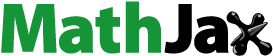
ABSTRACT
Using chitosan as pretreatment agent, the dyeing properties of peanut skin extracts (PSE) to flax fabrics were improved. Flax fabrics have excellent comfort property and are loved by many people. However, flax is different to dye, especially with natural dye. In this research, chitosan was used to pretreat flax fabrics and PSE was used to dye the pretreated flax fabric. The optimal conditions of the chitosan pretreatment and dyeing conditions of PSE were investigated according to the K/S value of the dyed flax fabrics. Comparing the original flax fabric, the chitosan pretreated flax fabric significantly improved the absorption to PSE, and the dyed fabrics had an increased K/S value from 0.9 to 3.9, and had good color fastnesses. The main chemical constitution in PSE such as catechin, chitosan, and cellulose of flax fabrics may form hydrogen bond and ionic bond. Chitosan pretreated and dyed flax fabrics had a stronger ability to UV resistance and scavenging free radicals. The fluorescence intensity of the dyed flax fabrics was improved while it was decreased after chitosan pretreatment. Chitosan pretreatment combined PSE dyeing can be used to develop functional flax textiles without using metallic mordants.
摘要
以壳聚糖为预处理剂,改善了花生皮提取物对亚麻织物的染色性能. 亚麻织物具有优异的舒适性,受到许多人的喜爱. 然而,亚麻与染料不同,尤其是与天然染料不同. 本研究采用壳聚糖对亚麻织物进行预处理,PSE对预处理后的亚麻织物染色. 根据染色亚麻织物的K/S值,研究了壳聚糖预处理和PSE染色的最佳条件. 与原亚麻织物相比,壳聚糖预处理的亚麻织物显著提高了对PSE的吸收,染色织物的K/S值从0.9提高到3.9,具有良好的牢度. PSE中的主要化学成分如儿茶素、壳聚糖和亚麻织物的纤维素可以形成氢键和离子键. 壳聚糖预处理和染色亚麻织物具有较强的抗紫外线和清除自由基的能力. 壳聚糖预处理后亚麻织物的荧光强度有所提高,但有所降低. 壳聚糖预处理结合PSE染色可以在不使用金属媒染剂的情况下开发功能性亚麻纺织品.
Introduction
At present, extracting natural functional dyes from agricultural by-products and applying them in textile dyeing has become an important subject (Pandit, Jose, and Pandey Citation2020; Rather et al. Citation2021). Rizal et al. (Citation2021) reviewed that the isolation technique and their applications of the processed cotton fibers. It was also reported that the seaweed-neem bio-composite film showed multi-functional properties and could be used as an excellent sustainable packaging material (Oyekanmi et al. Citation2021). According to the United States Department of Agriculture, the current peanut global production is 42.29 million tons and China is a major producer, followed by India, Nigeria and the United States (Do Valle Calomeni et al. Citation2017). As the by-product of peanut production, 740,000 tons of peanut skin (PS) are produced every year in world, a small part of which is usually used as animal feed and most of them are discarded (Putra et al. Citation2018). It was reported that PS extracts (PSE) have the functionalities of anti-oxidation, anti-aging, antivirus, scavenging free radicals, inhibiting tumors, hemostasis and detumescence (Wang et al. Citation2015; Zhang, Jin, and Wang Citation2005). Therefore, it is necessary to study the utilization of PSE with the high added value. PS is red-brown film and had moisture content of 10%, ash content of 2.1%, fat content of 10%, protein content of 11%, dietary fiber of 40%, tannin and a variety of colorants (Muñoz-Arrieta et al. Citation2021). PS colorants are soluble in water, and its extraction rate can be as high as 33.25% and its major compounds was the proanthocyanins followed by other flavonoids, such as catechin (Munekata et al. Citation2016). PSE has good dyeing performance to protein (Rather et al. Citation2021) and starch (Wen and Jiang Citation2009). At present, PSE are mainly used as food additives (Do Valle Calomeni et al. Citation2017; Rossi et al. Citation2020; Toomer Citation2020). Some scholars have reported that PSE combined with polysaccharides could prepare active biodegradable film (Ju and Song Citation2020; Meng et al. Citation2020). Some peoples studied its application in medical drugs (Christman et al. Citation2019).
PSE were also studied to dye cotton, wool and silk fabrics (Pandey et al. Citation2018; Rather et al. Citation2021), but the dyeing to flax has not been reported. Flax fabrics has a series of excellent characteristics, such as anti-static, anti-bacterial and deodorant, high moisture absorption, comfortable to wear, therefore flax fibers are called “fiber queen” and flax fabrics are loved by many people (Jiang et al. Citation2016). Therefore, the application of PSE in dyeing flax fabrics not only increase the economic benefits but also protect environmental benefit. However, flax fiber has dyeing problems such as the poor affinity to some natural dyes (Teli and Pandit Citation2017) and the poor color fastnesses. Particularly, natural dyes are mostly anionic and have poor affinity to cellulose fibers, resulting in low dyeing rate, poor color fastness. Therefore, it is necessary to pretreat flax fabrics to improve the dyeing performance of PSE.
Chitosan can be obtained by deacetylation to natural chitin biopolymer. Chitosan is nontoxic, degradable (Anitha et al. Citation2014; Morgado et al. Citation2014) and antibacterial (Wang et al. Citation2020; Yang et al. Citation2011). Shahid-ul-Islam et al (Shahid Ul and Butola Citation2019) reported that the pretreated flax fabrics with chitosan improved the dyeing property of aqueous pineapple extracts and the antioxidant activity of the chitosan coated and dyed flax was much higher. Some scholars (Bhuiyan et al. Citation2017) reported that the chitosan treatment could improve the dyeing and antimicrobial properties of the natural dye henna on jute fibers. Rym Mansour & Hayet Ben Ali (Mansour and Ben Ali Citation2019) reported that the depth of shade (K/S = 11) of the dyed cotton fabrics with Roselle after chitosan treatment were higher than that of the untreated cotton fabrics (K/S = 4). Therefore, chitosan can be expected to act as a bridge between PSE and flax fabrics, improving the dyeing properties of the fabrics and endowing them with strong functional properties.
In order to obtain the functional flax fabrics with high added value using green method, the flax fabric was pretreated with chitosan to improving the dyeing properties of PSE to flax fabrics in this study and the functional properties of the dyed fabrics were analyzed.
Experimental
Materials and chemicals
PS was purchased from Nanjing, Jiangsu Province, China; Flax fabrics were from Yixing Sunshine Linen textile Co., Ltd, Jiangsu, China and had the following characteristics: Plain weave, the fineness of warp and weft both 28 × 2 tex, warp density 205 threads per 10 cm, weft density 228 threads per 10 cm. Chitosan, a degree of deacetylation of > 90%, and a viscosity of about 1000 mPa·s (1% solution in 1% acetic acid at 25°C), was purchased from Hentai County Jinhu Shell Products Co., Ltd.
Anhydrous ethanol with a content of 99.5%, acetic acid with a content of 99.9%, methanol with a content of 99.8%, sodium hydroxide, citric acid, FeSO4·7 H2O and KAl(SO4)2·12 H2O are of analytical grade and were purchased from Sinopharm Group. Soap tablets in the washing fastness experiment were purchased from China Textile Science Research Institute. 1,1-diphenyl-2-trinitrophenylhydrazine (DPPH) with a purity of 97% was purchased from Beijing Solarbio Life Science Ltd, China.
Chitosan pretreatment and dyeing
Chitosan pretreatment
Chitosan pretreatment solution was prepared by dissolving chitosan (1–5% w/w) in 2% (v/v) acetic acid aqueous solution and magnetic stirring for 30 min at 50–90°C in water bath. The flax fabric was added into the chitosan pretreatment solution at a fabric-to-liquor ratio of 1:30 and treated in water bath for 30 min at 80°C. The treated flax fabric was padded with 2 dips and 2 nips to obtain a pickup of 100%. The flax fabric was then placed at room temperature for 12 h.
Preparation of PSE
PS was powdered with a disintegrator (Taisite company, China). The particle size distribution of the powdered PS was about 100–150 μm. According to our previous research, the extracted liquid with 60% (w/w) ethanol/water mixed solvent showed the highest color concentration. PS powder was added to 60% ethanol/water solution according to the ratio of material to liquid 1:30 and magnetic stirred for 60 min at 40°C, then the supernatant was filtered by medium speed quantitative filter paper. The supernatant was concentrated and heated to dry at 85°C, and PSE powder was obtained after cooling.
Dyeing methods and conditions
Dyeing conditions of PSE to flax fabrics were as follows: the dosage of PSE powder was 6% of weight of fabric (OWF), fabric-to-liquor ratio of 1:20, different dyeing pH was 5–11 for direct dyeing and 3–11 for chitosan pretreating and dyeing, different dyeing temperature was 80–120°C. The IR DYER dyeing machine (Xiamen Ruibi Precision Machinery Co., Ltd) was used for dyeing for 50 min. After the dyeing process, the dyed fabrics were thoroughly washed with water and dried at room temperature for further characterizations.
As control, pre-mordant dyeing methods with metallic mordants Fe2+ and Al3+ were also used to dye flax fabrics with PSE powder according to the previous experiment. For pre-mordant dyeing methods, the dosage of the mordants FeSO4·7 H2O and KAl(SO4)2·12 H2O were 3% and 6% OWF respectively. The fabrics were mordanted at 60°C for 45 min with a bath ratio of 1:25. The direct dyeing method, that is, the flax fabrics was directly dyed without any pretreatment, was also used as control. After pre-mordanting, the flax fabrics were dyed with PSE powder with the above mentioned dyeing conditions.
Characterizations of the structures of flax fabrics after chitosan pretreatment and dyeing with PSE
A HITACHI SU1510 scanning electron microscope (SEM, Japan) was used to observe the morphologies of the flax fabrics. The samples were observed under an accelerating voltage of 5kV. Sigma HD field emission electron microscope (Zeiss, Germany), Table top EDS analysis and mapping were used to testing the elemental composition of the pretreated flax fabric. FTIR spectra of PSE powder and the flax fabrics before and after dyeing were measured with a FTIR spectrophotometer (Nicolet iS10, USA). FTIR spectra was recorded from 4000 to 500 cm−1 at a resolution of 4 cm−1 using attenuated total reflection method.
UV-Vis absorbance spectra of the extracted solution from PS and the color and colorfastness of the dyed fabrics
The UV-Vis spectrophotometer (TU-1901, Purkinje General Instrument Co. Ltd., Beijing, China) were used to measure the UV-Vis absorbance spectra of the extracted solution from PS. ColorEye7000A spectrophotometer (Gemini BV, Gretag Macbeth Company) were used to measure the color depth (K/S) and characteristic values (L*, a*, b*) of color of the dyed flax fabrics using an illuminant D65 and 10 standard observers.
According to the standard methods (Hou et al. Citation2013, Citation2017), the color and colorfastness of the dyed flax fabrics were evaluated. Washing Colorfastness was evaluated according to ISO105-C10:2007. The dyed flax fabrics were attached between flax and wool fabrics and were then agitated at 40°C for 30 min using SW-12AII washing fastness testing machine (Wenzhou, China). Color changes of the flax fabrics and the extent of stain on the adjacent fabrics were determined by comparing with the original fabric. Colorfastness to rubbing was evaluated following ISO105-X12:2002. The dyed flax fabrics were rubbed 10 times with a dry and wet flax cloth on the crock meter. The staining of the flax fabrics was then determined according to the gray card. Atlas 150S lightfastness device (ATLAS Ltd., German) equipped with a xenon arc lamp was used to evaluate the light-fastness rating. The dyed flax fabrics and the blue wool reference materials were exposed for 9 or 20 h at the power of 2000 w.
Characterization of mechanical properties of the chitosan pretreated and dyed flax fabrics
The mechanical properties of the flax fabrics obtained after different treatment were tested according to the standard GB/T3923.1–2013 “Tensile properties of textile fabrics, Part 1: Determination of breaking strength and elongation (strip method).” The length of the sample is 200 mm and the width is 50 mm, the tensile speed is 100 mm/min, and the sample was pre-stretched by a force of 1 N. Five samples for every fabric after treatment under different conditions are tested to calculate the average value.
Characterizations of UV protection property of the flax fabrics dyed with PSE
The UV-protection properties of the fabrics were measured according to Australian/New Zealand standard AS/NZS 4399. Using Varian Cary 50 fabric UV protection measurement system (Agilent Technologies Ltd.), the UV-Protection Factor (UPF) and ultraviolet transmittance rates TUVA, TUVB were recorded. TUVA is the transmittance rate of UVA at wavelength between 315 nm and 380 nm; TUVB is the transmittance rate of UVB at wavelength between 280 nm and 315 nm. Lower ultraviolet transmittance rate and higher UPF value means better the UV protection property of the fabric.
Characterizations of fluorescence properties
The fluorescent properties of the yarns in flax fabrics were measured using an inverted fluorescence microscope Ti-S (Nikon Eclipse Inc., Japan) with a high-pressure mercury lamp as excitation source. Green excitation filter of 510–560 nm were used. All fluorescent images were taken in the dark.
Characterizations of radical scavenging activity
The antioxidant intensities were evaluated using DPPH free radical scavenging assay according to the method by (Rather et al. Citation2017). 2.5 cm2 of untreated flax as control sample, directly dyed flax, chitosan-pretreated flax and chitosan-pretreated dyed flax were mixed with 40 ml of 0.15 mM methanolic solution of DPPH and then were incubated in the dark for 30 min at room temperature. The hydrogen donors in sample produced stable non radical form of DPPH, indicated by color change from violet to pale yellow. The absorbance at 517 nm was measured with a UV-spectrophotometer. DPPH radical scavenging activity was calculated using the Equationequation (1)(1)
(1) (Shahid Ul and Butola Citation2019).
Here, g and h represent the absorbance at 517 nm of the control sample and the treated sample respectively.
Results and discussion
Effect of chitosan pretreatment conditions on the dyeing properties of PSE to flax fabric
Chitosan dosage
As shown in , the K/S value of dyed flax fabric increased with the increase of the amount of chitosan. When the amount of chitosan increased to 1%, the increase rate of the K/S value of the dyed flax fabric was fastest and its K/S value reached the maximum when the amount of chitosan increased to 5%, indicating that the adsorption of chitosan on the flax fabrics had reached saturation. Chitosan is a nitrogen-containing cationic polymer, which has a good affinity with cellulose fiber. It can dissolve into the interior of cellulose and combine with cellulose by hydrogen bonds. After chitosan modification, the surface of the flax fabric is is introduced with negative charge -NH3+, which is mutually attracted with the negatively charged PSE (Zhang, Cui, and Sui Citation2008). Therefore, as the amount of chitosan increased, the surface of flax fabric increased -NH3+, the fabric could absorb more PSE, and the K/S value of dyed fabric also increased. When the amount of chitosan reaches to 5%, the -NH3+ on the surface of the fabric reaches saturation. When the amount of chitosan continues to increase, the amount of -NH3+ on the fabric hardly increased and the amount of dye adsorbed on the fabric hardly increased, and it was observed that the levelness of the fabric was better under the 5% amount of chitosan. Therefore, the optimal amount of chitosan is 5%.
Pretreatment temperature
As shown in , with the increase of pretreatment temperature, the K/S value of the dyed flax fabric first increased and then decreased, and when the pretreatment temperature was 70°C, the K/S value of dyed flax fabric reached the maximum. When the pretreatment temperature is less than 70°C, the increase of the pretreatment temperature is conducive to the dissolution of chitosan, and the kinetic energy of chitosan molecules increases. The intense motion could make the chitosan molecules combine with the flax fabric better, which is conducive to the dyeing of PSE. When the temperature was higher than 70°C, more chitosan molecules existed in the solution, and the K/S value of the dyed fabric decreased (Liu et al. Citation2019). Therefore, the optimal pretreatment temperature is 70°C.
Analysis of the interaction of cellulose-chitosan-PSE
Change of the structures of the flax fabrics after chitosan pretreatment and dyeing with PSE
shows the surface morphologies of the untreated and chitosan pretreated flax fabrics by SEM. It can be seen that the untreated flax fabric has larger gaps between the yarns and the fabric was loose, while the chitosan pretreated flax fabric has smaller gaps between the yarns and the fabric was more compact. There are two reasons for the changes, on one hand, the flax fibers expanded in radial direction under water and heat conditions during the pretreatment and dyeing process. On the other hand, the chitosan got into the crevices of the pretreated flax fabric. Observing the flax fiber surface morphology under high magnification, it can be seen that the untreated flax surface was smooth and clean, whereas the treated flax surface was rough, which was consistent with the literature (Muñoz-Arrieta et al. Citation2021). The surface of the chitosan pretreated and dyed flax fabrics was rougher, which proved that the chitosan was successfully adsorbed on the fabric and the dye was adsorbed on the pretreated fabric. EDS mapping and spectrum of the chitosan pretreated flax fabric in shows nitrogen elements of chitosan uniformly presented the on the chitosan pretreated flax fabric. SEM and EDS results indicated that chitosan successfully permeated into the fabric, and the results were consistent with the conclusions in the literature (Ibrahim, El-Sayed, and Ameen Citation2010; Shahid Ul and Butola Citation2019).
Analysis of FTIR spectra of chitosan pretreated and dyed flax fabric
FTIR spectra of PSE, the untreated flax fabric and the chitosan pretreated or PSE dyed flax fabrics are shown in . From , PSE presented absorption peaks at 3299, 2920, 2850, 1604 and 1443 cm−1. The broad band at 3299 cm−1 was assigned as an O-H stretching vibration in phenolic component, whereas the peak at 2920 cm−1 corresponded to C-H stretching vibration. The absorption peak at 1604 and 1443 cm−1 correlated with the C=C stretching and the O-H bending in phenyl or tannin respectively. The presence of phenolic components in PSE were proved by the above-mentioned peaks. From , chitosan pretreated fabric, chitosan pretreated and dyed flax fabric both presented a new absorption peak at 1540 cm−1, which was assigned as an N-H stretching in chitosan molecular compared to the original flax fabrics and presented chitosan at flax fabric surface.
Interaction between cellulose, chitosan and catechin molecule in PSE
shows the possible interaction between cellulose, chitosan and catechin in PSE. Chemical constituents presented in PSE have polar hydroxyl groups which can form ionic interactions with protonated amine groups of chitosan on the pretreated flax fabrics (Shahid Ul and Butola Citation2019). Chemical constituents such as catechin in PSE have more polar hydroxyl groups (Larrauri et al. Citation2016). These hydroxyl groups are involved in the action of amino and hydroxyl ions in chitosan treated flax fabric and the flax fabric can absorb more PSE dyes by the combination of hydrogen and ionic bonds.
Effect of dyeing conditions on the color characteristics of the chitosan pretreated and dyed flax fabrics
Dyeing pH
and show the effect of the dyeing pH value on the K/S value and the color characteristic values of the flax fabrics dyed at different pH values with PSE, respectively. In and , it can be seen that with the increase of pH value, the K/S value of the fabric decreased first and then increased. At pH = 3 and pH = 11, the higher K/S values appeared. After the chitosan pretreatment (Liu et al. Citation2021), the positive charges were introduced into the surface of flax fabric under acid conditions. There is a lot of hydroxyl anion in the PSE and there are more positive amino ions on chitosan macromolecule in acid solution, resulting in the forming of more ionic bonds between the flax and the PSE. Moreover, the increase of pH value of dyeing solution led to the increase of negative charge on the surface of flax fiber and the increase of repulsive force between PSE and the flax (Ke, Zhu, and Chowdhury Citation2021). Under the pH value was less than 7, as the pH value increased, the amino group on the fabric decreased, leading to the decrease of the ionic bond and the K/S values of the dyed fabrics. However, when the pH value was greater than 9, the K/S value of the dyed fabric increased quickly. The higher K/S values may resulted from that the chemical changes in PSE compositions had occurred under higher alkaline conditions (Zhong, Wu, and Feng Citation2010).
Table 1. The color characteristic values of the dyed flax fabrics at different pH values (λ = 400 nm).
shows the UV-Vis absorption spectra of PSE under different pH conditions. From , it can be seen that the visible absorption spectrum curves of PSE solution are consistent when the pH value is lower than 7. For the pH values of 9 and 11, the absorption peaks of the spectrum curves were higher and obviously changed. From , the color depth of PSE solution gradually increased with the increase of pH value. Especially under alkaline conditions, the color of PSE solution has changed from light brown to dark brown, which is consistent with the higher K/S values in . Since the predominant colorants in PSE were phenolic compounds, more phenolic hydroxyl groups of PSE could dissociate into RO- anions at higher pH than 9.
Figure 8. (A) the UV-vis absorption curves and (b) the images of the extracted liquid from PS with different pH value; (c) Images of the dyed flax fabrics with PSE at different dyeing pH.

As shown in , it can be seen that the color depth of the chitosan pretreated and dyed fabrics were significantly increased compared with those of direct dyeing under the same pH value, and even was deeper than those of Al3+ pre-mordant dyeing. The color of the chitosan pretreated and dyed flax fabric was consistent with the K/S values as shown in . It can be seen that the color of the dyed fabric changed significantly from the yellow-brown to reddish-brown and the color depth increased under alkaline conditions, especially at pH value of 11. However, the color of the dyed fabric was uneven at dyeing pH value of 11, which might resulting from that chitosan could not uniformly distribute on the fabric surface.
Dyeing temperature
and shows the effects of the dyeing temperature and chitosan pretreatment on the K/S value and the color characteristic values of the dyed flax fabrics with PSE respectively. From and , with the dyeing temperature increase, the K/S value of the dyed fabrics increased for both dyeing methods. When the temperature was above 100°C, the K/S values of the dyed fabrics significantly increased as the increase of the dyeing temperature. This maybe resulted from the increased accessible surface in the flax fibers by PSE, which was beneficial to the bonding of the PSE with the flax. At the same dyeing temperature, the K/S values of the chitosan pretreated and dyed fabrics were all higher than those of the fabrics by direct dyeing method, and the differences of K/S values at lower temperature between both dyeing methods were more obvious. With the increase of the dyeing temperature, the K/S values for both dyeing methods gradually approached, which resulting from that the more chitosan molecules on the pretreated fabric entered into the dye solution and the chitosan molecules on the surface of the fabric decreased at high temperature.
Figure 9. (A) Effects of the dyeing temperature and chitosan pretreatment on the K/S values of dyed flax fabrics with PSE; (b) Images of the dyed flax fabrics with PSE at different dyeing temperature.

Table 2. The color characteristic values of the flax fabrics dyed at different temperatures (λ = 400 nm).
shows the dyed flax fabrics with PSE under different dyeing temperatures and from and it can be seen that the color of the dyed fabric with chitosan pretreatment gradually deepened with the increase of the dyeing temperature, changing from light brown to dark brown. Its hue is similar to that of direct dyeing. Under the same dyeing temperature, the color of the dyed fabric with chitosan pretreatment is darker than that of direct dyeing.
The mechanical properties of the flax fabrics before and after dyeing at the different temperatures are shown in . From , the dyed flax fabric at Dyeing Temperature of 100°C retained about 88% of the tensile breaking strength of that of the original fabric. The dyed flax fabric at dyeing temperature of 110°C or 120°C retained about 79% of the tensile breaking strength of that of the original fabric. The Breaking elongation of the dyed flax fabrics at different dyeing temperature of 100°C, 110°C and 120°C were about twice as much as that of the original flax fabric. The higher breaking elongation could resulted in the better contact comfort. Therefore, the mechanical properties of the dyed flax fabric could meet the requirement of clothing fabrics.
Table 3. The mechanical properties of the flax fabrics before and after dyeing at the different temperatures.
The following color fastnesses and functional properties were carried out on the fabrics dyed under the following conditions: the chitosan pretreatment temperature of 70°C, the amount of chitosan of 5% OWF, the dyeing pH value of 5, the dyeing temperature of 120°C and the PSE content of 6% OWF.
Color fastnesses of the chitosan pretreated and dyed flax fabrics
shows the color fastness of the flax fabrics dyed with PSE with by different methods. The color fastnesses of the flax fabrics dyed with PSE all reaches above Grade 3. The good dyeing performance of PSE may be due to the existence of tannin content (Pandey et al. Citation2018). The color fastness of the dyed fabric with chitosan pretreatment are the same with those of the fabric dyed by Al3+ pre-mordant dyeing. Its wet rubbing fastness is little inferior than that of Fe2+ pre-mordant dyeing. Chitosan pretreatment and PSE dyeing can obtain the bright-colored flax fabrics with satisfied color fastnesses.
Table 4. Fastness properties of the dyed flax fabrics with PSE by different method.
Functionality of the flax fabrics pretreated with chitosan and dyed with PSE
UV protection properties
shows the UV protection values of the fabrics dyed by different dyeing methods. The TUVA and TUVB of the dyed flax fabric are lower than that of the original fabric, which indicates that the dyed flax fabric has better UV protection. This UV protection property is mainly caused by the special chemical composition in PSE. The UV protection property of PSE may be a synergistic effect of many components of the PSE such as saponins, tannins, and polyphenols (Anitha Citation2012). According to the literature, the saponins, tannins and polyphenols in PSE play the main role for the ability to UV protection (Shahid et al. Citation2012). The UV protection of chitosan pretreated and dyed flax fabric is significantly higher than that of the untreated flax fabric. On the one hand, chitosan pretreatment can promote the adsorption of PSE on the surface of flax fabric, so as to improve its UV resistance; On the other hand, the chitosan itself covered the fabric, the interspace of the yarns in fabric decreased, which also could improve the UV protection performance.
Table 5. Ultraviolet protection properties of the dyed fabrics with PSE by different dyeing method.
Fluorescence properties
shows fluorescence properties of the flax yarns taken from the original and dyed flax fabrics with different dyeing methods. The flax yarn from the fabric before and after chitosan treatment had little fluorescence property, while the yarns from the dyed fabrics had strong fluorescence property, indicating that the fluorescent substance was contained in the PSE. Because of the anthocyanins, quercetin, catechin, flavonoids and other substances contained in the PSE, the benzene ring contains more conjugated C=C double bonds, which absorb light energy into the excited state and can return to the ground state in a relatively short time, thus emitting light with wavelength longer than the incident light and producing fluorescence effect. The fluorescence intensity of the dyed flax fabric with chitosan pretreatment is nearly 1/3 of that of the dyed flax fabric without chitosan pretreatment. After the chitosan treatment, the bonding between PSE and chitosan has negative effect to fluorescence performance.
Radical scavenging activity
shows that the chitosan pretreated and dyed flax fabric has highest ability to scavenging free radicals of 77.01%. PSE contains a large number of phenolic substances and flavonoids. It was reported that the content of total phenols in extracts had a close relationship with the free radical-scavenging activity (Elsorady and Ali Citation2018). The antioxidant capacity of the dyed flax fabric was greatly improved. The antioxidant capacity of chitosan pretreated flax fabric was 34.77 higher than that of original flax fabric. Chitosan could be applied to skin protectant textiles (Anitha et al. Citation2014). The highest free radical scavenging activity for the chitosan pretreated and dyed flax fabrics shows that the chitosan pretreatment combining dyeing with PSE have the positive effect to the antioxidant activity.
Table 6. Radical scavenging activity of the flax fabrics after different treatment methods.
Conclusions
Chitosan pretreatment can improve the dyeing properties of PSE to flax fabrics and the functional properties of the dyed flax fabrics. Chitosan can form hydrogen bond and ionic bond with catechin in PSE and cellulose. Under the chitosan pretreatment temperature of 70°C, the chitosan dosage of 5% OWF, the dyeing temperature of 120°C and the PSE amount of 6% OWF, the dyed flax fabric has the highest K/S value of 3.9 and its color fastnesses are above grade 4, which are similar with those of the dyed fabrics by the Al3+ pre-mordant dyeing and Fe2+ pre-mordant dyeing methods. The main chemical constitution in PSE such as catechin, chitosan, and cellulose of flax fabrics may form hydrogen bond and ionic bond. Chitosan pretreated and dyed flax fabrics had a stronger ability to UV resistance and scavenging free radicals. The fluorescence intensity of the dyed flax fabrics was improved while it was decreased after chitosan pretreatment. Chitosan pretreatment combining PSE dyeing is a potential eco-friendly method to prepare colored and functional flax fabrics for developing health care textiles with high added value. Using PSE in dyeing flax fabrics will show economic and environmental benefits.
Highlights
Chitosan pretreatment to flax fabric can improve the dyeing properties of PSE.
Chitosan can form hydrogen bond and ionic bond with catechin in PSE and cellulose.
Chitosan pretreated and dyed flax fabric has higher K/S value and color fastnesses.
The dyed flax fabric has higher UV protection and DPPH free radical scavenging.
Chitosan pretreatment and PSE dyeing is a green method to prepare health textiles.
Author’s contribution
This work was completed by the contribution of the authors: Qianqian Liu: Methodology, Software, Writing – Original Draft. Yu Zhang: Data Curation, Formal analysis. Zixiang Ma: Resources, Validation. Yingkun Wang: Visualization; Formal analysis; Yue Tian: Data Curation; Xu Helan : Investigation; Xiuliang Hou: Supervision, Conceptualization, Methodology, Writing-Review & Editing.
Acknowledgments
This work was supported by the Postgraduate Research & Practice Innovation Program of Jiangsu Province (KYCX20_1794).
Disclosure statement
No potential conflict of interest was reported by the author(s).
Additional information
Funding
References
- Anitha, A., S. Sowmya, P. T. S. Kumar, S. Deepthi, K. P. Chennazhi, H. Ehrlich, M. Tsurkan, and R. Jayakumar. 2014. Chitin and chitosan in selected biomedical applications. Progress in Polymer Science 39 (9):1644–17. doi:10.1016/j.progpolymsci.2014.02.008.
- Anitha, T. 2012. Medicinal plants used in skin protection. Asian Journal of Pharmaceutical and Clinical Research 5 (Suppl 3):40–43.
- Bhuiyan, M. A. R., A. Islam, S. Islam, A. Hossain, and K. Nahar. 2017. Improving dyeability and antibacterial activity of Lawsonia inermis L on jute fabrics by chitosan pretreatment. Textiles and Clothing Sustainability 3 (1):2–10. doi:10.1186/s40689-016-0023-4.
- Christman, L. M., L. L. Dean, J. C. Allen, S. F. Godinez, O. T. Toomer, and G. Pintus. 2019. Peanut skin phenolic extract attenuates hyperglycemic responses in vivo and in vitro. PLoS One 14 (3):1–16. doi:10.1371/journal.pone.0214591.
- Do Valle Calomeni, A., V. B. de Souza, M. T. Fabrício Luiz Tulini, M. Thomazini, L. C. Ostroschi, S. M. de Alencar, A. P. Massarioli, J. C. de Carvalho Balieiro, R. A. de Carvalho, and C. S. Favaro-Trindade. 2017. Characterization of antioxidant and antimicrobial properties of spray-dried extracts from peanut skins. Food and Bioproducts Processing 105:215–23. doi:10.1016/j.fbp.2017.08.001.
- Elsorady, M. E. I., and S. E. Ali. 2018. Antioxidant activity of roasted and unroasted peanut skin extracts. International Food Research Journal 25 (1):43–50.
- Hou, X., X. Chen, Y. Cheng, H. Xu, L. Chen, and Y. Yang. 2013. Dyeing and UV-protection properties of water extracts from orange peel. Journal of Cleaner Production 52:410–19. doi:10.1016/j.jclepro.2013.03.004.
- Hou, X., F. Fang, X. Guo, J. Wizi, B. Ma, Y. Tao, and Y. Yang. 2017. Potential of sorghum husk extracts as a natural functional dye for wool fabrics. ACS Sustainable Chemistry & Engineering 5 (6):4589–97. doi:10.1021/acssuschemeng.6b02969.
- Ibrahim, N. A., W. A. El-Sayed, and N. A. Ameen. 2010. A novel technique to minimise energy and pollution in the dyeing of linen fabric. Coloration Technology 126 (5):289–95. doi:10.1111/j.1478-4408.2010.00263.x.
- Jiang, H., W. Wu, Q. Zhou, and M. Dong. 2016. Reactive dyeing mechanism of flax fabric modified by low molecular weight chitosan quaternary ammonium salts. Journal of Tianjin Polytechnic University 35 (2):40–44.
- Ju, A., and K. B. Song. 2020. Active biodegradable films based on water soluble polysaccharides from white jelly mushroom (Tremella fuciformis) containing roasted peanut skin extract. Food Science & Technology 126:109293–96. doi:10.1016/j.lwt.2020.109293.
- Ke, G., K. Zhu, and M. H. Chowdhury. 2021. Dyeing of cochineal natural dye on cotton fabrics treated with oxidant and chitosan. Journal of Natural Fibers 18 (3):317–29. doi:10.1080/15440478.2019.1623742.
- Larrauri, M., M. P. Zunino, J. A. Zygadlo, N. R. Grosso, and V. Nepote. 2016. Chemical characterization and antioxidant properties of fractions separated from extract of peanut skin derived from different industrial processes. Industrial Crops and Products 94:964–71. doi:10.1016/j.indcrop.2016.09.066.
- Liu, H., L. Jia, G. Shan, N. Xue, M. Zhang, and J. Liu. 2019. Dyeing performance of chitosan treated cotton fabrics using lithospermum dyes. Shanghai Textile Science and Technology 47 (5):16–19.
- Liu, Q., Y. Tian, Z. Qiu, J. Li, M. Wang, and X. Hou. 2021. Dyeing performance of natural peanut skins dyes to linen fabrics. Applied Chemical Industry 10:1–6.
- Mansour, R., and H. Ben Ali. 2019. Investigating the use of chitosan: Toward improving the dyeability of cotton fabrics dyed with Roselle (Hibiscus sabdariffa L.). Journal of Natural Fibers 18 (7):1007–16. doi:10.1080/15440478.2019.1675217.
- Meng, W., J. Shi, X. Zhang, H. Lian, Q. Wang, and Y. Peng. 2020. Effects of peanut shell and skin extracts on the antioxidant ability, physical and structure properties of starch-chitosan active packaging films. International Journal of Biological Macromolecules 152:137–46. doi:10.1016/j.ijbiomac.2020.02.235.
- Morgado, P. I., P. F. Lisboa, M. P. Ribeiro, S. P. Miguel, P. C. Simões, I. J. Correia, and A. Aguiar-Ricardo. 2014. Poly(vinyl alcohol)/chitosan asymmetrical membranes: Highly controlled morphology toward the ideal wound dressing. Journal of Membrane Science 469:262–71. doi:10.1016/j.memsci.2014.06.035.
- Munekata, P. E. S., R. D. P. Paseto Fernandes, M. P. de Melo, M. A. Trindade, and J. M. Lorenzo. 2016. Influence of peanut skin extract on shelf-life of sheep patties. Asian Pacific Journal of Tropical Biomedicine 6 (7):586–96. doi:10.1016/j.apjtb.2016.05.002.
- Muñoz-Arrieta, R., D. Esquivel-Alvarado, E. Alfaro-Viquez, V. Alvarez-Valverde, C. G. Krueger, and J. D. Reed. 2021. Nutritional and bioactive composition of Spanish, Valencia, and Virginia type peanut skins. Journal of Food Composition and Analysis 98:103816–17. doi:10.1016/j.jfca.2021.103816.
- Oyekanmi, A. A., U. S. U. Kumar, N. G. Olaiya, A. A. Rahman, A. A. Amirul, C. K. Nuryawan, A. Abdullah, S. Rizal, and S. Rizal. 2021. Functional properties of antimicrobial neem leaves extract based macroalgae biofilms for potential use as active dry packaging applications. Polymers (Basel) 13 (10):1664. doi:10.3390/polym13101664.
- Pandey, R., S. Patel, P. Pandit, N. Shanmugam, and S. Jose. 2018. Colouration of textiles using roasted peanut skin-an agro processing residue. Journal of Cleaner Production 172:1319–26. doi:10.1016/j.jclepro.2017.10.268.
- Pandit, P., S. Jose, and R. Pandey. 2020. Groundnut testa: An industrial agro-processing residue for the coloring and protective finishing of cotton fabric. Waste and Biomass Valorization 12 (6):3383–94. doi:10.1007/s12649-020-01214-y.
- Putra, N. R., L. N. Yian, H. M. Nasir, Z. Binti Idham, and M. A. C. Yunus. 2018. Effects of process parameters on peanut skins extract and CO2 diffusivity by supercritical fluid extraction. IOP Conference Series: Materials Science & Engineering 334 (1):012057–9. doi:10.1088/1757-899X/334/1/012057.
- Rather, L. J., S. Akhter, R. A. Padder, Q. P. Hassan, M. Hussain, M. A. Khan, and F. Mohammad. 2017. Colorful and semi durable antioxidant finish of woolen yarn with tannin rich extract of Acacia nilotica natural dye. Dyes and Pigments 139:812–19. doi:10.1016/j.dyepig.2017.01.018.
- Rather, L. J., Q. Zhou, A. Ali, Q. M. R. Haque, and Q. Li. 2021. Valorization of agro-industrial waste from peanuts for sustainable natural dye production: Focus on adsorption mechanisms, ultraviolet protection, and antimicrobial properties of dyed wool fabric. ACS Food Science & Technology 1 (3):427–42. doi:10.1021/acsfoodscitech.1c00005.
- Rizal, S., P. S. A. H, A. A. Oyekanmi, O. N. Gideon, C. K. Abdullah, E. B. Yahya, T. Alfatah, F. A. Sabaruddin, and A. A. Rahman. 2021. Cotton wastes functionalized biomaterials from micro to nano: A cleaner approach for a sustainable environmental application. Polymers (Basel) 13 (7):1006. doi:10.3390/polym13071006.
- Rossi, Y. E., L. P. Bohl, N. L. Vanden Braber, M. B. Ballatore, F. M. Escobar, R. Bodoira, D. M. Maestri, C. Porporatto, L. R. Cavaglieri, and M. A. Montenegro. 2020. Polyphenols of peanut (Arachis hypogaea L.) skin as bioprotectors of normal cells. Studies of cytotoxicity, cytoprotection and interaction with ROS. Journal of Functional Foods 67:1–10. doi:10.1016/j.jff.2020.103862.
- Shahid, M., A. Ahmad, M. Yusuf, M. I. Khan, S. A. Khan, N. Manzoor, and F. Mohammad. 2012. Dyeing, fastness and antimicrobial properties of woolen yarns dyed with gallnut (Quercus infectoria Oliv.) extract. Dyes and Pigments 95 (1):53–61. doi:10.1016/j.dyepig.2012.03.029.
- Shahid Ul, I., and B. S. Butola. 2019. Effect of chitosan biological macromolecule on colorimetric analysis and radical scavenging activity of linen using pineapple peel extract biomolecules. International Journal of Biological Macromolecules 124:708–15. doi:10.1016/j.ijbiomac.2018.11.259.
- Teli, M. D., and P. Pandit. 2017. A novel natural source sterculia foetida fruit shell waste as colorant and ultraviolet protection for linen. Journal of Natural Fibers 15 (3):337–43. doi:10.1080/15440478.2017.1328327.
- Toomer, O. T. 2020. A comprehensive review of the value-added uses of peanut (Arachis hypogaea) skins and by-products. Critical Reviews in Food Science and Nutrition 60 (2):341–50. doi:10.1080/10408398.2018.1538101.
- Wang, J., M. Zhang, Q. Huo, S. Liu, and Y. Tan. 2020. Performance and application of chitosan. Journal of Textile Science & Engineering 37 (3):110–16.
- Wang, X., A. Wu, S. Zhang, Y. Wang, J. Liao, and Z. Zhen. 2015. High valued development and utilization of peanut red skin resources. Food Science & Technology 40 (11):235–39.
- Wen, Z., and N. Jiang. 2009. Microwave -assisted extraction of red pigment from peanut skin. China Oils & Fats 34 (4):54–57.
- Yang, D. Z., K. Yu, Y. F. Ai, H. P. Zhen, J. Nie, and J. F. Kennedy. 2011. The mineralization of electrospun chitosan/poly(vinyl alcohol) nanofibrous membranes. Carbohydrate Polymers 84 (3):990–96. doi:10.1016/j.carbpol.2010.12.057.
- Zhang, C., Y. Cui, and S. Sui. 2008. Study on dyeing of alizarin dye on modified cotton with chitosan. Journal of Dalian Polytechnic University 27 (4):380–84.
- Zhang, H., Z. Jin, and J. Wang. 2005. Microwave extraction the red pigment of peanut-skin and its property. Journal of Anhui Agriculture Science 33 (2):297–99.
- Zhong, S., P. Wu, and Z. Feng. 2010. Study on extraction technology and stability of the peanut-skin red pigment. Journal of Food Research and Development 31 (8):31–35.