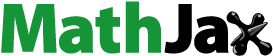
ABSTRACT
In this study, an attempt is made to check whether a hybrid composite made up of both synthetic and natural fibers (Carbon and Cordia Dichotoma respectively) can be made bio-degradable, at least to some extent, without much compromising on the mechanical properties. Hybrid composites were prepared by reinforcing alkali treated Cordia dichotoma and carbon fibers into epoxy resin using hand lay-up method. By varying the number of layers of fibers in the composite specimen and fixing 20% fiber weight for all composites, nine distinct combinations of specimens were prepared. The maximum tensile strength of 386.68 MPa, flexural strength of 647.08 MPa, and impact energy of 4.82 J were obtained for composites produced with pure carbon fiber, whereas hybrid composite exhibit tensile strength of 367.76 MPa, flexural strength of 646.41MPa and impact energy of 4.74 J. The interfacial bonding between the fibers and matrix of tested specimens was studied using a scanning electron microscope (SEM), as well as the arrangement of fibers within the matrix for the manufactured composite. Thermogravimetric analysis (TGA) was used to investigate thermal stability, and it was found that it was thermally stable up to 415°C. 9. Crystallinity value increases from 20% carbon fiber to 20% alkali fiber.
摘要
在这项研究中,试图检查由合成纤维和天然纤维(分别为Carbon和Cordia Dichtotoma)组成的混合复合材料是否可以生物降解,至少在一定程度上不会对机械性能造成太大影响. 采用手糊法将碱处理过的二歧虫草和碳纤维增强为环氧树脂,制备了杂化复合材料. 通过改变复合材料试样中纤维层的数量,并为所有复合材料固定20%的纤维重量,制备了九种不同的试样组合. 纯碳纤维复合材料的最大拉伸强度为386.68 MPa,弯曲强度为647.08 MPa,冲击能为4.82 J,而杂化复合材料的拉伸强度为367.76 MPa,弯曲性能为646.41 MPa,冲击能量为4.74 J. 使用扫描电子显微镜(SEM)研究了测试样品的纤维和基体之间的界面结合,以及所制造的复合材料的纤维在基体内的排列. 热重分析(TGA)用于研究热稳定性,发现其在高达415°C时是热稳定的.9.结晶度值从20%的碳纤维增加到20%的碱纤维.
Introduction
Composite material is formed by the combination of two or more constituent materials. The matrix phase is used to hold the fibers together and also to transport the load to the fibers, and the reinforcing phase is used to take the maximum load, but these two phases are chemically distinct, i.e., there is no chemical reaction between them. This material has high strength with sufficient stiffness and toughness with a light weight. This combination of properties offers a great advantage in mechanical design. So, these properties may be suitable for various industries, such as automotive, aerospace, infrastructure and sports (Jenish et al. Citation2022; Madhu et al. Citation2020; Yashas Gowda et al. Citation2022). Metals are proven engineering materials for various applications in terms of strength and stability. Nowadays, their usage is declining significantly due to high weight and high corrosiveness. Later, synthetic fiber-reinforced composites have become popular, but they are non-biodegradable and costly. To overcome this, natural fibers are introduced due to their biodegradability, low cost, availability, low density, and renewability (Ilyas et al. Citation2019; Senthamaraikannan et al. Citation2016). Mechanical properties of natural fibers are varying in nature (inconsistent) due to their growing environment, extraction methods, and seasons. Therefore, natural fiber-reinforced composites (NFRC) may not be suitable for heavy-load bearing applications and high-temperature applications. Natural fibers derived from plants such as jute, sisal, coir, hemp, and kenaf have attracted great attention as a strengthening element in the NFRC composite (Dos Santos et al. Citation2019; Ramesh et al. Citation2022).
Natural fibers have few limitations, such as quality variations and high moisture absorption, which cause fibers to swell, leading to poor bonding. Natural fibers are hydrophilic in nature due to the presence of lignin, hemicelluloses, waxes, etc. Mechanical strengths of natural fiber-reinforced composite (NFRC) purely depend on the compatibility between fiber and matrix. NFRC composites have poor compatibility or interfacial adhesion due to hydrophilic nature of fiber and hydrophobic nature of matrix. As a result, NFR composite shows reduced mechanical properties. Chemical treatment of fibers removes fiber constituents such as wax, lignin, hemicelluloses, and other substances present over the surface of the fiber. As a result, a rough surface of the fiber is obtained, which is helpful in improving the interfacial bonding with the matrix. Because of alkali treatment, damage to fibers may be less at optimum weight proportions, and cost is also reduced. This increased interfacial bonding can transfer the load more effectively from the fiber to the matrix, improving the mechanical properties.
Reddy et al. (Citation2021) investigated and compared the mechanical properties of composites made with and without alkali-treated Prosopis juliflora fiber and epoxy, concluding that the composite made with alkali-treated fiber has superior mechanical properties. Rizal et al. (Citation2018) studied the mechanical, chemical, FT-IR, and XRD properties of Typha fiber-reinforced epoxy composites after alkali treatment. Compared to untreated Typha fiber composites, 5% of NaOH-treated composites demonstrated improved mechanical characteristics. Many works have employed alkali treatment for natural fibers such as cordia dichotoma (Reddy et al. Citation2020), abutilon indicum fibers (Mohana Krishnudu, Sreeramulu, and Reddy Citation2020) and foxtail millet (Reddy et al. Citation2020) into polymeric matrix composite and reported improved mechanical properties and interfacial bonding between the fibers and the matrix. (Reddy et al. Citation2020).
Hybrid composites are materials made from a common matrix by combining two or more separate types of synthetic fibers or different types of natural fibers, or by combining both synthetic and natural fibers in varied proportions (Shanmugam and Thiruchitrambalam Citation2013). The hybrid composite has superior qualities that a single type of reinforcement can match. When natural and synthetic fibers are used, the cost of a hybrid composite can be greatly reduced. The weight content (Wt.%) of reinforcement and the layer in which synthetic and natural fibers are put influence the hybrid composite’s final characteristics (TG et al. Citation2022). The mechanical properties and vibration characteristics have improved significantly with hybridization of carbon fiber with flax fiber as was investigated by Flynn, Amiri, and Ulven (Citation2016). Ramesh et al. (Citation2017) carried out studies on a banana/carbon fiber-reinforced hybrid composite to determine its mechanical capabilities and water absorption characteristics. The results of this experiment are the highest tensile strength for 20% carbon and 80% banana fiber, the highest flexural strength for pure carbon fiber and the highest water uptake for banana fiber compared to carbon fiber. The physical and mechanical properties of the fiber constituents were used to determine the performance of hybrid composites (Dinesh et al. Citation2020; Vinod et al. Citation2022). The shear strength, hardness, wear resistance, water absorption, and FT-IR properties of the carbon/flax fiber-reinforced composites were fabricated using a hand-lay-up approach. According to the findings, each of the aforementioned features of hybrid composites is comparable to that of pure carbon fiber-reinforced composites. This also suggested that carbon and flax fiber composite hybridization can be used as a structural application while also reducing environmental consequences (Ramesh et al. Citation2018). Nagappan et al. (Citation2022) investigated on the effects of fiber length and fiber content along with alkali treatment effects on the mechanical properties of prepared natural fiber-based polymer composites. The work concluded that the optimum fiber length and fiber weight % for obtaining maximum mechanical properties are 7 mm and 30 wt%, respectively, for both raw and alkali-treated fiber composites.
Sarasini et al. (Citation2016) developed a hybrid composite with two distinct stacking sequences, such as flax/carbon/flax (FCF) and carbon/flax/carbon (CFC) as well as pure carbon and pure flax epoxy composites, and examined their impact and flexural strengths. Results have confirmed that the impact and flexural performance of CFC composite laminate is slightly higher than that of FCF composite laminate, pure carbon and pure flax epoxy composite laminate. Several researchers have reviewed, studied and confirmed that carbon fiber is an effective hybridizing element of both natural and synthetic fiber-reinforced epoxy composite, due to improved mechanical performance, cost-effective material and decreased environmental impact (Jawaid and Khalil Citation2011). Najafi, Khalili, and Eslami-Farsani (Citation2014) manufactured the hybrid composite using pure basalt fibers, carbon fibers, and by altering the weight proportions of basalt fibers and carbon fibers into phenolic resins, and these fibers are stacked in layers within the phenolic resin. The impact and flexural strength of the hybrid composite are improved by incorporating basalt fiber into the carbon fiber-reinforced phenolic resin. BCFP composite with weight proportions of 0.83:0.17 in the stacking sequence B/C/B/C/B has the highest impact and flexural strength. Jebadurai et al. (Citation2021) selected low-cost and widely available coccinia grandis stem fibers and laid the composite by reinforcing it in polyester resin. The mechanical, thermal, and hardness properties of the produced composite were examined to ensure that it was suitable for structural purposes. The highest mechanical properties were reported when 40% by weight of the coccinia grandis fibers were reinforced with polyester resin. Thermal stability (250°C) is also reasonable for the aforementioned hybrid composite. Such hybrid composites are used for a variety of applications, such as car interior, sporting equipment, bone plate and wind turbine blades (Jawaid and Khalil Citation2011; Jothibasu et al. Citation2020; TG et al. Citation2021). Mahakur, Bhowmik, and Patowari (Citation2022) reviewed the various works to identify the parametric effects on the machining of natural fiber-based composites. Work explored the various methods that can be implemented to reduce the machining defects. Rangappa et al. (Citation2022) reviewed the use of cellulosic fiber as a reinforcing element in the preparation of composites. The research work explored the recent developments on the utilization of cellulosic fibers in the polymer industries focusing much on the improvement of the mechanical properties, manufacturing methods and its applications in various industries.
The goal of this research is to combine carbon fiber with alkali-treated cordial dichotoma fiber in an epoxy matrix using a hand-lay-up method and compression molding. The study on alkali-treated Cordia Dichotoma fiber-reinforced epoxy composite with carbon fiber was not published in the literature. Carbon fiber and alkali-treated Cordia Dichotoma fibers reinforced with epoxy resin were used to create a hybrid composite. In this study experimental investigations were carried out to determine the tensile, flexural, and impact properties of Cordia dichotoma fiber and carbon fiber-reinforced epoxy composites and also characterization using scanning electron microscopy (SEM), Fourier transformation infrared spectroscopy (FT-IR), and thermo-gravimetric analysis (TGA). The mechanical, thermal and SEM morphology could be applied in structural, automotive, wind turbine blades, aerospace, and infrastructure applications.
Materials and methods
Materials
Cordia dichotoma fiber, carbon fiber, and epoxy resin are the materials utilized to make hybrid composites. Cordia dichotoma fibers are harvested in and around the village of Dorigallu in Andhra Pradesh, India. Ram Composite Products, Hyderabad, Telangana, India, provided carbon fiber (unidirectional mat 200 gsm). Ram Composite Products, Hyderabad, Telangana, provided the epoxy resin (LY556 with viscosity at 250°C: 0,7 ÷ 1, 1 Pa.s), which is a diglycidyl ether of biphenyl-A, and the hardener (HY 951 with specific gravity at 25°C: 0.95 g/cm3), which is triethylenetetramine.
Fiber extraction
The cordia dichotoma fibers were extracted from the bark of the cordia dichotoma tree by a water retting process followed by mechanical beating. Extracted fiber contains foreign impurities and wax substances; this has been removed by shaking and squeezing of the fiber in running water. Natural fibers are hydrophilic in nature because of their fiber constituents, such as lignin, wax, pectin, etc., which are responsible for water absorption and also lead to poor interfacial bonding. To overcome such phenomenon, a surface modification called alkali treatment is carried out on the fibers. shows the sample fabrication technique for hybrid composite laminates.
Alkali treatment
Alkali treatment is simple and economic for treating the natural fibers. In a separate beaker, create the alkali solution (5% NaOH) by dissolving 100 g of NaOH pellets in 2 L of pure water. After that, the dry fiber is soaked in the alkaline solution for 2 h at room temperature. The fibers were removed from the solution and thoroughly washed with distilled water to remove any NaOH that had adhered to the fiber surface. Hemicelluloses and other contaminants that cling to the fiber are also removed. The fibers were dried in an oven at a continuous temperature of 100 degrees Celsius for 24 h.
Fabrication of composites
For this work, the laminates of the hybrid composites were prepared using a hand lay-up method. Well-dried cordia dichotoma (D) and carbon (C) fibers are segregated and chopped to a length of 150 mm. Nine different forms of laminates have been prepared with different stacking sequencesD/D/D/D (Sample 1), D/D/C/D (Sample 2), C/D/D/D (Sample 3), D/C/D/C (Sample 4), C/D/C/D (Sample 5), C/D/D/C (Sample 6), C/C/D/C (Sample 7), C/C/C/D (Sample 8) and C/C/C/C (Sample 9) as shown in . Along with a pure epoxy composite, pure alkali-treated cordia dichotoma fiber-reinforced epoxy composite and pure carbon fiber-reinforced epoxy composites were manufactured to compare mechanical properties. The total fiber content was fixed at 20 wt%. Epoxy resin and hardener were properly mixed with the 100:10 weight ratio in a separate beaker. A glass mold with a size of 150 mm x 150 mm x 3 mm was used to prepare the composite laminates. Releasing gel was spread over the surface of mold for easy removal of composite laminate. A thin layer of epoxy mixture was applied on the surface of the mold, and then carbon fibers were uniformly laid over the mixture, and the required amount of epoxy mixture was poured over the carbon fiber. After proper impregnation of epoxy mixture on carbon fiber, cordia dichotoma fibers were mounted on the first layer of carbon fiber. This procedure was continued for the rest of layers (four layers). The remaining mixture of resin was poured on top of fourth layer and roller was used to distribute the epoxy mixture and to eliminate air gaps formed between the layers during the processing. Releasing gel was applied to OHP (Over Head Projector) sheet and is placed above it to obtain a smooth surface. For 24 h, a weight of 20 kg was loaded onto each mold to obtain the required dimensions of the laminate and cure the laminate. shows the reinforcement sequence arrangement in various composite samples.
Table 1. Layer designation and fiber weight content (wt. %) in hybrid composite.
Characterization
Tensile strength test
The tensile strength of a material refers to its ability to be stretched without breaking under uniaxial force. The INSTRON Universal Testing Machine (Model-3369) with a load cell capacity of 10 kN was used to determine tensile parameters such as tensile strength and the tensile modulus of hybrid material. With a dimension of 150 mm x 15 mm x 3 mm, a tensile test was performed according to the ASTM standard ASTM: 3039. Each hybrid composite sample was tested three times, with the average values recorded.
Flexural strength test
The INSTRON Universal Testing Machine (Model-3369) was used to assess the flexural properties of prepared hybrid composites under specific conditions (crosshead speed rate: 10 mm/min; load cell: 10 kN; support span length: 50 mm). The flexural characteristics of all prepared hybrid composite samples were tested using the three-point bending method. Samples were flexural tested in accordance with ASTM D 760–03 standards. For each of the hybrid composites, three samples were examined, and the average values were recorded.
Impact strength test
When impact or abrupt loads are applied, composite impact strength is defined as the amount of energy absorbed during fracture and was determined using an IZOD impact tester (Composite Lab-GPREC, Kurnool). The composite sample was impact tested according to the ASTM D-256 standard, with a test sample size of 63.5 mm x 12.7 mm x 3 mm.
Scanning Electron Microscope (SEM) analysis
SEM is an effective instrument for determining the failure mechanism, mode of failure, and interfacial bonding (between matrix and fiber) of a failed specimen. The JEOL/EO Model-JSM-6390 was used to investigate the cracked surface of the hybrid composite sample. For this analysis, the fractured surfaces of the hybrid composite samples were washed, air dried and a thin gold film of gold (3 μm) is coated with JEOL sputter ion coater to impart the conductivity of the sample, and SEM was observed at a voltage of 10 kV and 15 kV.
Thermo-gravimetric Analysis (TGA)
TGA (thermo-gravimetric analysis) is a technique for determining a material’s thermal characterization by measuring its mass change as a function of temperature. A thermo-gravimetric analyzer (NETZSCH STA 2500) and differential thermogravimetry were used to evaluate the thermal stability of a hybrid composite (DTG). In a nitrogen atmosphere, a weighted quantity of composite sample was deposited in a platinum plate and heated to a temperature range of 26°C to 850°C at a rate of 20°C/min (N2).
FTIR spectroscopy
In hybrid composite samples, Fourier transform infrared spectroscopy (Model-Thermo Nicolet, Avatar 370) was utilized to detect functional groups, chemical linkages, and their absorption bands. When the wavelengths of infrared (IR) rays match the vibrational wavelength of a bond with a functional group, absorption of bands occurs. The FTIR spectra of hybrid composites were collected with 32 scans with a resolution of 4 cm−1 in the spectral region of 4000 cm−1 to 400 cm−1.
Results and discussion
Tensile test
designates the laminates and displays the experimental results of the tensile tests. The stress–strain plot is shown in . Tensile strength is observed to be the highest (386.68 MPa) in only carbon laminate i.e., (C/C/C/C) and the lowest strength (63.62 MPa) in pure cordia dichotoma epoxy composites (D/D/D/D). The addition of carbon fiber to natural fiber-reinforced composite laminate increases the tensile strength as carbon fiber is stronger and stiffer than natural fibers (Saravanakumar et al. Citation2013). The same has been clearly observed in the present work. A carbon fiber layer that occupies the place of one of the layers of natural fibers in (D/D/D/D) resulted in an improvement in tensile strength (Sample 2 D/D/C/D) by 74% to 110.98 MPa and Sample 3 (C/D/D/D) by 109% to 133.11 MPa). The reason why the tensile strength of sample 3 is higher compared to Sample 2 may be because the carbon fiber bearing the total load acts on it and gradually transferring to other layers of natural fibers. To further increase the tensile strength of the composite sample, two layers of carbon fibers are introduced at different positions of sample 1 replacing natural fibers (D/C/D/C, C/D/C/D, C/D/D/C). Tensile strength improved by 168%, 248% and 300%, respectively, compared to (D/D/D/D). The layers of carbon fibers at both ends of the sample of C/D/D/C, could’ve resisted the pulling force greatly, and hence the tensile strength is higher between the two layers of carbon fibers (Sample 4 and Sample 5). Similar results were obtained by (Venkatasudhahar, Kishorekumar, and Dilip Raja Citation2020) for the hybrid composite laminate with Carbon-Jute-Jute-Jute-Carbon layering sequence possessing superior mechanical properties than composite laminate without hybridizing elements such as pure banana (B-B-B-B-B) and pure jute fiber (J-J-J-J-J). Similarly, the strengths of the laminates with three layers of carbon fiber (C/C/D/C and C/C/C/D) increased by 342% and 478%, respectively, compared to those of Sample 1 (D/D/D/D). Three contiguous layers of carbon fibers appear to have added strength to sample 8 (C/C/C/D). In , the stress–strain curve grows linearly to the elastic limit before declining due to the brittle nature of the composite. This is in accordance with (Kureemun et al. Citation2018) that proved incorporation of carbon fiber into un-hybridized composite laminate results in an increase in the linearity of the composite laminate. Tensile strain increases with the number of carbon fiber layers in the hybrid composite laminate, as shown in .
Figure 2. Tensile stress–strain curves of carbon and cordia dichotoma fiber-reinforced hybrid composite laminate.

Table 2. Tensile properties of carbon and cordia dichotoma fiber-reinforced hybrid composite laminate.
Flexural test
shows flexural stress versus strain graph of the plain carbon fiber, pure cordia dichotoma fiber and carbon-cordia dichotoma-reinforced epoxy hybrid composite subjected to flexural loading and results are displayed in . The highest flexural strength (647.08 MPa) was observed in only carbon epoxy composite Sample (C/C/C/C), while the lowest strength (174.03 MPa) was observed in pure cordia dichotoma epoxy composites (D/D/D/D). The addition of carbon fiber to natural fiber-reinforced composite laminate increases the flexural strength as carbon fiber is stronger and stiffer than natural fibers (Saravanakumar et al. Citation2013). The same has been clearly observed in the present work. A layer of carbon fiber occupying the place of one of the layers of natural fibers in (D/D/D/D) resulted in the improvement of the flexural strength Sample 2 (D/D/C/D) by 34% to 234.12 MPa and Sample 3 (C/D/D/D) has 99% to 319.62 MPa). The reason why the flexural strength of sample 3 is higher than that of sample 2 may be because the carbon fiber carries the total flexural load acting on it and gradually transfers to other layers of natural fibers. To further increase the flexural strength of the composite laminate, two layers of carbon fibers are introduced at different positions of sample 1 replacing natural fibers (D/C/D/C, C/D/C/D, and C/D/D/C). Flexural strength improved by 137%, 144% and 163%, respectively; compared to epoxy composite laminate reinforced with pure cordia dichotoma fiber (D/D/D/D). The layers of carbon fibers at both ends of the sample of C/D/D/C could have resisted the flexural force greatly and therefore the flexural strength is higher among the two layered carbon fiber samples (Sample 4 and Sample 5). Similar results were obtained by (Venkatasudhahar, Kishorekumar, and Dilip Raja Citation2020) for the hybrid composite laminate with a carbon-jute-jute-carbon layering sequence that possesses superior mechanical properties than the composite sample without hybridizing elements such as pure banana (B-B-B-B) and pure jute fiber (J-J-J-J-J). Similarly, the strengths of the samples with three layers of carbon fiber (C/C/D/C and C/C/C/D) were enhanced by 225% and 271%, respectively, compared to that of Sample 1 (D/D/D/D). Three contiguous layers of carbon fibers appear to have added strength to sample 8 (C/C/C/D). The flexural strength of Samples 8 and 9 is nearly the same, indicating that the use of natural fiber in the Samples improves the load-bearing capacity. Another notable finding from is that increasing the carbon fiber content while decreasing the natural fiber content increases the composite sample’s flexural strength and flexural modulus. Due to the brittle nature of the composite, the flexural stress vs. strain graph grows linearly to the elastic limit and then decreases.
Figure 3. a) Flexural stress–strain curves of carbon and cordia dichotoma fiber-reinforced hybrid composite laminate b) Composite laminate vs Elastic/Flexural modulus.

Table 3. Flexural properties of carbon and cordia dichotoma fiber-reinforced hybrid composite laminate.
The carbon/flax hybrid composite laminate has superior flexural properties compared to the unhybridized composite laminate. Carbon fiber has stiffer and stronger than natural fibers. Showing that hybridization with synthetic fibers is an effective method to improve mechanical properties (Dhakal et al. Citation2013). In general, natural fiber composite laminate has a non-linear behavior, which has been reduced by reinforcing synthetic fiber, such as carbon fiber in hybrid composite. This behavior is purely dependent on the amount of weight percentage (wt. %) of carbon fiber used in hybrid composite laminate (Kureemun et al. Citation2018).
Impact test
The impact strength of nine samples composed of carbon, cordia dichotoma fibers, and epoxy components is evaluated using an impact test. The impact strength of various samples is determined by applying a transverse force with a hammer on the Izod impact tester, and the findings are presented in . When a transverse load is applied to the specimen, crack propagation occurs followed by fiber breakage and pull-out. Sample 9 (C/C/C/C) shows the highest impact strength (4.82 J) compared to the other samples, which happens due to the presence of carbon fibers in the sample. The impact strength of the sample of the pure cordia dichotoma fiber composite (Sample 1) has a lower impact strength (1.26 J) than the carbon fiber hybrid composite. With the addition of one layer of carbon fiber (Samples 2 & 3) at different positions in the sample, a sharp increase in impact strength is observed, which is 60% and 171% higher than the sample of pure cordia dichotoma fiber composite. The reason that impact strength of Sample 3 is more compared to Sample 2 may be because of carbon fiber resists the impact load acting on it and gradually transferring to other layers of natural fibers. Two layers of carbon fibers are placed at different positions of the Sample (Sample 4, 5 & 6) which increases the impact strength by 220%, 231%, & 242% when compared to pure cordia dichotoma fiber composite. A composite with three or more than three layers of the composite at different positions increases impact strength by 261%, 271%, and 282% relative to the sample of pure cordia dichotoma fiber composite sample. Layers of carbon fibers at both ends of the Sample C/D/D/C, could have resisted the impact load greatly and therefore the impact strength is higher among the two layered carbon fiber samples (Sample 4 and Sample 5). Similarly, the strengths of the samples with three layers of carbon fiber (C/C/D/C and C/C/C/D) improved by 262% and 276%, respectively, compared to that of Sample 1 (D/D/D/D). Three contiguous layers of carbon fibers appear to have added strength to sample 8 (C/C/C/D). From , it was observed that an increase of the carbon fiber layer in the hybrid sample increases the impact strength; here a lot of energy is absorbed by the carbon fiber. Impact strength was also affected by the damping of the constituent materials (Assarar et al. Citation2015; RaviKumar et al. Citation2021) and height of dropping weight (Sarasini et al. (Citation2014). The reason for low impact strength in the case of composite sample with cordia dichotoma fiber is due to the fiber breakage and fiber de bonding (Tranchard et al. Citation2017).
Figure 4. Impact strength of carbon and cordia dichotoma fiber-reinforced hybrid composite laminate.

Table 4. TG analysis results in terms of thermal degradation temperature and residual content.
Thermo-gravimetric Analysis (TGA)
One of the most important methodologies for investigating the thermal stability of hybrid composite samples is thermo-gravimetric analysis. The rate of mass loss of the sample as a function of temperature was used to determine its thermal stability. Different hybrid composite samples such as pure cordia dichotoma fiber (D/D/D/D), varying the layers of carbon-cordia dichotoma fiber (C/D/D/C) and pure carbon fiber (C/C/C/C) were prepared, and subjects to TG analysis and obtained thermographs are shown in .
Figure 5. Thermographs of Alkali treated cordia dichotoma, carbon fiber & cordia dichotoma and pure carbon fiber-reinforced epoxy composite.

The onset temperature (To) of the thermograph of pure alkali treated composite (D/D/D/D) sample is approximately 120°C, and at this temperature, 4% mass loss was detected, which could be related to the removal of captured moisture content and water vapors contained in the sample. The predominant thermal breakdown of cellulosic component materials is thought to take place between 200°C and 400°C. Similar trend of mass loss was also observed in thermographs of hybrid composite (C/D/D/C) and pure carbon fiber (C/C/C/C) composite sample, which has onset temperature of 130°C and 145°C. The thermal stability gradually declines as the temperature rises, and thermal disintegration occurs in two stages. For the pure cordia dichotoma fiber (D/D/D/D) reinforced composite sample, the first step of thermal deterioration occurs at temperatures ranging from 210°C to 380°C. This can be attributed to the degradation of the content of hemicelluloses present in the fiber. At this step, the mass loss of the sample was about 6%. In the case of thermograph of hybrid composite sample, almost similar type of thermal degradation temperature 210°C–380°C and same amount of mass loss (6%) was observed after hybridizing the carbon fiber. However, a pure carbon fiber (C/C/C/C) reinforced composite sample with a thermal breakdown temperature of 300°C to 450°C and a moderate mass loss of 4% showed increased thermal stability. The degradation of organic-based sizing compounds in carbon fiber can be attributed to it (Tranchard et al. Citation2017; Yatim et al. Citation2020). For pure alkali treated cordia dichotoma fiber (D/D/D/D) composite samples, the second step of disintegration occurs at temperatures ranging from 430°C to 500°C. In this temperature range, the mass loss was nearly 81%. Because cellulose is more heat resistant than the other fiber elements, the content of epoxy, cellulose, and lignin content may degrade in this temperature range. Similarly, in the case of hybrid composite sample (C/D/D/C), nearly the same thermal behavior was observed in a temperature range of 430°C to 500°C, but the mass loss of the sample was 90%. However, the pure carbon fiber composite laminate has improved thermal stability from a temperature range of 460°C to 600°C. The mass loss of the sample was nearly 72%. It has been suggested that it is linked to carbon fiber degradation. The alkali-treated cordia dichotoma fiber-reinforced composite laminate (D/D/D/D); hybrid composite with carbon and cordia dichotoma fiber (C/D/D/C); and pure carbon fiber-reinforced composite sample had a final residual mass of approximately 15.38%, 10.53%, and 26.92%, respectively, at 849.6°C 850°C, and 849.7°C. The use of carbon fiber increased the thermal stability of hybrid composites.
Tranchard et al. (Citation2017) studied the thermal decomposition of a carbon fiber/epoxy composite sample by using TG analysis, and findings show that thermal decomposition happens at one stage of degradation at a temperature of 300°C to 500°C in the nitrogen atmosphere correlated to organic sizing compounds on the carbon fiber. This thermal stability of the hybrid composite laminate is applicable for aircraft applications. Meyer, Schulte, and Grove-Nielsen (Citation2009) reported related findings of restored carbon fiber at 550°C in nitrogen after the first step of decomposition. Several authors carried out a TG analysis and the results obtained, such as the temperature of thermal degradation temperature and the final residual content, are recorded in .
Fourier transform infrared spectroscopy (FTIR)
shows the FTIR spectra of alkali treated cordia dichotoma reinforced epoxy composite (D/D/D/D), the carbon fiber and cordia dichotoma fiber-reinforced epoxy composite (C/D/D/C), and pure carbon fiber-reinforced epoxy composite (C/C/C/C/C). The H-bonded O-H group in the D/D/D/D composite was found to have a broad and high absorption peak at 3450 cm−1 (Yap, Que, and Chia Citation1991). This usually means that a good number of O-H groups and H-bonds were associated with each other in the composite sample. For C/D/D/C composites, a similar type of intensity peak is observed, whereas a shift in peak position in terms of magnitude is observed at 3439 cm−1. Similar type of intensity peak was observed for C/D/D/C composites, while the peak position shifted to 3439 cm−1 in magnitude. In the case of C/C/C/C composites, a weak intensity of the peak and a different magnitude peak shift (3405 cm−1) were observed due to changes in the H-bonding and O-H groups in the composite. A noticeable peak at 3049 cm−1indicates the asymmetric stretching of C-CH3 bond of its chemical formula is (CH3C≡CCH3) in C/D/D/C composite, whereas no such peak is observed in the other two composites (D/D/D/D and C/C/C/C) (Sivaraman et al. Citation2015). The weak absorption peak at 2925 cm−1 corresponds to the α-cellulose O-H group in the compound D/D/D/D, indicating a reduced cellulose content after alkali treatment. In C/D/D/C composite, the rich content of cellulose is mainly responsible for shifting the absorption peak to 2961 cm−1. But the absorption peak position is shifted to 2961 cm−1 due to rich content of cellulose present in the C/D/D/C composite. The narrow absorption peak was observed in the C/C/C/C composite at 2920 cm−1, which represents the rich α-cellulose and O-H groups within it (Jayaramudu et al. Citation2011). The weak absorption peaks at 2856 cm−1 (D/D/D/D), 2875 cm−1 (C/D/D/C), and 2853 cm−1 (C/C/C/C) were attributed to the methyl (-CH3) and methylene (-CH2-) groups present in it (Tran, Bénézet, and Bergeret Citation2014). The absorption peak at 1639 cm−1 in the D/D/D/D composite confirms the rich carbonyl groups (C=O) present in it, a decrease in carbonyl groups was observed in the C/D/D/C and C/C/C/C composites due to the hybridization of carbon fiber with the reinforced epoxy composite (Yap, Que, and Chia Citation1991). All composites (D/D/D/D, C/D/D/C and C/C/C/C) exhibit similar absorption peaks at around 1508 cm−1 confirms the presence of aromatic C-H bond stretching in lignin (Yap, Que, and Chia Citation1991). Similar absorption peaks appeared around 1508 cm−1 on the curves of D/D/D/D, C/D/D/C, and C/C/C/C composites, which can be recognized as C-H bond stretching, which is a characteristic of aromatic molecules. The oxidative alcohol stretching of the aliphatic ester group in lignin correlates with the absorption peak in the D/D/D/D compound at 1252 cm−1. Peaks in both C/D/D/C and C/C/C/C composites were reduced to around 1243 cm−1, indicating a reduction in ester and a drop in lignin (Tran, Bénézet, and Bergeret Citation2014). This FTIR spectrum shows the establishment of a bond between the fiber and the matrix. These results are consistent with previous research findings reported in the literature by other authors (Tran, Bénézet, and Bergeret Citation2014; Yap, Que, and Chia Citation1991).
Scanning Electron Microscope (SEM) analysis
The surface morphology and fractured surface area of alkali treated cordia dichotoma fibers (D/D/D/D), carbon fiber & cordia dichotoma fiber (C/D/D/C), and epoxy reinforced composites reinforced with pure carbon fiber (C/C/C/C) were studied as shown in .
show the proper wetting of cordia dichotoma fibers, carbon fibers and hybridization of these fibers into the epoxy composite. Alkali treatment (5% NaOH) (D/D/D/D) removes impurities, hemicelluloses, and unwanted fiber constituent materials from the fiber surface and makes the fiber surface rougher and uneven, resulting in better mechanical interlocking (). This mechanism results in better stress transfer with improved mechanical properties. It was also revealed that fewer amounts of fiber pullouts were observed due to alkali treatment. The FTIR spectra () indicated that there is a strong interfacial bond between the fiber matrix due to the elimination of hydroxyl groups on the fiber surface after alkali treatment, thus improving the mechanical properties of the composite. Alkali treatment affects the innate properties of fiber such as structural, chemical and morphology.
indicates strong interfacial adhesion between the carbon fiber and epoxy matrix (C/C/C/C), as the epoxy matrix is properly infused into carbon fibers. shows a strong interaction between the hybridized fibers (C/D/D/C) and the epoxy matrix that leads to improved mechanical properties. SEM micrograph of cordia dichotoma fiber and carbon fiber shows the predominance of brittle fiber failures and the indication of ductile failure of the matrix can be seen from .
XRD analysis
The %-crystallinity and %-amorphous of the cordia-Dichotoma fiber, cordia-Dichotoma/carbon fiber and carbon fibers-reinforced hybrid epoxy composites were examined by X-ray diffraction (Model: Bruker D8 Advance) technique (XRD) and obtained results are shown in . The values for %-crystallinity of carbon fibers, cordia-Dichotoma/carbon fiber and cordia-Dichotoma fiber-reinforced hybrid epoxy composites are 39.1, 44.2 and 54, respectively. From , %-Crystallinity value increases from 20% carbon fiber to 20% alkali fiber. The more crystalline a fiber, the more regularly aligned its chains. Increasing the degree of crystallinity increases hardness and density (Vijay et al. Citation2021).
shows the X-ray diffraction of a composite made of cordia dichotoma fibers and epoxy. Clear reflections can be seen in microcrystalline cellulose at 2θ = 22.5, 24.30, 29.82, 35.87, 38, 39.72 and 43.5º, which correspond to the planes (002), (012), (104), (110), (113), (113) and (202), respectively. The lattice planes of glycosidic rings, which are the densely charged structures in the cellulose chain, correlate to the maximum reflection plane (012) in terms of charge density. Compounds such as chromium (Cr), sucrose (C12H22O11), and calcite (CaCO3) can be found using the pattern 00-006-0694 JCPDS-ICDD with X’Pert High Score Plus software.
shows X-ray diffractograms of a cordia dichotoma and carbon fibers reinforced epoxy hybrid composite sample. Clear reflections can be seen in microcrystalline cellulose at 2θ = 21.35, 22.5, 24, 26.5, 28, 30, 31, 34.5, 38, 40, 47, 54.5, 73, 76 and 83º, which correspond to the planes (100), (100), (101), (101), (111), (104), (200), (110), (113), (102), (220), (222), (331), (420) and (422), respectively. Compounds such as Fluorite (Ca F2), Calcite (CaCO3), Quartz (Si 02) (pattern 00–035–0816 JCPDS-ICDD) can be detected from X’Pert High Score Plus software.
shows an X-ray diffraction image of a carbon fiber-reinforced epoxy composite sample. Microcrystalline cellulose exhibits clear reflections about 2θ = 21.30, 23.5, 26.5, 30, 31.5, 35, 39, 44.5, 46.5, 57.5, 69, 76.5 and 83.5º and corresponding to the planes (100), (012), (101), (104), (006), (110), (113), (202), (024), (122), (217), (220), and (134), respectively. The elements Calcite (CaCO3), Chromium (Cr), Quartz (Si 02) (pattern 00–005–0586 JCPDS-ICDD) can be recognized from X’Pert High Score Plus software.
The Crystallite Size (CS) of the hybrid composite is calculated by the following Scherrer’s formula mentioned in equation 1 (Saravanakumar et al. Citation2014).
Where K = Scherrer’s constant 0.68 to 2.08, 0.94 for spherical crystallites with cubic symmetry and it is, “β” denotes the peak’s full-width at half-maximum (HWFM) and “λ” denotes the wavelength of the radiation = 1.54060 Å. ϴ is the Bragg’s angle in degrees. X-ray diffractograms of cordia dichotoma fibers reinforced epoxy composite = 14.66 nm. X-ray diffractograms of cordia dichotoma and carbon fibers reinforced epoxy composite = 17.94 nm and the X-ray diffractograms of carbon fibers reinforced epoxy composite = 30.54 nm.
Conclusion
Current research focused on cordia dichotoma fiber-based epoxy composites and carbon fiber hybridization into cordia dichotoma fiber-reinforced epoxy composites, as well as their potential impact on tensile, flexural, and impact properties, thermal stability, structural, and morphological behavior, with the following findings:
The addition of carbon fiber to cordia dichotoma fiber-reinforced epoxy hybrid composites increased mechanical properties such as tensile, flexural, and impact capabilities.
Tensile strength of the laminates with three layers of carbon fiber (C/C/D/C and C/C/C/D) increased by 342% and 478%, respectively, compared to those of Sample 1 (D/D/D/D). This increase in mechanical properties may be suitable for aerospace, structural, infrastructural, packaging, sports, and automobile applications.
Flexural strength of the samples with three layers of carbon fiber (C/C/D/C and C/C/C/D) was enhanced by 225% and 271%, respectively, compared to that of Sample 1 (D/D/D/D).
Impact strength of the samples with three layers of carbon fiber (C/C/D/C and C/C/C/D) improved by 262% and 276%, respectively, compared to that of Sample 1 (D/D/D/D).
The SEM morphology revealed that good interfacial bonding and fewer pullouts were observed between the cordia dichotoma and epoxy matrix. Both natural fibers and synthetic fibers are present in the same composite, and their alignment is proper, and epoxy is infused properly between the fibers, resulting in minimal defects and small voids in the hybrid composite.
The alkali-treated cordia dichotoma fiber-reinforced composite laminate (D/D/D/D); hybrid composite with carbon and cordia dichotoma fiber (C/D/D/C); and pure carbon fiber-reinforced composite sample had a final residual mass of approximately 15.38%, 10.53%, and 26.92%, respectively, at 849.6°C 850°C, and 849.7°C.
A hybrid composite containing cordia dichotoma fiber and carbon fiber performed better in terms of heat stability, while a pure carbon fiber-reinforced epoxy composite performed best.
The prepared hybrid composites are suited for a variety of applications like banners, fabrication of pipes and cylinders where the temperature is less than 450°C.
Crystallinity value increases from 20% carbon fiber to 20% alkali fiber.
Highlights
The addition of carbon fiber to cordia dichotoma fiber-reinforced epoxy hybrid composites increased mechanical qualities such as tensile, flexural, and impact capabilities.
Tensile and flexural test revealed that carbon fibers-reinforced cordia dichotoma fiber epoxy composite gives enhanced strength and modulus.
The SEM morphology revealed that good interfacial bonding and fewer pullouts were observed between the cordia dichotoma and epoxy matrix.
The carbon hybridized composite’s residual char yield was found to be higher than cordia dichotoma composites without carbon fiber hybridization.
Disclosure statement
No potential conflict of interest was reported by the author(s).
References
- Aisyah, H. A., M. T. Paridah, S. M. Sapuan, A. Khalina, O. B. Berkalp, S. H. Lee, C. H. Lee, N. M. Nurazzi, N. Ramli, M. S. Wahab, et al. 2019. Thermal properties of Woven Kenaf/Carbon fibre-reinforced Epoxy Hybrid composite panels. International Journal of Polymer Science 2019:1–19. doi:10.1155/2019/5258621.
- Assarar, M., W. Zouari, H. Sabhi, R. Ayad, and J. M. Berthelot. 2015. Evaluation of the damping of hybrid carbon–flax reinforced composites. Composite Structures 132:148–54. doi:10.1016/j.compstruct.2015.05.016.
- Dhakal, H. N., Z. Y. Zhang, R. Guthrie, J. MacMullen, and N. Bennett. 2013. Development of flax/carbon fibre hybrid composites for enhanced properties. Carbohydrate Polymers 96 (1):1–8. doi:10.1016/j.carbpol.2013.03.074.
- Dinesh, S., P. Kumaran, S. Mohanamurugan, R. Vijay, D. Lenin Singaravelu, A. Vinod, M. R. Sanjay, Suchart Siengchin, K. Subrahmanya Bhat, and S. Siengchin. 2020. Influence of wood dust fillers on the mechanical, thermal, water absorption and biodegradation characteristics of jute fiber epoxy composites. Journal of Polymer Research 27 (1):1–13. doi:10.1007/s10965-019-1975-2.
- Dos Santos, J. C., L. A. de Oliveira, L. M. G. Vieira, V. Mano, R. T. Freire, and T. H. Panzera. 2019. Eco-friendly sodium bicarbonate treatment and its effect on epoxy and polyester coir fibre composites. Construction and Building Materials 211:427–36. doi:10.1016/j.conbuildmat.2019.03.284.
- Flynn, J., A. Amiri, and C. Ulven. 2016. Hybridized carbon and flax fiber composites for tailored performance. Materials & Design 102:21–29. doi:10.1016/j.matdes.2016.03.164.
- Ilyas, R. A., S. M. Sapuan, M. R. Ishak, and E. S. Zainudin. 2019. Sugar palm nanofibrillated cellulose (Arenga pinnata (Wurmb.) Merr): Effect of cycles on their yield, physic-chemical, morphological and thermal behavior. International Journal of Biological Macromolecules 123:379–88. doi:10.1016/j.ijbiomac.2018.11.124.
- Jawaid, M. H. P. S., and H. P. S. Khalil. 2011. Cellulosic/Synthetic fibre reinforced polymer hybrid composites: A review. Carbohydrate Polymers 86 (1):1–18. doi:10.1016/j.carbpol.2011.04.043.
- Jayaramudu, J., E. R. Arjun Maity, B. R. Sadiku, A. Guduri, R. Varada, C. V. Ramana, and R. Li. 2011. Structure and properties of new natural cellulose fabrics from Cordia dichotoma. Carbohydrate Polymers 86 (4):1623–29. doi:10.1016/j.carbpol.2011.06.071.
- Jebadurai, S. G., R. D. E. Raj, V. S. Sreenivasan, and J. S. Binoj. 2021. Coccinia grandis stem fiber polymer composite: Thermal and mechanical analysis. Iranian Polymer Journal 30 (4):369–80. doi:10.1007/s13726-020-00896-4.
- Jenish, I., S. G. Veeramalai Chinnasamy, S. Basavarajappa, S. Indran, D. Divya, Y. Liu, M. R. Sanjay, and S. Siengchin. 2022. Tribo-mechanical characterization of carbonized coconut shell micro particle reinforced with Cissus quadrangularis stem fiber/epoxy novel composite for structural application. Journal of Natural Fibers 19 (8):2963–79. doi:10.1080/15440478.2020.1838988.
- Jothibasu, S., S. Mohanamurugan, R. Vijay, D. Lenin Singaravelu, A. Vinod, and M. R. Sanjay. 2020. Investigation on the mechanical behavior of areca sheath fibers/jute fibers/glass fabrics reinforced hybrid composite for light weight applications. Journal of Industrial Textiles 49 (8):1036–60. doi:10.1177/1528083718804207.
- Kureemun, U., M. Ravandi, L. Q. N. Tran, W. S. Teo, T. E. Tay, and H. P. Lee. 2018. Effects of hybridization and hybrid fibre dispersion on the mechanical properties of woven flax-carbon epoxy at low carbon fibre volume fractions. Composites Part B: Engineering 134:28–38. doi:10.1016/j.compositesb.2017.09.035.
- Madhu, P., M. R. Sanjay, A. Khan, A. A. Otaibi, S. A. Al-Zahrani, S. Pradeep, S. Siengchin, P. Boonyasopon, and S. Siengchin. 2020. Hybrid effect of PJFs/E-glass/Carbon fabric reinforced hybrid epoxy composites for structural applications. Journal of Natural Fibers 19 (10):3742–52. doi:10.1080/15440478.2020.1848724.
- Mahakur, V. K., S. Bhowmik, and P. K. Patowari. 2022. Machining parametric study on the natural fiber reinforced composites: A review. Proceedings of the Institution of Mechanical Engineers, Part C: Journal of Mechanical Engineering Science 236 (11):6232–49. doi:10.1177/09544062211063752.
- Meyer, L. O., K. Schulte, and E. Grove-Nielsen. 2009. CFRP-recycling following a pyrolysis route: Process optimization and potentials. Journal of Composite Materials 43 (9):1121–32. doi:10.1177/0021998308097737.
- Mohana Krishnudu, D., D. Sreeramulu, and P. V. Reddy. 2020. Alkali treatment effect: Mechanical, thermal, morphological, and spectroscopy studies on abutilon indicum fiber-reinforced composites. Journal of Natural Fibers 17 (12):1775–84. doi:10.1080/15440478.2019.1598917.
- Nagappan, S., S. P. Subramani, S. K. Palaniappan, and B. Mylsamy. 2022. Impact of alkali treatment and fiber length on mechanical properties of new agro waste Lagenaria Siceraria fiber reinforced epoxy composites. Journal of Natural Fibers 19 (13):6853–64. doi:10.1080/15440478.2021.1932681.
- Najafi, M., S. M. R. Khalili, and R. Eslami-Farsani. 2014. Hybridization effect of basalt and carbon fibers on impact and flexural properties of phenolic composites.Iranian. Iranian Polymer Journal 23 (10):767–73. doi:10.1007/s13726-014-0272-5.
- Ramesh, M., R. Bhoopathi, C. Deepa, and G. Sasikala. 2018. Experimental investigation on morphological, physical and shear properties of hybrid composite laminates reinforced with flax and carbon fibers. Journal of the Chinese Advanced Materials Society 6 (4):640–54. doi:10.1080/22243682.2018.1534609.
- Ramesh, M., C. Deepa, M. Tamil Selvan, and K. H. Reddy. 2022. Effect of Alkalization on characterization of Ripe Bulrush (Typha Domingensis) grass fiber reinforced epoxy composites. Journal of Natural Fibers 19 (3):931–42. doi:10.1080/15440478.2020.1764443.
- Ramesh, M., R. Logesh, M. Manikandan, N. S. Kumar, and D. V. Pratap. 2017. Mechanical and water intake properties of banana-carbon hybrid fiber reinforced polymer composites. Materials Research 20 (2):365–76. doi:10.1590/1980-5373-mr-2016-0760.
- Rangappa, S. M., S. Siengchin, J. Parameswaranpillai, M. Jawaid, and T. Ozbakkaloglu. 2022. Lignocellulosic fiber reinforced composites: Progress, performance, properties, applications, and future perspectives. Polymer Composites 43 (2):645–91. doi:10.1002/pc.26413.
- RaviKumar, P., G. Rajeshkumar, J. Prakash Maran, N. A. Al-Dhabi, and P. Karuppiah. 2021. Evaluation of mechanical and water absorption behaviors of jute/carbon fiber reinforced polyester hybrid composites. Journal of Natural Fibers 19 (13):6521–33. doi:10.1080/15440478.2021.1924339.
- Reddy, P. V., D. Mohana Krishnudu, P. Rajendra Prasad, and V. S. R. R. 2021. A study on alkali treatment influence on Prosopis juliflora fiber-reinforced epoxy composites. Journal of Natural Fibers 18 (8):1094–106. doi:10.1080/15440478.2019.1687063.
- Reddy, R. S., D. Mohana Krishnudu, P. Rajendra Prasad, and P. V. Reddy. 2020. Alkali treatment influence on characterization of Setaria italica (Foxtail Millet) fiber reinforced polymer composites using vacuum bagging. Journal of Natural Fibers 19 (5):1851–63. doi:10.1080/15440478.2020.1788494.
- Reddy, B. M., Y. V. Mohana Reddy, B. C. Mohan Reddy, and R. M. Reddy. 2020. Mechanical, morphological, and thermogravimetric analysis of alkali-treated Cordia-Dichotoma natural fiber composites. Journal of Natural Fibers 17 (5):759–68. doi:10.1080/15440478.2018.1534183.
- Rizal, S., D. A. Gopakumar, S. Huzni, S. Thalib, H. P. S. Abdul Khalil, and H. Abdul Khalil. 2018. Interfacial compatibility evaluation on the fiber treatment in the Typha fiber reinforced epoxy composites and their effect on the chemical and mechanical properties. Polymers 10 (12):1316. doi:10.3390/polym10121316.
- Sarasini, F., S. D. J, C. Valente, T. Santulli, L. D. Touchard, F. Mellier, L. Lampani, P. Gaudenzi, L. Lampani, and P. Gaudenzi. 2016. Damage tolerance of carbon/flax hybrid composites subjected to low velocity impact. Composites Part B: Engineering 91:144–53. doi:10.1016/j.compositesb.2016.01.050.
- Sarasini, F., J. Tirillò, L. Ferrante, M. Valente, T. Valente, L. Lampani, P. Gaudenzi, S. Cioffi, S. Iannace, and L. Sorrentino. 2014. Drop-weight impact behaviour of woven hybrid basalt–carbon/epoxy composites. Composites Part B: Engineering 59:204–20. doi:10.1016/j.compositesb.2013.12.006.
- Saravanakumar, S. S., A. Kumaravel, T. Nagarajan, and I. Ganesh Moorthy. 2014. Investigation of physico-chemical properties of alkali-treated Prosopisjuliflorafibers. International Journal of Polymer Analysis and Characterization 19 (4):309–17. doi:10.1080/1023666X.2014.902527.
- Saravanakumar, S. S., A. Kumaravel, T. Nagarajan, P. Sudhakar, and R. Baskaran. 2013. Characterization of a novel natural cellulosic fiber from Prosopis juliflora bark. Carbohydrate Polymers 92 (2):1928–33. doi:10.1016/j.carbpol.2012.11.064.
- Senthamaraikannan, P., S. S. Saravanakumar, V. P. Arthanarieswaran, and P. Sugumaran. 2016. Physico-chemical properties of new cellulosic fibers from the bark of Acacia planifrons. International Journal of Polymer Analysis and Characterization 21 (3):207–13. doi:10.1080/1023666X.2016.1133138.
- Shanmugam, D., and M. Thiruchitrambalam. 2013. Static and dynamic mechanical properties of alkali treated unidirectional continuous Palmyra Palm Leaf Stalk Fiber/jute fiber reinforced hybrid polyester composites. Materials & Design 50:533–42. doi:10.1016/j.matdes.2013.03.048.
- Sivaraman, B., N. Radhika, A. Das, G. Gopakumar, L. Majumdar, S. K. Chakrabarti, K. P. Subramanian, B. N. Raja Sekhar, and M. Hada. 2015. Infrared spectra and chemical abundance of methyl propionate in icy astrochemical conditions. Monthly Notices of the Royal Astronomical Society 448 (2):1372–77. doi:10.1093/mnras/stu2602.
- TG, Y. G., S. Jawaid, S. Siengchin, M. Mavinkere Rangappa, S. Siengchin, and M. Jawaid. 2022. Mechanical and thermal properties of flax/carbon/kevlar based epoxy hybrid composites. Polymer Composites 43 (8):5649–62. doi:10.1002/pc.26880.
- TG, Y. G., V. Siengchin, S. MR, S. Kushvaha, M. R. Sanjay, and S. Siengchin. 2021. A new study on flax-basalt-carbon fiber reinforced epoxy/ bioepoxy hybrid composites. Polymer Composites 42 (4):1891–900. doi:10.1002/pc.25944.
- Tran, T. P. T., J. C. Bénézet, and A. Bergeret. 2014. Rice and Einkorn wheat husks reinforced poly (lactic acid)(PLA) biocomposites: Effects of alkaline and silane surface treatments of husks. Industrial Crops and Products 58:111–24. doi:10.1016/j.indcrop.2014.04.012.
- Tranchard, P., S. Duquesne, F. Samyn, B. Estebe, and S. Bourbigot. 2017. Kinetic analysis of the thermal decomposition of a carbon fibre-reinforced epoxy resin laminate. Journal of Analytical and Applied Pyrolysis 126:14–21. doi:10.1016/j.jaap.2017.07.002.
- Venkatasudhahar, M., P. Kishorekumar, and N. Dilip Raja. 2020. Influence of stacking sequence and fiber treatment on mechanical properties of carbon-jute-banana reinforced epoxy hybrid composites. International Journal of Polymer Analysis and Characterization 25 (4):238–51. doi:10.1080/1023666X.2020.1781481.
- Vijay, R., A. Vinod, D. L. Singaravelu, M. R. Sanjay, and S. Siengchin. 2021. Characterization of chemical treated and untreated natural fibers from Pennisetum orientale grass-A potential reinforcement for lightweight polymeric applications. International Journal of Lightweight Materials and Manufacture 4 (1):43–49. doi:10.1016/j.ijlmm.2020.06.008.
- Vinod, A., J. Tengsuthiwat, Y. Gowda, R. Vijay, M. R. Sanjay, S. Siengchin, and H. N. Dhakal. 2022. Jute/Hemp bio-epoxy hybrid bio-composites: Influence of stacking sequence on adhesion of fiber-matrix. International Journal of Adhesion and Adhesives 113:103050. doi:10.1016/j.ijadhadh.2021.103050.
- Yap, M. G. S., Y. T. Que, and L. H. L. Chia. 1991. FTIR characterization of tropical wood–polymer composites. Journal of Applied Polymer Science 43 (11):2083–90. doi:10.1002/app.1991.070431115.
- Yashas Gowda, T. G., A. Vinod, P. Madhu, M. R. Sanjay, S. Siengchin, and M. Jawaid. 2022. Areca/Synthetic fibers reinforced based epoxy hybrid composites for semi‐structural applications. Polymer Composites 43 (8):5222–34. doi:10.1002/pc.26814.
- Yatim, N. M., Z. Shamsudin, A. Shaaban, N. A. Sani, R. Jumaidin, and E. A. Shariff. 2020. Thermal analysis of carbon fibre reinforced polymer decomposition. Materials Research Express 7 (1):015615. doi:10.1088/2053-1591/ab688f.