendly and growing environmental awareness, the usage of natural fibers in composites fabrication were increasing day by day. Polymer composites reinforced with natural fibers have played an important role for a long time due to their merits such as low density, non-abrasive, biodegradability, high specific strength, and modulus and low cost (Christian and Billington Citation2011; Harry et al. Citation2011). The key problem in the application of natural fibers is weak adhesive bonding between the hydrophobic matrix and hydrophilic fiber. The high polar surface of natural fibers induces an incompatibility problem with many synthetic matrices, which imply poor mechanical properties in a composite material Pickering, Aruan Efendy, and Tan Minh (Citation2016). It is necessary to enhance the bonding between the reinforcement and matrix and fiber dispersion either by chemical or physical methods. Preliminary tests conducted by researchers have confirmed that alkali treatment has been found to be the most feasible one among various chemical treatments (Chandrasekar et al. Citation2017; Reddy, Yoganandam, and Mohanavel Citation2020; Xue, Tabil, and Panigrahi Citation2007).
Huang (Huang Citation2009) and Yan et al (Yan et al. Citation2016) investigated the influence of NaOH concentration and microstructure of coir fiber-reinforced polymer composites. From their study, it was observed using SEM that the coir fibers are clean and rough after 5 wt% NaOH treatment, which improvised the flexural strength and tensile strength by 16.7% and 17.8%, respectively. The influence of NaOH treatment on impact strength of sugar palm-reinforced polymer composites was evaluated by Bachtiar et al (Bachtiar, Sapuan, and Hamdan Citation2009). The results disclosed a rise in impact energy of alkali-treated fiber composite by 12.85% at 0.5 M NaOH concentration when compared with untreated composite. Enhancement of mechanical characteristics of hemp fiber-reinforced PLA biocomposites was reported by sawpan et al (Sawpan, Pickering, and Fernyhough Citation2011). From this study the composites with 35 wt% of fiber loading and 5% NaOH concentration displayed maximum impact energy of 7.4 KJ/m2 and tensile strength of 85.4 MPa. Mohana Krishnudu et al. (Mohana Krishnudu et al.) investigated the influence of NaOH concentration on mechanical and thermal properties of Abutilon Indicum fiber-reinforced composites and the results indicated that the composite made up of alkali-treated fibers possessed better mechanical properties. Meenakshi Reddy et al. (Meenakshi Reddy et al.) examined the mechanical behavior of Tapsi fiber-reinforced polyester composites at different NaOH concentration, and the properties were observed to be far and away superior when alkali-treated tapsi fibers were utilized as a part of the composites.
Yousif et al (Yousif et al. Citation2012) examined the flexural behavior of unidirectional long kenaf fiber-reinforced polymer composites at the concentration of 6% NaOH. The study disclosed an improvement in flexural properties of treated fiber-reinforced composite by 36% due to low porosity and better interfacial bonding of the composite. Haameem et al (Haameem et al. Citation2014) investigated the impact of NaOH treatment [5%, 10%, 15%, and 20%] on the tensile properties of Napier grass fibers. The results exhibited 88.3% growth in tensile strength of the single Napier reinforcement when compared to untreated fiber at 10% NaOH concentration. Suardana et al (Suardana, Piao, and Kyoo Lim Citation2011) evaluated the effects of silane water and sodium hydroxide treatment on hemp fiber-reinforced polypropylene composites. From the study, an increase in tensile strength, flexural strength, and good surface topology of treated fibers with 4% NaOH concentration when related to untreated fiber PP composites.
Surface modification effects on mechanical behavior of bamboo cellulose fiber-reinforced epoxy composites were examined by Lu et al (Tingju et al. Citation2013) and the results indicated that an improvement in tensile strength by 34% when the fibers were treated with NaOH aqueous solution at 20 wt% of fiber loading. The effect NaOH fiber surface treatment on visco-elastic and mechanical performance of banana epoxy composites was assessed by Venkateshwaran et al (Venkateshwaran, Elaya Perumal, and Arunsundaranayagam Citation2013). The assessment displayed an increase in flexural strength by 16.65%, tensile strength by 52%, and impact energy by 81.8% at 1% NaOH concentration treatment when related with untreated banana epoxy composite. Bachtiar et al (Bachtiar, Sapuan, and Hamdan Citation2010) examined the flexural properties of sugar palm reinforced composites at 10 wt% fiber loading subjected to NaOH treatment with 5% and 10% concentrations. The results exposed an increase in flexural strength by 24.41% at 5% concentration and flexural modulus by 148% at 10% concentration when compared with untreated fiber-reinforced composite. Owen (Owen Citation2014) investigated the effects of alkali treatment on mechanical properties of jute fabric-reinforced epoxy composites using two samples [A, B] of woven jute fabric. The results indicated an improvement in tensile and flexural strength by 27.88% and 17.82%, respectively, for sample A and 53.08% and 64.45% improvement for sample B, respectively. The hemp plant was easily grown and has comparatively short cropping cycle, in specific, which produces high quality natural fibers that show excellent mechanical properties and inexpensive when compared to other natural fibers.
In earlier study of authors, hemp and palmyra fiber-reinforced epoxy composites were fabricated with 10 wt.%, 20 wt.%, 30 wt.%, and 40 wt.% of fiber contents (Islam, Pickering, and Foreman Citation2010). Among different fiber contents, composites fabricated with 30 wt.% fiber loading showed better mechanical properties. Hence, in the present investigation, all the hybrid composites were fabricated with 30 wt.% fiber loading and the fibers are subjected to alkali treatment at different NaOH concentrations (2%, 4%, 6%, and 8%) to study the physical, mechanical, and thermal properties of hemp and palmyra fiber-reinforced hybrid composites.
Materials and methods
Materials
Mechanical performance of hybrid composites was extremely relied on direction of the fibers. Compared with the randomly aligned short orientations of fibers, the unidirectional fibers were giving better strength (Devireddy and Biswas Citation2017). In the current research work, the unidirectional hemp and palmyra fibers were selected as reinforcement material. The continuous palmyra fiber is extracted from outer bask of the palm tree and the unidirectional hemp fiber was purchased from the local commercial sources. The unidirectional hemp and palmyra fibers were cut into 30 cm length and prepared in layers form as shown in . The polymer matrix used is a combination of LY 556 Liquid Epoxy and HY 951 Hardener, which were provided by Sree industrial composite products, Hyderabad. The sodium hydroxide (NaOH) procured from the Thermo Fisher Scientific India Pvt. Ltd, India, was used for surface treatment of the hemp and palmyra fibers.
Fiber treatment
The main problem with untreated natural fibers is poor interfacial bonding between the matrix and reinforcement. To overcome this problem, the continuous hemp and palmyra fibers were drenched separately in four different alkali concentrations (2%, 4%, 6%, and 8%). These fibers were retained to immerse in the NaOH concentrations for 5 h at room temperature as recommended for better penetration of the NaOH solutions into the reinforcement material (Hamidon et al. Citation2019). shows the chemical treatment of hemp and palmyra fibers. The treated hemp and palmyra fibers were removed from the NaOH solution and thoroughly cleaned with running tap water to remove any excess of NaOH over the fiber surface. Finally, the cleaned hemp and palmyra fibers were dried in an oven at 70°C for 4 h to remove the remaining moisture content.
Composites preparation
The fabrication of hemp and palmyra fiber-reinforced hybrid composite laminates was done by a simple hand lay-up process. All the composite specimens were prepared with 30% fiber loading with four different alkali concentrations (2%, 4%, 6%, and 8%). The designation and NaOH concentration of fabricated composites specimens are listed in . Firstly, the continuous hemp and palmyra fibers were chopped into 30 cm length to prepare into a layer’s form. The layers were kept in three modes of stacking sequences HPHPHP (Hemp-Palmyra- Hemp-Palmyra-Hemp-Palmyra), HHPPHH (Hemp-Hemp-Palmyra-Palmyra-Hemp-Hemp), and PPHHPP (Palmyra-Palmyra-Hemp-Hemp-Palmyra-Palmyra) by maintaining the relative weight ratios of the hemp and palmyra as 1:1, 2:1, and 1:2. shows the preparation of fibers in three modes of stacking sequence. Epoxy and hardener were blended with weight ratio of 10:1, respectively. Each layer was pre-impregnated with matrix and placed one layer over the other layer in the mold until it achieved the thickness of 4 mm. The bubbles trapped between the layers were removed by moving the steel roller over the composite. Finally, the composite laminate was compressed by placing the mold release sheet and allowed to cure at room temperature for 48 h. The required specimens were prepared as per dimensions given in the ASTM standards to investigate the physical, mechanical, and thermal behavior. shows the fabricated composite laminate and specimens as per ASTM standards.
Table 1. Detailed designation and NaOH concentration of fabricated composites.
Mechanical behavior of hybrid composites
Tensile test was conducted under the uniaxial loading conditions of the fabricated specimens. Tensile properties were evaluated with a cross head speed of 5 mm/min using ASTM D3039–76 standards. The test was performed using tensometer with specimen gage length of 50 mm. The flexural properties of the prepared composite specimens were evaluated using three-point bending test on the same tensometer. Flexural strength was determined as per ASTM D790 standard with a speed of 5 mm/min. Impact energy of the fabricated specimens was evaluated by low velocity Izod impact tester supplied by VEEKAY test lab, India, as per ASTM D 256 standard. The specimen dimension for the impact test was 64 mm × 12.7 mm × 4 mm. Leitz microhardness tester was used to evaluate the Vickers hardness of the specimens as per ASTM D785 test standard.
Physical behavior of hybrid composites
The experimental densities of composite specimens were measured by Archimedes method as per ASTM D 792–91 standard. Knowing the mass and volume of the samples, the density is calculated using the Eq. (1).
Thermal conductivity of hybrid composites
The thermal conductivity of samples was measured using the slab method as per the process mentioned in ASTM E1530–99 standards. The dimensions of the composite specimen were 50 mm in diameter and 4 mm in thickness. To find the thermal conductivity, UnithermTM model 2022 is used. The composite samples were located between cold plate and hot plate. Based on the temperature difference and heat flux, the thermal conductivity was measured as shown in Eq. (3).
Thermogravimetric analysis
The temperature of the phase transformation and the decomposition behavior of the hemp and palmyra fiber-reinforced hybrid composites are done by Simultaneous Thermal Analyzer, STA7200, Hitachi HTG equipment. Samples weighing approximately 5 mg are subjected to pyrolysis in oxygen environment. The temperature range is taken from 30 to 600°C at a heating rate of 3.5°C/min. The mass loss is recorded in response to increasing temperature.
Scanning electron microscopy (SEM)
The cross-section and fiber surface of untreated and treated fibers were taken using a scanning electron microscope model JEOL JSM-6480LV. Before conducting the SEM evaluation, the specimens were placed on aluminum stubs coated with gold by means of a plasma sputtering apparatus.
Results and discussion
Density and water absorption
The experimental density of the untreated and NaOH-treated hemp and palmyra fiber-reinforced composites is shown in . For NaOH-treated composites, there was some slight increase in the density values of the hybrid composites as the NaOH concentration was increased. The alkali treatment eliminates the impurities, which contribute to decrease the fiber volume; hence, the density of treated fiber composites increases as compare to untreated fiber composites. On the other hand, the density values of the composites did not appear to be affected by the stacking sequence of the fibers. represents the effect of stacking sequence and NaOH concentration on the water absorption properties of fabricated composites. In all the stacking sequences, the water absorption of the NaOH-treated hemp and palmyra fiber-reinforced hybrid composites was lower than that of the untreated fiber-reinforced composites. This may due to strong intermolecular reinforcement and matrix bonding decreased the rate of water absorption in hybrid composites. The composites with stacking sequence PPHHPP have a slight positive tendency was observed compared with the other two stacking sequences.
Tensile properties
depict the influence of alkali treatment and stacking sequence on tensile strength and tensile modulus of fabricated composite specimens. Significant difference was found in the results between the alkali-treated and untreated composites. Improvement in the tensile properties of alkaline-treated hybrid composites is observed due to the removal of impurities such as fats, pectin, and lignin along the fiber surface, which improvises the fiber bonding with matrix. Up to 6% NaOH concentration, the tensile characteristic of the composite specimens increases and then decreases at 8% NaOH concentration. At 6% NaOH concentration, the maximum tensile strength is found 81.83 MPa, 76.48 MPa, and 70.83 MPa with stacking sequence of PPHHPP, HPHPHP, and HHPPHH, respectively. Compared with the untreated composites, the alkali-treated composites at 6% NaOH concentration improve the tensile strength up to 19.34%, 14.5%, and 16.03% with stacking sequence of PPHHPP, HPHPHP, and HHPPHH, respectively. At 6% NaOH concentration, the maximum tensile modulus is found to be 4.965 GPa, 4.734 GPa, and 4.507 GPa with stacking sequence of PPHHPP, HPHPHP, and HHPPHH, respectively. In the earlier research work reported on the effect of surface treatment of fiber on mechanical characteristics of composites, coir (Rout et al. Citation2001) jute (Saha et al. Citation2010), sisal (Towo and Ansell Citation2008), Agave (Mylsamy and Rajendran Citation2011), and oil palm (Shinoj et al. Citation2011) fibers with 6% sodium hydroxide concentration increased the tensile properties of composites.
Flexural properties
The influence of alkali treatment and stacking sequence on flexural strength and flexural modulus of the fabricated composites are shown in . The figure displays the average flexural strength related with maximum and minimum values of each specimen. The 6% NaOH-treated composites showing maximum flexural strength values of about 116.83 MPa, 101.48 MPa, and 128.91 MPa with stacking sequence of HPHPHP, HHPPHH, and PPHHPP, respectively. The percentage improvement from untreated to treated composites with 6% NaOH concentration in HPHPHP, HHPPHH, and PPHHPP layering hybrid composites are found 14.73%, 9.45%, and 16.77%, respectively. The 6% NaOH-treated composites showing maximum flexural modulus values about 7.426 GPa, 6.781 GPa, and 7.996 GPa with stacking sequence of HPHPHP, HHPPHH, and PPHHPP, respectively. This could be due the alkali treatment improves the bonding between reinforcement and epoxy matrix and permitting the matrix to enter into reinforcement in the fabrication, which leads to the high load carrying by the reinforcement during testing conditions. The decrease in flexural strength at 8% NaOH concentration may be due to the higher NaOH concentration that may damage the fiber cellulose which will reduce the strength. On other hand, lower flexural strength was observed when the hemp fiber was used as outer layer and palmyra fiber as the inner layer. This may be due to the lower intrinsic properties of hemp fiber as related to palmyra fiber (Narayana, Rao, and Rao Devireddy Citation2020).
Impact energy
It is known that the impact strength of composite material is depending on the interfacial bonding, fiber pullout, fiber, and matrix properties. illustrates the impact energy values of tested composites in graphic form. The alkali treated hybrid composite specimens was shown better values as compared to untreated composites. Up to 6% NaOH concentration, the impact of energy values of fabricated hybrid composites are progressively increased to the maximum value and then decrease at 8% NaOH concentration. And the similar trend also occurred for all the stacking sequences. From the figure, it is detected that the impact energy of HPHPHP, HHPPHH, and PPHHPP composites is high about 12.78%, 13.06%, and 11.07%, respectively, as compared to untreated composites. A similar trend of observations was found with oil palm fiber by Obasi et al. (Obasi et al. Citation2014), in which after alkali treatment, NaOH was effectually placed on the oil palm fiber surface and there was an increase in the impact strength by 18.21%.
Hardness
The Vickers hardness of untreated and NaOH-treated composites with different stacking sequence is present in . Similar to the tensile and flexural properties, the NaOH treatment increases the hardness of composite as compared to untreated composites. The hybrid composites fabricated with 6% NaOH treated to hemp and palmyra fiber contribute to the better performance of compared to the untreated and other NaOH treatments. At 6% NaOH concentration, the maximum hardness values for the composites with stacking sequence HPHPHP, HHPPHH, and PPHHPP are 45.1 Hv, 42.6 Hv, and 42.6 Hv, respectively.
Thermal conductivity
The influence of stacking sequence and NaOH treatment on thermal conductivity of hemp and palmyra composites is shown in . Due to the lower thermal conductivity values of both the hemp and palmyra fibers, the overall thermal resistance offered by the composite increases and accordingly the thermal conductivity of the hybrid composite material decreases. It is evident from the figure that with the addition of hemp and palmyra fibers, irrespective of the stacking sequence, there is always a reduction in the heat conduction capability of the hybrid composites. However, it is found that this reduction is more in case of composites with stacking sequence HHPPHH. From the Figure, it is found that the thermal conductivity value composites with 8% NaOH concentration show values 0.186 W/m-K, 0.175 W/m-K, and 0.198 W/m-K with stacking sequence of HPHPHP, HHPPHH, and PPHHPP, respectively. The thermal conductivity of the composites with stacking sequence HPHPHP, HHPPHH, and PPHHPP is decreased by 31.40%, 32.23%, and 29.75%, respectively.
Thermogravimetric analysis
The thermal degradation analysis of fabricated composite specimens was carried out for 6% NaOH treated and 30 wt.% of fiber loading with three different stacking sequences HPHPHP, HHPPHH, and PPHHPP. shows the thermogravimetric analysis curves for the hybrid composites at 30 wt.% fiber loading with different stacking sequences. All the hybrid composites degradation occurred in two stages: a first stage with a slight weight loss in the range of 30–250°C due to the release of moisture content present in the natural fiber component of these composites and a second stage, which presents between 250 and 430°C due to the pyrolysis process. This second stage is also where the lignin and hemicellulose degradation happened (250–430°C), while another loss of weight at 430–500°C is related to the degradation of the cellulose component. The thermal degradation properties of hybrid natural fiber-reinforced composites are influenced by the contributions of the individual thermal degradation properties of both the fibers and matrix. It is observed that the thermal stability of the hybrid composite specimen with HHPPHH stacking sequence is better when compared to HPHPHP and PPHHPP stacking sequence.
Scanning electron microscopy (SEM)
To study the effect of NaOH concentration on hemp and palmyra fiber, surface morphology was carried out on the untreated and treated fibers and their hybrid composites using scanning electron microscopy. The surface morphology photographs of both untreated and alkali-treated hemp fiber surfaces are exhibited in . As expected, the surface morphology of treated fibers was different to those untreated fibers in terms of their level of smoothness and roughness. Clearly from , the impurities were found on the surface of the untreated hemp fiber. On the other hand, displays hemp fiber after 6% NaOH treatment, which was soaked for 5 h. The impurities have been removed from fiber surface and also show some scrapes along the surface.
Figure 15. Surface morphology of (a) untreated hemp fiber and (b) treated hemp fiber with 6% NaOH solution (c) untreated palmyra fiber and (d) treated palmyra fiber with 6% NaOH solution.

shows SEM spectrographs of the tensile fracture surfaces of the hemp and palmyra hybrid composites fabricated with untreated and NaOH-treated composites. From the , it is clearly observed that the hemp and palmyra fibers were pull-out easily from the epoxy matrix due to the interfacial failure between reinforcement and matrix. However, for NaOH-treated composites as shown in , maximum fibers were adhered to the epoxy matrix and the length of fiber pull-out was very shorter than untreated composites. These results support that the NaOH treatment of hemp and palmyra fibers show good interfacial bonding with epoxy resin. The key reason for good interfacial bonding between treated fibers and epoxy resin is that the increase of hydroxyl groups over the fiber surface by removal of the impurities during the NaOH treatment. Fiber pull-out, fiber breakage, and air set-ups are detected in figure, which are a consequence of poor adhesive bonding between the reinforcement and matrix.
Conclusions
The influence of alkali treatment and stacking sequence on physical, mechanical, and thermal behavior of hybrid composites has been studied and the following conclusions were drawn:
Compared with the untreated composites, the 6% NaOH-treated hybrid composites increased the flexural strength (+16.77%), tensile strength (+19.34%), impact energy (+13.06%), and hardness (+12.35%) of hybrid composites. SEM investigation revealed that load carrying capacity by the fiber and interfacial bonding improved after NaOH treatment which attributed to the increment in mechanical behavior of hybrid composite.
Compared with the untreated composites, composite with HHPPHH stacking sequence decreases thermal conductivity by 32.23%.
The investigation exhibited that 8% NaOH concentration would damage the fiber strength, which plays a main role as far as the mechanical properties was decreased.
Highlights
Preparation of hybrid composite materials reinforced with hemp and palmyra fibers.
A comprehensive discussion on effect of alkali treatment on physical, mechanical, and thermal properties of hemp and palmyra fiber-reinforced composites.
Improvement of mechanical and thermal properties of the elaborated materials.
Disclosure statement
No potential conflict of interest was reported by the authors.
References
- Bachtiar, D., S. M. Sapuan, and M. M. Hamdan. 2009. The influence of alkaline surface fibre treatment on the impact properties of sugar palm fibre-reinforced epoxy composites. Polymer-Plastics Technology and Engineering 48 (4):379–16. doi:10.1080/03602550902725373.
- Bachtiar, D., S. M. Sapuan, and M. M. Hamdan. 2010. Flexural properties of alkaline treated sugar palm fibre reinforced epoxy composites. International Journal of Automotive and Mechanical Engineering 1 (1):79–90. doi:10.15282/ijame.1.2010.7.0007.
- Chandrasekar, M., M. R. Ishak, S. M. Sapuan, Z. Leman, and M. Jawaid. 2017. A review on the characterisation of natural fibres and their composites after alkali treatment and water absorption. Plastics, Rubber & Composites 46 (3):119–36. doi:10.1080/14658011.2017.1298550.
- Christian, S. J., and S. L. Billington. 2011. Mechanical response of PHB-and cellulose acetate natural fiber-reinforced composites for construction applications. Composites Part B: Engineering 42 (7):1920–28. doi:10.1016/j.compositesb.2011.05.039.
- Devireddy, S. B. R., and S. Biswas. 2017. Physical and mechanical behavior of unidirectional banana/jute fiber reinforced epoxy based hybrid composites. Polymer Composites 38 (7):1396–403. doi:10.1002/pc.23706.
- Haameem, M. J. A., M. S. Abdul Majid, M. Haslan, M. Afendi, E. A. Helmi, and F. Idris. 2014. Effects of alkaline treatments on the tensile strength of Napier grass fibres. Applied Mechanics & Materials 695:340. doi:10.4028/0000www.scientific.net/AMM.695.340.
- Hamidon, M. H., M. T. Sultan, A. H. Ariffin, and A. U. Shah. 2019. Effects of fibre treatment on mechanical properties of kenaf fibre reinforced composites: A review. Journal of Materials Research and Technology 8 (3):3327–37. doi:10.1016/j.jmrt.2019.04.012.
- Harry, K., H. Wang, N. Pattarachaiyakoop, and M. Trada. 2011. A review on the tensile properties of natural fiber reinforced polymer composites. Composites Part B: Engineering 42 (4):856–73. doi:10.1016/j.compositesb.2011.01.010.
- Huang, G. 2009. Tensile behaviours of the coir fibre and related composites after NaOH treatment. Materials & Design 30 (9):3931–34. doi:10.1016/j.matdes.2009.01.035.
- Islam, M. S., K. L. Pickering, and N. J. Foreman. 2010. Influence of alkali treatment on the interfacial and physico-mechanical properties of industrial hemp fibre reinforced polylactic acid composites. Composites Part A, Applied Science and Manufacturing 41 (5):596–603. doi:10.1016/j.compositesa.2010.01.006.
- Mylsamy, K., and I. Rajendran. 2011. The mechanical properties, deformation and thermomechanical properties of alkali treated and untreated Agave continuous fibre reinforced epoxy composites. Materials & Design 32 (5):3076–84. doi:10.1016/j.matdes.2010.12.051.
- Narayana, V. L., L. B. Rao, and S. B. Rao Devireddy. 2020. Effect of fiber percentage and stacking sequence on mechanical performance of unidirectional hemp and palmyra reinforced hybrid composites. Revue des Composites et des Matériaux Avancés 30:30. doi:10.18280/rcma.303-405.
- Obasi, H. C., N. C. Iheaturu, F. N. Onuoha, C. O. Chike-Onyegbula, M. N. Akanbi, and V. O. Ezeh. 2014. Influence of alkali treatment and fibre content on the properties of oil palm press fibre reinforced epoxy biocomposites. American Journal of Engineering Research 3 (2):117–23.
- Owen, M. M. 2014. The effects of alkali treatment on the mechanical properties of jute fabric reinforced epoxy composites. International Journal of Fiber and Textile Research 4 (2):32–40.
- Pickering, K. L., M. G. Aruan Efendy, and L. Tan Minh. 2016. A review of recent developments in natural fibre composites and their mechanical performance. Composites Part A, Applied Science and Manufacturing 83:98–112. doi:10.1016/j.compositesa.2015.08.038.
- Reddy, R. A., K. Yoganandam, and V. Mohanavel. 2020. Effect of chemical treatment on natural fiber for use in fiber reinforced composites–Review. Materials Today: Proceedings 33:2996–99. doi:10.1016/j.matpr.2020.02.982.
- Rout, J., M. Misra, S. S. Tripathy, S. K. Nayak, and A. K. Mohanty. 2001. The influence of fibre treatment on the performance of coir-polyester composites. Composites Science and Technology 61 (9):1303–10. doi:10.1016/S0266-3538(01)00021-5.
- Saha, P., S. Manna, S. R. Chowdhury, R. Sen, D. Roy, and B. Adhikari. 2010. Enhancement of tensile strength of lignocellulosic jute fibers by alkali-steam treatment. Bioresource Technology 101 (9):3182–87. doi:10.1016/j.biortech.2009.12.010.
- Sawpan, M. A., K. L. Pickering, and A. Fernyhough. 2011. Improvement of mechanical performance of industrial hemp fibre reinforced polylactide biocomposites. Composites Part A, Applied Science and Manufacturing 42 (3):310–19. doi:10.1016/j.compositesa.2010.12.004.
- Shinoj, S., R. Visvanathan, S. Panigrahi, and M. Kochubabu. 2011. Oil palm fiber (OPF) and its composites: A review. Industrial Crops and Products 33 (1):7–22. doi:10.1016/j.indcrop.2010.09.009.
- Suardana, N. P. G., Y. Piao, and J. Kyoo Lim. 2011. Mechanical properties of hemp fibers and hemp/pp composites: Effects of chemical surface treatment. Materials Physics and Mechanics 11 (1):1–8.
- Tingju, L., M. Jiang, Z. Jiang, D. Hui, Z. Wang, and Z. Zhou. 2013. Effect of surface modification of bamboo cellulose fibers on mechanical properties of cellulose/epoxy composites. Composites Part B: Engineering 51:28–34. doi:10.1016/j.compositesb.2013.02.031.
- Towo, A. N., and M. P. Ansell. 2008. Fatigue evaluation and dynamic mechanical thermal analysis of sisal fibre–thermosetting resin composites. Composites Science and Technology 68 (3–4):925–32. doi:10.1016/j.compscitech.2007.08.022.
- Venkateshwaran, N., A. Elaya Perumal, and D. Arunsundaranayagam. 2013. Fiber surface treatment and its effect on mechanical and visco-elastic behaviour of banana/epoxy composite. Materials & Design 47:151–59. doi:10.1016/j.matdes.2012.12.001.
- Xue, L., L. G. Tabil, and S. Panigrahi. 2007. Chemical treatments of natural fiber for use in natural fiber-reinforced composites: A review. Journal of Polymers and the Environment 15 (1):25–33. doi:10.1007/s10924-006-0042-3.
- Yan, L., N. Chouw, L. Huang, and B. Kasal. 2016. Effect of alkali treatment on microstructure and mechanical properties of coir fibres, coir fibre reinforced-polymer composites and reinforced-cementitious composites. Construction and Building Materials 112:168–82. doi:10.1016/j.conbuildmat.2016.02.182.
- Yousif, B. F., A. Shalwan, C. W. Chin, and K. C. Ming. 2012. Flexural properties of treated and untreated kenaf/epoxy composites. Materials & Design 40:378–85. doi:10.1016/j.matdes.2012.04.017.