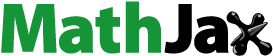
ABSTRACT
Conventional dyeing of cellulose with reactive dyes requires the use of huge quantities of electrolytes and alkalis and the temperature between 60–90°C. Using the above conditions a stable covalent bond is formed between the reactive group of the dye and the hydroxyl group of cellulose. Cationization of cellulose allows to carry out reactive dyeing without electrolytes and alkalis even at room temperature. The dye, depending on the cationic modifier used, can form only ionic bond between sulfonic group of the dye and quaternary group of the modifier or also covalent bond between reactive group of the dye and hydroxyl group of the modifier. In this work we investigate how light fastness is affected by the type of bond with which the dye bound to the cationized cellulose. So far, such research has not been published. We found that the light fastness of the reactive dyeings on cationized cellulose was lower compared to non-cationized cellulose and depends on the kind of modifier used: chlorocholine chloride, 3-chloro-2-hydroxy-propyltrimethylammonium chloride and copolymer [(chloro-methyl)oxirane +1 H-Imidazole]. Density electron changes of ionic bonds between quaternary group of modified cellulose and sulfonic group of reactive dyes were analyzed in relation to the fading process.
摘要
用活性染料对纤维素进行常规染色需要使用大量的电解质和碱,温度在60-90摄氏度之间. 使用上述条件,在染料的反应基团和纤维素的羟基之间形成稳定的共价键. 即使在室温下,纤维素的阳离子化也可以在没有电解质和碱的情况下进行活性染色. 根据所使用的阳离子改性剂,染料只能在染料的磺酸基和改性剂的四元基团之间形成离子键,也可以在染料的反应性基团和改性器的羟基之间形成共价键. 在这项工作中,我们研究了染料与阳离子纤维素结合的键类型如何影响耐光性. 在这项工作中,我们研究了染料与阳离子纤维素结合的键类型如何影响耐光性. 到目前为止,此类研究尚未发表. 我们发现,与非阳离子纤维素相比,阳离子纤维素上的活性染料的耐光性较低,3-氯-2-羟基丙基三甲基氯化铵和共聚物[(氯甲基)环氧乙烷+1 H-咪唑]分析了改性纤维素季铵基和活性染料磺酸基之间离子键的密度电子变化与褪色过程的关系.
Introduction
The light fastness of dyed textiles is one of the most important features of finished products. Light fastness refers to the resistance of the dyed material to changes in its color during exposition to sunlight or artificial light and is in a special case of the broader concept of color fastness (Nelson Citation1995). The phenomenon by which dyes undergo fading is associated with photo degradation as a complex process (Latif et al. Citation2015). Many factors influence the fading of colored textiles such as the bond between the dye and the fiber, the chemical structure of the dye and substrate, radiation quality as well as temperature and humidity. The concentration of dye on the fiber and the chemical modification of the fiber are also important factors. Permanent bonding with the fiber ensures the coplanarity of the dye molecules. In the case of cellulose fibers, the large size, linear shape and the presence of electron donating groups in the dye molecules allow to create additional hydrogen bonds with cellulose (Tera et al. Citation2016). Oakes (Citation2001) showed that 40% of fading is caused by UV rays, another 25% due to heat and 25% being caused by normal visible light. The remaining 10% of fading results from indoor artificial lighting, humidity, and poor dye anchorage. Both visible light and UV radiation cause fading, where visible light leads to photoxidation (Genc and Can-Dogan Citation2006), while UV radiation triggers the degradation process of molecules (Brenton, Morgan, and Beynon Citation1979). In both cases, this reaction requires energy in the form of light as well as oxygen in the form of singlet (Latif et al. Citation2015).
It was observed that reactive dye light fastness mainly depends on the chemical structure of chromophore (Thiagarajan and Nalankill Citation2010, Citation2014). The azo chromophore has lower light fastness, while metal complex and anthraquinone chromophore dyes has good light fastness. Sulfonic group in reactive dye does not have a noticeable impact on light fastness while the presence of – OH, –NH2, and – NHR groups in the azo dye molecule decrease light stability of the dyes.
Reactive dyes are becoming increasingly popular for dyeing cellulosic fibers because of their wide shade range, ease of application, and excellent wet fastness properties. Reactive dyes are mainly used in the long-liquor dyeing processes (exhaustion dyeing) (Lewis Citation2014). The first interaction between fibers and dyes during the dyeing process is dye exhaustion onto the fiber. However, in an aqueous bath, cellulosic fibers will develop a negative charge on their surface. Also, the sulfo group of the reactive dyes dissociate to a negative ion (‒SO3‒) in water. Thus, the negatively charged dyes and negatively charged fiber undergo ion repulsion, causing the exhaustion to be more difficult. In order to overcome this problem, electrolytes (the most common one is sodium sulfate) are added to assist the exhaustion process. Following the exhaustion, fixation step is carried out by adding alkali. Covalent bond formation between the dye and the fiber can only occur when the dye has been absorbed into the cellulose phase.
Despite of the many advantages of this group of dyes, their application poses a high risk to the environment. Electrolytes environmental pollution is estimated at around 200,000–250,000 tons per year (Aktek and Malekul Millat Citation2017).
Introducing of the cationic groups to the chain of cellulose in the modification process completely changes the cotton surface’s charge from negative to partially or totally positive. That allows the electrostatic attraction, simultaneous exhaustion, and fixation of reactive dyes on cellulose without presence of electrolytes and alkalis.
The most popular as modification agent for cellulose is still CHPTAC, which in cationization process react with hydroxyl group of cellulose on etherification way (Farrell Citation2012; Hashem et al. Citation2010). In the past decades, nearly a thousand publications (Correia et al. Citation2020) about the cationization of cotton fibers and dyeing, printing and finishing of cationized cotton were published.
Many of these works contain results of dyeing fastness to washing, rubbing and light in comparison to conventional methods. Giles (Citation1957) showed, based on the statistical study, that the light fastness is often reduced by cationic surface-active agents or hydrogen-bonding compounds, e.g. phenols or urea, used as dyeing assistants or after treating agents. Also, the smaller particles of dye have tendency to fade more rapidly than larger ones, and therefore their fading rate determines the fastness grade of the dyed material, which is judged in the earlier stages of fading. Chattopadhyay (Citation2001) showed that the poor light fastness on PAE/EDA treated cotton was attributed to the presence of dye mainly on the surface of the fiber. This phenomenon is commonly called as ring dyeing. Kannan et al. (Citation2006) reported that the light fastness rating is slightly reduced for some dyes, about half to one point, as was presented by various researches previously. The presence of an aliphatic chain between the dye and fiber may be disturbing the stable electronic configuration of dye that leads to the shifting of electrons disintegration of dye by the photons of light rays. Ghazal, El-Masry, and Mosaad (Citation2011) reported that the better light fastness of Acid Blue 25 and Acid Yellow 36 on CHPTAC cationized fabrics results from higher concentration of the dyes on cationized samples (dark shade) compared to pale shade of uncationized samples. Ilango (Citation2015) reported that the light fastness of dyeings with some reactive dyes on of the CHPTAC cationized fabric samples were improved when the dyeing process was performed comparably to conventional method in the absence salt only. Chatha et al. (Citation2016) observed that all the dyed cotton fabric samples untreated and treated with different concentrations of chitosan have equally good light fastness properties. Aktek and Malekul Millat (Citation2017) reported that cationization of cellulose with NMA-HTCC owning quaternary ammonium groups gave lower light fastness due to it molecular size compared to uncationised cellulose. The NMA-HTCC due to large molecule does not penetrate into the fiber structure and remains mainly on surface of cellulosic fibers. However, careful selection of reactive dyes can reduce above problem to some extent. Abedin et al. (Citation2021) reported that the light fastness of the reactive dyes on CHPTAC cationised cotton were improved to 4 from 3 compared to conventional dyeing resulted from better dye fixation and the lack of dye degradation by the light photons. It can be noticed that cationized cotton was dyed with soda. Correia at coworkers (Correia et al. Citation2021) examinated the light fastness of dyeings with Reactive Red 195 on cationized cellulose with two cationic agents i.e. CHPTAC and Polyquatermium2 (P42). The cationized samples were additionally treated with plasma before and after cationization process. Fabrics treated with CHPTAC have satisfactory level of light fastness (5/4–5) (both on fabric with and without plasma treatment) in comparison to conventional dyeings. In case of P42 the level of light fastness (2/1)was unsatisfactory.
It is obvious that the permanent modification of cellulose with the use of different modifying agents leads to the production of various new cellulose polymers with altered properties compared to unmodified cellulose. This is especially true of the cationization process in which, depending on the modifier used, the new cellulose polymer obtains a partial or complete positive charge on its surface.
It was shown in our previous researches (Pruś et al. Citation2022a, Citation2022b) that cellulose cationized with 3-chloro-2-hydroxy-propyltrimethylammonium chloride or copolymer [(chloro-methyl)oxirane +1 H-Imidazole] can be dyed with reactive dyes without electrolytes and alkalis at room temperature. Reactive dyes during the dyeing process in above conditions form covalent bonds with the hydroxyl group of the cationic modifier in adjacent position to the quaternary group instead of with the hydroxyl group of the glucopyranose ring. The mechanism of this reaction was proposed and confirmed by extraction with boiling DMF and by analysis electron density calculation. The dyes covalently bonded with the fiber were not extractable. The dyeing cellulose cationized with chlorocholine chloride (no hydroxyl group in modificator chain) showed that in the same conditions only electrostatic strong ionic pair was formed. The formation of an ionic bond between the sulfonic group of the dye and the quaternary group of the modifier is confirmed by its sensitivity to extraction in DMF.
The main aim of this study was to find the influence on light fastness of these bonds in comparison to the covalent bond formed between reactive dye and unmodified cellulose after dyeing in conventional process. Thus, unmodified and modified cellulose dyed with selected reactive dyes was used for examined their light fastness.
Materials and methods
The unmodified and cationized cotton samples dyed with selected reactive dyes used for irradiation experiments in this work were prepared according to the our earlier research works receipies (Pruś et al. Citation2022a, Citation2022b). According to the above, a 100% bleached cotton fabric with a smooth wave and a declared basis weight of 180 g/m2, was modified with three different cationic modifiers (A, B and C - ). Dyeing process with selected reactive dyes () of unmodified cellulose samples was performed using conventional methods in accordance with the recipes recommended by the manufacturers of these dyes (Pruś et al. Citation2022a). Cationized cellulose samples were dyed under ecological conditions (without electrolytes, alkalis and at room temperature), using 1% owf (RR 221 or RB 160) and 0.9% owf (RR 24:1 or RR 274 or RB 19) respectively to obtain a similar color strength. The samples prepared in this way were used for experimental tests to test the color fastness to light by irradiation under the same conditions.
The light fastness of dyed samples was studied after exposing to artificial light for 1, 2, 6, 12, 24 and 36 hours (according to the PN-EN ISO 105-B02: 2014–11 Method 2). The samples were irradiated in Q-SUN Xenon Test Chamber (Q-Lab Corporation, model XE-2). Change of color of covered part and exposed part of dyed sample was estimated by K/S on spectrophotometer Datacolor 400 (Datacolor, USA). Measurement was done using: measuring geometry d/8, measuring window 9 mm, DSLR disabled, no UV filter, D65 illuminant, and 10° observer.
Relative color change of the analyzed samples after exposition to light was calculated according to EquationEquation 1[1]
[1] :
where:
- relative color change after irradiation,
- color values for started sample,
- color values for samples after irradiation for 1, 2, 6, 12, 24 and 36 hours respectively.
Results and discussion
The linkage of reactive dyes with unmodified and cationized cellulose samples according to our earlier works (Pruś et al. Citation2022a, Citation2022b) is shown in : All analyzed samples lose some of their color strength and nuance of shade progressively to the time of exposition to irradiation. The changes after 1, 2, 6, 12, 24 and 36 hours irradiating measured as K/S at λmax and expressed as ∆Rcf were calculated accordingly to the EquationEq. [1][1]
[1] and presented in as final results (∆Rcf after 36 hours irradiation) and as color changes (during the time of irradiation) respectively.
Figure 3. Chemical bonds between selected reactive dyes with cellulose uncationised (I) and cationised with: chlorocholine chloride (II), CHPTAC (III) and Texamin ECE New (IV) respectively.

Table 1. Comparison of the light fastness for reactive dyes on cellulose unmodified in relation to cationised with selected modifiers (1) Light fastness after 36 hours irradaiation according to the blue scale).
Taking under consideration that all dyed samples were irradiated in the same conditions it was possible to indicate the following general statements:
(1) Most of the reactive dyes used for dyeing unmodified cellulose in conventional method have better light fastness properties than on cationised ones. Apart of permanent covalent linkage between the dye and the hydroxyl group of the cellulose [] they have a possibility of creation a lot of hydrogen, van der Waals, dipoles and π bonds as well as intra- and intermolecular linkages. The light fastness of selected reactive dyes on unmodified cellulose according to the ∆Rcf index after 36 hours irradiation can be ordered as follow:
RB 160 (19.41)> RR 274 (24.40)> RB 19 (28.60)> RR 221 (29.85)> RR 24:1 (41.90)
(2) ∆Rcf index after 36 hours irradiation for dyes bonded with cationised cellulose only as ionic pair between the sulfonic group of the dye and quaternary group of the modifier () indicate for all dyes lower values. Those types of bonds are typical for acid dyes and can be ordered as follow:
RB 160 (30.80)> RR 274 (39.30)> RR 24:1 (50.65)> RB 19 (52.81)> RR 221 (55.86)
(3) ∆Rcf index after 36 hours irradiation for dyes bonded with cationised cellulose as ionic pair in the same way as the dyes in point 2. and covalent bonded according to the nucleophile substitution or addition mechanism like dyes with uncationised cellulose but with hydroxyl group belonging to the moderator chain instead to the hydroxyl group of glucopyranose ring. ∆Rcf index for selected dyes can be ordered as follow:
a) for cationised cellulose with [CHPTA]+Cl−
RB 160 (23.86)> RR 24:1 (30.9))> RR 221 (37.7)> RR 274 (40.6)> RB 19 (48.64)
b) for cationised cellulose with [IME]+Cl−
RB 160 (45.74)> RR 274 (54.67)> RB 19 (55.42)> RR 24:1 (67.50)> RR 221 (75.54)
The results of the light fastness of samples dyed in ecological conditions on cationic modified cellulose indicate their decreasing relation to dyeings on unmodified cellulose, regardless of the type of modifier and reactive dye used. After 36 hours of irradiation the lowest loss of color was for dyeings on unmodified cellulose and the highest for dyeings of cotton samples cationised with [IME]+Cl−.
Table 2. Electron densities data for quaternary groups and sulfonic group.
(4) Modification of cellulose fibers by cationisation creates many positive charge sites, mainly on their surface what allow to form of the ionic bonds with sulfonic group of reactive dye[ (II)]. At the second step covalent bond between reactive dye and hydroxyl group, belonging to the modificator chain of cationised cellulose was formed [ (III and IV)]. Cationization process leads to decreasing of amount of hydroxyl groups in cellulose capable to create bonds what reduced the ability to establish hydrogen bond between polymer and dye molecules. Lower amount of hydrogen linkages, causing weaker protection of the dye chromophore by cellulose macromolecules, results in a decrease in light fastness. Additionally, cationized cellulose, thanks to the introduction of quaternary groups containing nitrogen atoms becomes like to proteinaceous fibers. The dyeings on this group of fibers tend to fade according to the photo-reduction mechanism (Oakes Citation2001) what need for excitation more high-energy states. Photoreductive processes also generally involve radicals but high-energy light (UV or near UV) is required to facilitate their formation via bond cleavage. On the other hand, the photooxidative route becomes progressively more important when the dye is subject to lower-energy visible excitation in the presence of oxygen, particularly with cellulosic substrates. Visible radiation quanta carry energy capable of breaking the weakest chemical bonds (∼160-300 kJ/mol), while UV radiation energy also breaks permanent bonds.
(5) Presence of positive charges on cationized cellulose causes reduction of electron density on the surface of dyeings what decrease the barrier before UV light attack. Most of the active species responsible for degradation of chromophores of the dyes have total or partial negative charge. presents electron densities for quaternary group of analyzed modificators in cationised cellulose compared with sulfo group in reactive dye (Pruś et al. Citation2022a, Citation2022b).
Results presented in show that the most negative charge has the surface on unmodified cellulose. Modification cellulose with CHPTAC or chlorocholine chloride gives very similar changing in electron density per one quaternary group. The introduction of one quaternary group of [IME]+Cl− copolymer to cellulose gives the most positive values compared to [ClCh]+Cl− and [CHPTA]+Cl−. These values correspond to obtained results of light fastness after irradiation of unmodified and cationised samples cellulose materials.
Conclusion
The research confirmed that cellulose modified by cationization with quaternary modifiers dyed with reactive dyes had lower light fastness parameters in comparison to unmodified ones. Probably this is one of the reasons, that this method of modification is not introduced to the industrial scale, although thanks to that modifiacion is possible to eliminate a huge quantity of electrolytes and alkalis may be released from dyeing houses to environment. This research presents the problems with light fastness dyed cationised cotton samples related to chemical bonds formed during dyeing in ecological conditions (without electrolytes and alkalis in room temperature).
It seems also that, the greater impact for improving fading process is involved with decreasing of electron densities on the surface cationised cellulose materials. That hypothesis confirmed electron density analysis.
Abbreviations
Cell-OH | = | cellulose |
[ClCh]+Cl− | = | chlorocholine chloride |
[CHPTA]+Cl− | = | 3-chloro-2-hydroxypropyltrimethylammonium chloride (CHPTAC) |
[Cell-O-IME]+Cl− | = | cellulose cationised with copolymer (chloromethyl)oxirane-1 H-Imidazole |
DMF | = | dimethylformamide |
Glu | = | glucopiranose ring |
[IME]+Cl− | = | copolymer (chloromethyl)oxirane-1 H-Imidazole (Texamin ECE New) |
NMA-HTCC | = | O-acrylamidomethyl-N-[2-hydroxy-3-trimethylammonium)propyl]chitosan chloride |
owf | = | on weight fiber |
PAE/EDA | = | polyamide epichlorhydrin/ethylenediamine polymer |
PhSO3H | = | benzenesulfonic acid |
Polyquatermium2 (P42) | = | poly[bis(2-chloroethyl)ether-alt-1,3-bis[3-dimethylamino)propyl]urea quaternized |
RB 19 | = | Reactive Blue 19 |
RB 160 | = | Reactive Blue 160 |
RR 24:1 | = | Reactive Red 24:1 |
RR 221 | = | Reactive Red 221 |
RR 274 | = | Reactive Red 274 |
= | relative color change after irradiation |
Higihlights
Reactive dyes when dyeing cationised cellulose strongly absorb the fibers which leads to elimination of the electrolytes normally used in conventional methods.
Our innovative work has also shown the possibility of eliminating alkalis from the dyeing process and obtaining permanent and efficient dyeing with these dyes even at ambient temperature. It was found that under these completely ecological conditions, covalent bonds are formed between the modified cellulose and the reactive dye, which are different than in the dyeing of unmodified cellulose. In this work, tests were performed to confirm the hypothesis that the dyeings obtained in ecological dyeing with reactive dyes on samples of cationised cellulose with three different modifiers may show significant differences in their light fastness.
Irradiation of the stained samples confirmed our hypothesis. The relative change in the color strength of the irradiated samples over time and after 36 hours showed a greater or lesser change. The tests were carried out in comparison to dyeings obtained according to the conventional method.
Further work taking into account the possibilities of other modifiers and dyes with full elimination of electrolytes and alkalis and dyeing in ambient temperature may lead not only to environmental protection, but also to better resistance indicators.
Disclosure statement
No potential conflict of interest was reported by the authors.
Additional information
Funding
References
- Abedin, A., S. I. Rizvi, R. Islam, and K. S. Islam. 2021. Comparative study between cationic cotton dyeing and conventional dyeing: An approach towards sustainability. Last updated at November 8, 2021 10:16:46 am. Accessed September 15, 2022. https://www.textiletoday.com.bd/comparative-study-between-cationic-cotton-dyeing-and-conventional-dyeing-an-approach-towards-sustainability/
- Aktek, T., and A. K. M. Malekul Millat. 2017. Salt free dyeing of cotton fiber- a critical review. International Journal of Textile Science 6 (2):21–10. doi:10.5923/j.textile.20170602.01.
- Brenton, A., G. R. P. Morgan, and J. H. Beynon. 1979. Unimolecular ion decomposition. Annual Review of Physical Chemistry 30 (1):51–78. doi:10.1146/annurev.pc.30.100179.000411.
- Chatha, S. A. S., A. I. Hussain, S. Ali, M. J. Saif, A. I. Mallhi, M. Sagir, and M. Y. Naz. 2016. Significance of chitosan to improve of substantivity of reactive dyes. Journal of the Chilean Chemical Society 2 (2):2895–97. doi:10.4067/S0717-97072016000200009.
- Chattopadhyay, D. P. 2001. Cationisation of cotton for low-salt or salt-free dyeing. Indian Journal of Fiber & Textile Research 20:108–15.
- Correia, J., K. Mathur, M. Bourham, F. R. Oliveira, R. Curto Valle, J. A. B. Valle, and A. -F.M. Seyam. 2021. Surface functionalization of greige cotton knitted fabric through plasma amd cationization for dyeing with reactive and acid dyes. Research Square. Accessed 15 November 2022 https://assets.researchsquare.com/files/rs-248369/v1/cc4a0de1-df31-494e-9e47-1a5b969bd4d8.pdf?c=1631887162
- Correia, J., K. T. Rainert, F. R. Oliveira, R. Curto Valle, and J. A. B. Valle. 2020. Cationization of cotton fiber - an integrated view of cationic agents, processes variables, properties, market and future prospects. Cellulose 27 (15):8527–50. doi:10.1007/s10570-020-03361-w.
- Farrell, M. J. 2012. Sustainable cotton dyeing. A dissertation doctor of philosophy. North Carolina: http://repository.lib.ncsu.edu/ir/bitstream/1840.16/7452/1/etd.pdf.
- Genc, N., and E. Can-Dogan. 2006. Photooxidation: A decolorization procedure and a pre-treatment step for biodegradation of reactive dye. Polish Journal of Environmental Studies 15:73–79.
- Ghazal, H. A., A. A. M. El-Masry, and R. M. Mosaad. 2011. Multifunction finishing of cellulose based fabrics via-3-chloro-2-hydroxypropyltrimethylammonium chloride (Quat-188) and silver nanoparticles (AgNps) to improve its dyeability and antibacterial. Biointerface Research in Applied Chemistry 11 (4):11666–78. Accessed 10 May 2021. https://biointerfaceresearch.com/wpcontent/uploads/2020/12/20695837114.1166611678.pdf.
- Giles, C. 1957. The light fastness of dyed fibers-a statistical study. Coloration Technology 73 (4):127–60. April 1957. doi:10.1111/j.1478-4408.1957.tb02185.x.
- Hashem, M., M. El-Bisi, S. Sharaf, and R. Refaie. 2010. Pre-cationization of cotton fabrics. Carbohydrate Polymers 79 (3):533–40. doi:10.1016/j.carbpol.2009.08.038.
- Ilango, V. 2015. Dyeing of cotton using reactive dyes without salt. International Journal of Innovative Research in Technology 2 (7):27–31.
- Kannan, M. S. S., M. Gobalakrishnan, S. Kumaravel, R. Nithyanadan, K. J. Rajashankar, and T. Vadicherala. 2006. Influence of cationisation of cotton on reactive dyeing. Journal of Textile and Apparel, Technology and Management 5 (2):1–16.
- Latif, Z., F. Liu, S. Wen, S. Long, X. -Y. Xiao, L. -N. Lin, and Y. -J. Cai. 2015. Effect of cationic UV absorber on light fastness property of reactive dye. International Conference on Engineering, Science and Applications. ICMSA 2015).
- Lewis, D. M. 2014. Developments in the chemistry of reactive dyes and their application processes, Color. Coloration Technology 130 (6):382–412. doi:10.1111/cote.12114.
- Nelson, L. W.1995. US Patent 5.387.262A, Process for increasing the lightfastness of dyed fabrics. Accessed 15 November 2022. https://patents.google.com/patent/US5387262A/en
- Oakes, J. 2001. Photofading of textile dyes. Review of Progress in Coloration 31(1):21–28. Accessed 15 November 2022. doi:10.1111/j.1478-4408.2001.tb00134.x.
- Pruś, S., P. Kulpiński, E. Matyjas-Zgondek, and K. Wojciechowski. 2022a. Eco-friendly dyeing of cationised cotton with reactive dyes - mechanism of bonding reactive dyes with CHPTAC cationised cellulose. Cellulose 29 (7):4167–82. doi:10.1007/s10570-022-04521-w.
- Pruś, S., P. Kulpiński, E. Matyjas-Zgondek, and K. Wojciechowski. 2022b. Mechanism of bonding reactive dyes with copolymer (chloromethyl)oxirane-1H-imidazole cationised cellulose. Materials 15 (13):4664. doi:10.3390/ma15134664.
- Tera, F. M., E. M. Osman, S. F. Ibrahim, and M. N. Michael. 2016. Quantification of the light fastness of colored substrates by applying fading rate constant values. Egyptian Journal of Chemistry 59 (6):1113–25.
- Thiagarajan, P., and G. Nalankill. 2014. Effect of reactive dyes structure on light fastness. International Journal of Engineering Research & Technology 3 (2): ISSN: 2278-0181.
- Thiagarajan, P., and G. Nalankilli. 2010. A review on light fastness of reactive and other classes of dyes on cotton material. Asian Dyer 7 (6):161–164.