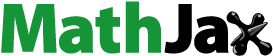
ABSTRACT
This experimental study aims to explore natural lignocellulosic fibers from Jenfokie and Doby plants. The effect of the fiber (water-retted and non-retted) extraction methods on physical, mechanical, thermal, chemical, and crystallinity properties were experimentally investigated for fibers collected from the eastern highlands of Ethiopia. The chemical composition (cellulose, hemicellulose, lignin, extractives, etc.) was determined after different treatment processes. The tensile strength maximum of up to 72 cN/tex and 56 cN/tex, and cellulose content up to 85% and 81% were obtained for Jenfokie and Doby retted extracted fibers, respectively. There was small difference lignin extracted by Klason method and the alkaline hydrogen peroxide (APH) method. Each step-wise extracted fiber was characterized to cognize the intrinsic changes during the multi-step extraction process. The diameters were determined by Optical Microscope (OM), the removal of non-cellulosic materials by Fourier Transform Infrared (FT-IR) spectroscopy, the thermal stability (up to 375°C) by thermogravimetry (TGA), and the crystallinity index (up to 73%) by using X-ray Diffraction (XRD). An improvement in cellulose content, density, moisture absorption, tensile strength, thermal stability, and crystallinity of Jenfokie (unstudied or new) and Doby plant retted fibers would be promising for composite and textile materials.
摘要
本实验旨在探索Jenfokie和Doby植物的天然木质纤维素纤维. 实验研究了从埃塞俄比亚东部高地采集的纤维(水浸和非浸)提取方法对物理、机械、热、化学和结晶性能的影响. 在不同的处理工艺后,测定了化学成分(纤维素、半纤维素、木质素、提取物等). Jenfokie和Doby retted提取纤维的最大拉伸强度分别高达72 cN/tex和56 cN/tex,纤维素含量分别高达85%和81%. Klason法和碱性过氧化氢法提取木质素差异较小. 对每一步提取的纤维进行表征,以识别多步提取过程中的内在变化. 通过光学显微镜(OM)测定直径,通过傅立叶变换红外(FT-IR)光谱测定非纤维素材料的去除,通过热重分析(TGA)测定热稳定性(高达375°C),通过X射线衍射(XRD)测定结晶度指数(高达73%). Jenfokie(未研究的或新的)和Doby植物脱胶纤维在纤维素含量、密度、吸湿性、拉伸强度、热稳定性和结晶度方面的改善将有望用于复合材料和纺织材料.
Introduction
The various environmental changes caused by the use of synthetic materials over the past few decades have promoted the use of biomaterials. Under these conditions, bio-composites are manufactured by using lignocellulose fibers derived from plants, which are cheaper, more abundant in nature, and more renewable than aramid, carbon, and glass fibers (Moudood et al. Citation2019). Recent environmental awareness has made the development of natural fiber composite materials (Sanjay et al. Citation2019). The attractive features of natural fibers have been their low cost, lightweight, low environmental impact, high specific modulus, and relatively good mechanical properties compared to the health hazards of composites reinforced with synthetic fibers (Rokbi et al. Citation2011).
Numerous natural fibers, such as flax, hemp, jute, oats, rye, cane, grass reeds, kenaf, ramie, nettle, sisal, and coir, have been studied in plastics (Memon et al. Citation2019). Among many natural fibers, stinging nettle (Urtica dioica) is a well-known herbaceous plant that groups 30–45 species that are considered as weeds in intensive agriculture (Suryawan et al. Citation2019). One of the primary interests of nettle is that the complete plant can be used for a range of purposes, such as food, fodder, medicine, cosmetics, biodynamic agriculture, and textile production (Ilyas et al. Citation2018). Doby –Girardina bullosa (steudel) wedd. – is one among those nettle plants with hollow tapered stems, seasonal, fine hairs, and it grows in the highlands of Ethiopia 2–3 m in length and 60–120 mm in external diameter and cause skin irritation when touched by the human body (Kale, Getachew Alemayehu, and Gorade Citation2020).
Climber plants (more than 110 families) are a group of vascular plants that germinate on the ground by winding, adhering to other plants to attain great stature, which share nearly one-quarter of the total vascular plants (He and Paul Chen Citation2014; Hossain, Sayedur Rahman, and Ahammad Khan Citation2015). Forty-two percent (42%) of the trees in the forest carried climbers’ liana (thick-stemmed) and vines (weak and thin stemmed) (Muoghalu and Okerinmola Okeesan Citation2005). The Jenfonkie, an Asclepiadaceae (Artemisia abyssinica Sch.Bip. ex A. Rich) climber plant, has a solid stem, non-flowering, separate joints or internodes, and the fibers were extracted between the internodes. It grows throughout the year and in rich soils up to 18 m in length and 8–22 mm in external stem diameter (middle) and releases a small amount of milky-like liquid substance between the core and the bark.
The objective of this study focused on the extraction and characterization of Ethiopian Highland Jenfokie and Doby herbaceous plant fibers for engineering application. The study further investigated the effects of extraction (retted and non-retted) method on the physical, mechanical, thermal, chemical, and crystallinity properties of Jenfokie and Doby fibers. This study contributes the new fiber from the Jenfokie plant for engineering applications.
Materials and methods
Sample preparation
Jenfokie and Doby plants were harvested from the highland of Dima Wuchale kebele, Arsi Zone, Oromia Regional State, Ethiopia with two different traditional fiber extraction methods (i.e., water retted and non-retted), as shown in –4. The details of fiber extraction steps are shown in the supplementary materials, Figures S1 and S2.
Figure 1. Jenfokie fiber unretted extraction process (a & b) Jenfokie plant, (c) bark with fiber, (d) Jenfokie fibers, (e) alkali treated fiber, and (f) bleached cellulose.

Figure 2. Jenfokie fiber water retted extraction process (a) Jenfokie plant, (b) internodes cut and water pre-retted Jenfokie stem (c) fiber (d) alkali treated fibers, and (e) bleached cellulose.

Reagents used
Chemicals such as toluene AR (99.9%) and sulfuric acid (assay: 98%); sodium hydroxide pellets LR (98%); ethanol absolute (99.8%), and hydrogen peroxide LR (H2O2, 30%) in aqueous solution were purchased from Fine General Trading, Ethiopia.
Fiber yield and density measurements
The bark and fiber yield were measured dividing the extracted bark by stem and fiber by bark, respectively (Nguyen, Pirak, and Yildiz Citation2019). The Archimedes principle was used for the density measurement with the help of pycnometer and distilled water (Ahmed et al. Citation2019; Lokantara et al. Citation2020).
where ρf is the density of the fibers, ρw is the density of the distilled water (1 g/cm3) in the pycnometer, and m1 is the mass of the pycnometer when it is empty, m2 is the mass of the pycnometer when it is filled with fiber, m3 is the mass of the pycnometer when it is filled with water and m4 is the mass of the pycnometer filled with fiber and water.
Fiber moisture content analysis
The weight of the sample was measured (M1) in three replicates after exposure. The samples were placed in an oven at 105°C for 24 h and were subsequently weighed (M2). The percentage of moisture content was weighed using the following formula:
Single fiber tensile test and diameter measurement
A single fiber tensile test was conducted using the TEXTECHNO STATIMAT ME+ machine at the Ethiopian Textile Industry Development Institute according to the ASTM-D-2256 method in five replicates. The diameter of both Jenfokie and Doby fibers was determined using an Optical Microscope (OM) by taking two different fibers and cutting them at three different points along their length.
Determination of chemical composition
The chemical composition analysis was done through gravimetric method as shown in (Manimaran et al. Citation2021). The AOAC (Cheung Citation1996; Idris, Abosede Wintola, and Jide Afolayan Citation2019), method was used to determine the ash content and the lignin was burned to determine in ash content in the in the lignin. The acid-insoluble lignin was determined by the TAPPI T222 om–88 method (Paschoal et al. Citation2015). By assuming that extractives, hemicellulose, lignin, ash, and cellulose are the only components of the total biomass, the cellulose content (%w/w) was estimated by difference, i.e. [% Cellulose = 100 − (% extractive + % ash + % acid-insoluble lignin + % hemicelluloses)] (Ayeni et al. Citation2015; Lin et al. Citation2010).
Delignification using Alkali Hydrogen Peroxide (AHP)
After toluene-ethanol and alkali pre-extraction, the sample was followed by AHP treatment in a water bath at a temperature of 85°C for 2 h using 30% H2O2 with a dosage of 10% (w/w) at an initial pH of 11.5 (adjusted with 5 M NaOH) (You et al. Citation2017) keeping the solid-to-liquid ratio constant at 1:10. Then, the sample was washed using distilled water and the weight loss (Wl) of lignin in three replicates.
where W1 and W2 represents the dry weight of the fiber before and after bleaching, respectively.
Fourier transform infrared (FT-IR) spectroscopy analysis
The functional groups of both Jenfokie and Doby fibers samples (raw, extractive free, alkali treated, and bleached) were performed on Spectrum 65 FT-IR (PerkinElmer) in the range of 4000–400 cm− 1 with four scans at a resolution of 4 cm− 1 using KBr pellets.
Thermogravimetric analysis
The thermal stability of Jenfokie and Doby fibers was characterized by using a thermo-gravimetric analyzer (TG-DTA, HCT-3, Beijing Henven Instruments, China) under constant nitrogen flow, from room temperature to 700°C, at a heating rate of 20°C/min. Approximately 10 mg of each sample was used.
Crystallinity index by X-ray diffraction (XRD)
Powdered milled samples were analyzed by an X-ray diffractometer (XRD-7000S) equipped with a Cu Kα radiation source operated at 30 mA and 40 kV, scanned in the 2θ range between 5° and 80°, at a scan speed of 3°/min. The crystallinity index was determined using the amorphous subtraction method after baseline subtraction (Douard et al. Citation2021), where Acryst is the area of all the crystallinity peaks, and Atotal is the total area under the diffractogram (Ovalle-Serrano, Blanco-Tirado, and Combariza Citation2018) Eq. (10).
Result and discussion
Fiber characterization
Effects of extraction techniques on Jenfokie and Doby fiber yield
Three replications were done and the average yield measurement values were evaluated at the bark level and fiber level, as shown in . The retting was done after four, seven, and ten days, and the maximum fiber yield was at seven days for both fibers chosen for this experimental investigation. Retted extracted fibers had greater uniformity and fiber quality than non-retted fibers due to the removal of pectin and water-soluble constituents from the fibers’ surface (Jankauskienė et al. Citation2015).
Table 1. Jenfokie and Doby bark and fiber yield.
Effect of extraction techniques on absorbing moisture of Jenfokie and Doby fibers
The moisture absorption of both Jenfokie and Doby chopped fibers was evaluated after exposed to an open atmosphere of humidity ranging from 25% to 54% . As a result, the increased moisture absorption of non-retted Jenfokie and Doby fibers may be attributed to the failure to remove pectin components, which are primarily found in the middle lamella (Baley, Morvan, and Grohens Citation2005; Martin et al. Citation2013).
Table 2. Mean mechanical test results of both retted and unretted Jenfokie and Doby fibers.
Table 3. Chemical composition and physical properties result obtained from Jenfokie and Doby fibers.
Effect of extraction techniques on Jenfokie and Doby fiber density and diameter
The density of non-retted and retted Jenfokie fibers (1.5–1.77 g/cm3) was slightly greater than Cotton (1.5–1.6 g/cm3), Flax (1.4–1.5 g/cm3) and Ramie (1.0–1.55 g/cm3) fibers, while the density of non-retted and retted Doby fibers (0.88–1.39 g/cm3) is comparable with Bamboo (0.6–1.1 g/cm3) fibers (Dittenber and GangaRao Citation2012). The increase in density of water retted Jenfokie and Doby fibers compared to non-retted was due to the removal of less dense surface impurities such as pectin, fats, waxes, etc., which causes increase in cellulose crystallinity and packing density, as recommended in previous study (KılınÇ et al. Citation2018).
Some of the fiber’s diameters measured are shown in . Jenfokie fiber diameters range from 131.85 to 258.90 μm and from 110.17 to 134.36 μm for non-retted and retted fibers, respectively. The fiber diameter of Doby measured varies from 89.05–109.60 µm and 75–99.74 µm for non-retted and retted fibers, respectively. The diameters of Jenfokie and Doby fibers are in the range of other bast fibers such as Acacia leucophloea (168 µm), Coir (280–380 µm), Chloris barbata (180–200 µm), Epipremnum aurem (105–150 µm), Thespesia populnea (120–200 µm), Enset (333.97 µm) and Sisal (474.4 µm) (Bekele, Lemu, and Jiru Citation2022; Seki et al. Citation2022). A slight increase in the average diameters of non-retted Jenfokie and Doby fibers observed in this study might be from the non-removal of pectin (middle lamellae) and other substances from the surface of the fibers, as noted by (Dey et al. Citation2021).
Effect of the gauge length and extraction techniques on the tensile strength of Jenfokie and Doby fibers
shows the summary of the mechanical properties in which the highest tensile strength and Young’s modulus were obtained for both retted extracted fibers due to the higher cellulose content in the fibers, respectively. This observation is in line with the results reported in previous studies (Tarabi, Ghasemloo, and Mani Citation2016). An increased amount of hemicellulose and extractive content in the non-retted fibers also contributed to the reduction in tensile strength. The tensile strength result obtained in this study were Jenfoki 62.07−72.7 cN/tex and Doby 36.69–56.84 cN/tex fibers at lower gauge length is comparable with other fibers such as Caster oil (57.8 − 86.9 cN/tex), Flax (57 cN/tex) fibers, etc. (Belachew, Gebino, and Haile Citation2021).
As the gauge length increased from 10 cm to 20 cm, the elongation of both retted and non-retted fibers decreased (Tamanna et al. Citation2021). The lower elongation percentage of retted fibers may be due to the smaller diameter of the fiber bundles compared to non-retted fibers, which resulted from the removal of some constituents from the fibers’ surface. This result is in-line with previous reports (Dey et al. Citation2021).
Effect of Jenfokie and Doby fibers extraction methods on the chemical composition
The number of extractives of non-retted fibers was more significant than the amount of water-retted extracted fibers due to the removal of some constituents like fats, pectin, etc., from the fiber surface during the retting process as shown in (Mazian et al. Citation2018).
The greater the ash content found in non-retted than retted Jenfokie and Doby fibers was due to the presence of pectin, as reported in previous study (Nguyen, Pirak, and Yildiz Citation2019). A slight increase in lignin content of both retted fibers is probably due to substantial loss of carbohydrates, soluble extractable, etc., as recommended in previous study (Placet, Day, and Beaugrand Citation2017). As noted and studied by (Wang et al. Citation2019), the reduction of hemicellulose content for retted Jenfokie and Doby fibers was with slight glucose loss after the retting process. The cellulose content obtained for Jenfokie (79.43–84.87 wt%) and Doby (73.90–81.20 wt%) is comparable with other higher cellulose content plant fibers such as Ramie (68.6–85 wt%), PALF (70–83 wt%), Nettle (86 wt%) and Cotton (82.7–90 wt%) (Asmare and Technol Citation2017; Dittenber and GangaRao Citation2012). A slight increase in cellulose content was found in the case of water-retted fibers in this study, probably due to the removal of pectin and non-cellulosic substances in the water phase (Brindha et al. Citation2019).
Effect of AHP extraction on delignification of Jenfokie and Doby fibers
The delignification weight loss after AHP extraction were 3.1%, 3.2%, 3.0%, and 3.2% for JU – AHP, JR – AHP, DU – APH, and DR – APH, respectively. A small difference between acid-insoluble lignin extracted by 72% H2SO4 and lignin extracted by the AHP process might be that some amounts of lignin were removed during alkali extraction, and acid-soluble lignin was not considered in this study which probably increases the amount Klason lignin (Wang et al. Citation2019).
FT-IR spectroscopy result
Effect of Jenfokie and Doby fibers extraction techniques on the chemical composition
The chemical functional group changes at each extraction step (raw, toluene-ethanol, alkali, and AHP bleaching) of both Jenfokie and Doby fibers are shown in from FT-IR analysis. The OH stretching vibrations of the hydrogen-bound hydroxyl groups from cellulose, hemicellulose, and lignin are represented by the absorption bands in the range of 3346–3440 cm− 1 seen in the spectra of all the fibers. The band from 2899 to 2925 cm−1 is characteristic of C – H stretching in cellulose, hemicellulose, and lignin. The spectra on both Jenfokie and Doby fibers had an absorption band from 1727 to 1744 cm−1 which is associated with the C=O bond of the acetic and ester groups of the hemicelluloses, and carboxyl aldehyde in lignin. Additionally, waxes and fats exhibit absorbance in the C=O (1727–1744 cm− 1) bond region from the ester group. After alkali treatment and bleaching, the peaks decrease and disappear due to the removal of hemicellulose and lignin content from the surface of the fibers (Li et al. Citation2015).
Figure 7. FT-IR spectral of stepwise extracted lignocellulose (a) unretted Jenfokie fibers, (b) retted Jenfokie fibers, (c) unretted Doby fibers, and (d) retted Doby fibers.

Both fibers in the spectra in the range of 1601–1644 cm− 1 represents, the OH bending vibration of absorbed water. These bands, further in the spectrum of both Jenfokie and Doby samples, are attributed to lignin C – H deformation. With the removal of lignin, these bands become weak and narrow. The bands of 1424‒1444 cm− 1, the characteristic absorbance of aromatic C=C stretching and CH2 bending found in lignin (Viju and Thilagavathi Citation2021) showed that the alkali treatment slightly affected and disappeared after bleaching with alkaline hydrogen peroxide in both Doby and Jenfokie fibers.
Effect of extraction techniques on Jenfokie and Doby fibers thermal stability
The thermal stability of Jenfokie and Doby fibers was determined with the aid of Thermogravimetric (TGA) and its Differential Thermogravimetric (DTG) analysis curves after stepwise extraction. For each progressively extracted retted and non-retted fiber, illustrates the mass loss by TGA and the rate of degradation by DTG as a function of temperature. According to (Shahedifar and Masoud Rezadoust Citation2013), hemicelluloses degrade between 180°C and 350°C, cellulose degrades between 250°C and 380°C, and lignin degrades between 250°C and 500°C.
Figure 8. TGA/DTG curves of both retted and unretted Jenfokie and Doby fibers (a) unretted Jenfokie fiber, (b) retted Jenfokie fiber, (c) retted Doby fiber, and (d) retted Doby fiber.

When the temperature ranged from room temperature (RT) to 140°C, weight loss ranged from 6.1% to 13.3%, which represents moisture evaporation and the decomposition of volatile extractives, as shown in . The highest weight loss for both non-retted raw fibers could be due to the presence of volatile extractives on the surface of the fibers (Sadrmanesh and Chen Citation2019). The second weight loss of both raw fibers was recorded between 226.1°C and 407.5°C attributed to the degradation of hemicellulose, pectin and cellulose. Non-retted fibers degrade at a lower temperature than retted extracted fibers because the decomposition of the chemicals starts at the surface.
Table 4. Thermal degradation temperatures and residual weight of the investigated Jenfokie and Doby lignocellulose.
The degradation temperature of extractive free samples was increased for both retted and non-retted fiber due to the thermal depolymerization of hemicelluloses and the breakdown of glycosidic linkages of cellulose. The major weight loss of up to 55.4% is related to the degradation of cellulose. The TGA curve for both fibers shifted to a higher temperature after they were bleached with hydrogen peroxide compared to raw fibers.
The third degradation occurs between 387.8°C and 625°C due to the breakdown of non-polysaccharides. The rate of thermal degradation after alkali extraction was higher than that of the raw fibers attributed to the removal of hemicelluloses as reported by (Martin et al. Citation2013; Xiao et al. Citation2015). Lastly, the residual ash and char levels were decreased for both retted and non-retted Doby and Jenfokie lignocellulose fibers due to high char contributors of lignin and hemicellulose, as recommended in previous study (Dizbay-Onat et al. Citation2018). The maximum decomposition temperature of Jenfokie (345.1–355.8°C) and Doby (323.5–347.6°C) raw fibers are comparable with other natural fibers such as Jute (283°C), Okra (359°C), Hemp (390°C), Curaua (335°C) and Kenaf (284°C) even though this result is increased after alkali treatment and bleaching (Sampathkumar et al. Citation2015). An increase in cellulose content for retted Jenfokie and Doby fibers is observed might be due to the removal of non-cellulosic materials (Mazian et al. Citation2020).
Effect of extraction techniques on Jenfokie and Doby fibers crystallinity
XRD patterns of both retted and non-retted Jenfokie and Doby (raw, treated with alkali, and bleached) powder fibers are represented in . The deconvolution of XRD pattern shows major crystalline peaks around 2θ = 15.5°, 16.5°, and 22.94° for retted and non-retted raw fibers corresponding to (10), (1 1 0), and (2 0 0) planes, respectively. The beginning peak around 15.5° indicates the diffraction of cellulose type I, which represents the crystalline constitute of cellulose (Araújo, Vilarinho, and Machado Citation2019). While crystalline peaks for alkaline extracted and bleached are around 2θ = 12.5°, 20.4°, and 22° can be attributed to the planes of (1
0), (1 1 0), and (0 2 0) due to cellulose II, respectively (Gong et al. Citation2017). Strong alkali extraction in combination with bleaching treatment is known to induce a partial transformation of cellulose type I to cellulose type II structure. The crystallinity index is increased after alkali treatment and bleaching in both Jenfokie and Doby fibers, this is most probably due to the removal of amorphous materials such as waxes, lignin, and hemicellulose (Dalle et al. Citation2021).
Figure 9. X–ray diffraction pattern of Jenfokie and Doby fibres. (a) Unretted Jenfokie fibres, (b) retted Jenfokie fibres, (c) unretted Doby fibres, and (d) retted Doby fibres.

The XRD result showed an increase in the CI after alkali extraction and bleaching of both retted and non-retted fibers as shown in . The peaks were more defined after dewaxing, alkali, and hydrogen peroxide extraction which might be resulted from the removal of the amount of non-cellulosic materials such as contributors such as hemicellulose, lignin, and wax (Kamali and Meghdad Citation2022). The crystallinity index after stepwise extraction up to 65.23–73.33% for Jenfokie and 68.62 − 70.53% for Doby cellulose obtained is comparable with other natural fibers such as Sansevieria cylindrica (60%), Acacia planifrons (65.38%), Jute (71.39%), and Sisal (62.8%) (Totong et al. Citation2022; Vinod et al. Citation2021).
Table 5. The CI of both retted and unretted Jenfokie and Doby fibers.
The higher CI of retted Jenfokie and Doby fibers might be due to the degradation of non-cellulosic compounds during the water-retting process (Mazian et al. Citation2019). After stepwise extraction, the CI was increased, which might be due to the decrease in non-cellulosic materials that promote an increase in cellulose content (Marques et al. Citation2020; Pereira et al. Citation2021).
Conclusion
This study, shows the extraction method highly influences most of the fiber properties. Pre-retting improves the ease of removal of the bark from the stem. Water retted Jenfokie and Doby fibers had higher cellulose content up to 85% and 81%, respectively. Water retted Jenfokie and Doby fibers showed better tensile strength (72.7 cN/tex and 56.84 cN/tex), and flexural strength (3275.7 cN/tex and 1669.19 cN/tex) at lower gauge lengths, respectively
An increase in CI was observed for both retted extracted fibers. The TG curves for both retted and non-retted extracted fibers show an increase in thermal stability after toluene-ethanol (up to 363.9°C), alkali removed (375.7°C), and alkali hydrogen peroxide (368.9°C) treated fibers compared to the raw (355.8°C) fibers. Finding a new (Jenfokie) fiber having the highest quantity of cellulose content, tensile strength, and thermal properties would be a promising material for the next level of engineering applications.
Highlights
In this work, the fibers and cellulose were extracted from the natural lignocellulosic fibers of Jenfokie and Doby plants. The extraction of fibers and cellulose from Doby plants is compared with Jenfokie (relatively unstudied/new) plants. The key highlights of this work are mentioned below:
Water-retted extraction of both Jenfokie and Doby fibers improved the physicochemical, mechanical, and chemical properties compared to unretted/dry retted extraction process.
An increase in thermal stability and crystallinity index was observed for both retted-extracted Jenfokie and Doby fibers.
The high cellulose content of retted-extracted fibers ensures good physical, mechanical, and thermal properties.
Tensile strength maximum up to 72 cN/tex and 56 cN/tex and the cellulose content of up to 85% and 81% were obtained for Jenfokie and Doby retted extracted fibers, respectively.
An improvement in cellulose content, density, moisture absorption, tensile strength, thermal stability, and crystallinity of Jenfokie plant (unstudied or new) fiber would be a promising material for composite and textile applications.
We confirm that neither the manuscript nor any parts of its content are currently under consideration or published in another journal.
Credit author statement
Solomon Estifo Wossine: Data collection, Testing, Investigation, Methodology, Result analysis, Writing-original draft. Thothadri Ganesh Conceptualization, Methodology, Supervision, Review & Editing and validation. Habtamu Beri Tufa Conceptualization, Methodology, Validation and Supervision.
Supplemental Material
Download MS Word (62.6 KB)Acknowledgements
The first author’s PhD study was made possible and supported by Wachamo University and Adama Science and Technology University (ASTU), both in Ethiopia, the authors acknowledge.
Disclosure statement
No potential conflict of interest was reported by the authors.
Supplementary material
Supplemental data for this article can be accessed online at https://doi.org/10.1080/15440478.2023.2229518
Additional information
Funding
References
- Ahmed, J., M. A. Balaji, S. S. Saravanakumar, and P. Senthamaraikannan. 2019. A comprehensive physical, chemical and morphological characterization of novel cellulosic fiber extracted from the stem of Elettaria cardamomum plant. Journal of Natural Fibers 18 (10):1460–17. doi:10.1080/15440478.2019.1691121.
- Araújo, D., M. Vilarinho, and A. Machado. 2019. Effect of combined dilute-alkaline and green pretreatments on corncob fractionation: Pretreated biomass characterization and regenerated cellulose film production. Industrial Crops and Products 141. doi:10.1016/j.indcrop.2019.111785.
- Asmare, T. A. D. E. L. E., and J. Technol. 2017. Effect of maturity on fundamental properties of nettle fibers. JTFT 1:1–10.
- Ayeni, A. O., O. A. Adeeyo, O. M. Oresegun, and T. E. Oladimeji. 2015. Compositional analysis of lignocellulosic materials: Evaluation of an economically viable method suitable for woody and non-woody biomass. American Journal of Engineering Research 4 (4):14–19.
- Baley, C., C. Morvan, and Y. Grohens. 2005. Influence of the absorbed water on the tensile strength of flax fibers. Macromolecular Symposia 222 (1):195–202. doi:10.1002/masy.200550425.
- Bekele, A. E., H. G. Lemu, and M. G. Jiru. 2022. Experimental study of physical, chemical and mechanical properties of enset and sisal fibers. Polymer Testing 106:107453. doi:10.1016/j.polymertesting.2021.107453.
- Belachew, T., G. Gebino, and A. Haile. 2021. Extraction and characterization of indigenous Ethiopian castor oil bast fibre. Cellulose 28 (4):2075–86. doi:10.1007/s10570-020-03667-9.
- Brindha, R., C. K. Narayana, V. Vijayalakshmi, and R. P. Nachane. 2019. Effect of different retting processes on yield and quality of banana pseudostem fiber. Journal of Natural Fibers Nachane 16 (1):58–67. doi:10.1080/15440478.2017.1401505.
- Cheung, P. C. -K. 1996. Dietary Fibre Content and Composition of Some Edible Fungi Determined by Two Methods of Analysis. Journal of the Science of Food and Agriculture 73 (2): 255–260.
- Dalle, S., V. Karunakaran, A. Patrucco, M. Zoccola, L. Douard, S. Ronchetti, M. Gallo, A. Schreier, Y. Leterrier, J. Bras, et al. 2021. Valorization of byproducts of hemp multipurpose crop: Short non-aligned bast fibers as a source of nanocellulose. Molecules Bras 26 (16):4723. doi:10.3390/molecules26164723.
- Dey, P., B. S. Mahapatra, B. Pramanick, A. Ajay Kumar, M. S. Negi, J. Paul, D. K. Shukla, and S. P. Singh. 2021. Quality optimization of flax fibre through durational management of water retting technology under sub-tropical climate. Industrial Crops and Products 162:113277. doi:10.1016/j.indcrop.2021.113277.
- Dittenber, D. B., and V. S. GangaRao. 2012. Critical review of recent publications on use of natural composites in infrastructure. Composites Part A, Applied Science and Manufacturing 43 (8):1419–29. doi:10.1016/j.compositesa.2011.11.019.
- Dizbay-Onat, M., U. K. Vaidya, J. Anne G Balanay, and C. T. Lungu. 2018. Preparation and characterization of flax, hemp and sisal fiber-derived mesoporous activated carbon adsorbents. Adsorption Science & Technology 36 (1–2):441–57. doi:10.1177/0263617417700635.
- Douard, L., J. Bras, T. Encinas, and M. N. Belgacem. 2021. Natural acidic deep eutectic solvent to obtain cellulose nanocrystals using the design of experience approach. Carbohydrate Polymers 252:117136. doi:10.1016/j.carbpol.2020.117136.
- Gong, J., J. Li, J. Xu, Z. Xiang, and L. Mo. 2017. Research on cellulose nanocrystals produced from cellulose sources with various polymorphs. RSC Advances 7 (53):33486–93. doi:10.1039/C7RA06222B.
- He, J., and J. Paul Chen. 2014. A comprehensive review on biosorption of heavy metals by algal biomass: Materials, performances, chemistry, and modeling simulation tools. Bioresource Technology 160:67–78. doi:10.1016/j.biortech.2014.01.068.
- Hossain, G. M., M. Sayedur Rahman, and S. Ahammad Khan. 2015. Species composition, richness, density and distribution of climbers in relation to salinity in Sundarbans mangrove forest of Bangladesh. International Journal of Chemical, Environmental & Biological Sciences(ijcebs) 3 (1):78–83.
- Idris, O. A., O. Abosede Wintola, and A. Jide Afolayan. 2019. Comparison of the proximate composition, vitamins (ascorbic acid, α-tocopherol and retinol), anti-nutrients (phytate and oxalate) and the GC-MS analysis of the essential oil of the root and leaf of Rumex crispus L. Plants 8 (3):51. doi:10.3390/plants8030051.
- Ilyas, R. A., S. M. Sapuan, M. R. Ishak, E. S. Zainudin, and M. S. N. Atikah. 2018. “Nanocellulose reinforced starch polymer composites: A review of preparation, properties and application.” Proceeding: 5th international conference on applied sciences and engineering (ICASEA, 2018), Capthorne Hotel, Cameron Highlands, Malaysia.
- Jankauskienė, Z., B. Butkutė, E. Gruzdevienė, J. Cesevičienė, and A. L. Fernando. 2015. Chemical composition and physical properties of dew- and water-retted hemp fibers. Industrial Crops and Products 75:206–11. doi:10.1016/j.indcrop.2015.06.044.
- Kale, R. D., T. Getachew Alemayehu, and V. G. Gorade. 2020. Extraction and characterization of lignocellulosic fibers from girardinia bullosa (Steudel) wedd (Ethiopian kusha plant). Journal of Natural Fibers 17 (6):906–20. doi:10.1080/15440478.2018.1539940.
- Kamali, M., and J. Meghdad. 2022. Typha leaves fiber and its composites: A review. Journal of Natural Fibers 19 (13):4993–5007. doi:10.1080/15440478.2020.1870643.
- KılınÇ, A. Ç., S. Köktaş, M. Atagür, and M. Ö. Seydibeyoglu. 2018. Effect of extraction methods on the properties of althea officinalis L. fibers. Journal of Natural Fibers Seydibeyoglu 15 (3):325–36. doi:10.1080/15440478.2017.1325813.
- Lin, L., R. Yan, Y. Liu, and W. Jiang. 2010. In-depth investigation of enzymatic hydrolysis of biomass wastes based on three major components: Cellulose, hemicellulose and lignin. Bioresource Technology Jiang 101 (21):8217–23. doi:10.1016/j.biortech.2010.05.084.
- Li, X., C. Sun, B. Zhou, and Y. He. 2015. Determination of hemicellulose, cellulose and lignin in moso bamboo by near infrared spectroscopy. Scientific Reports 5 (1):1–11. doi:10.1038/srep17210.
- Lokantara, I. P., N. P. G. Suardana, I. W. Surata, and I. N. S. Winaya. 2020. A review on natural fibers: Extraction process and properties of grass fibers. International Journal of Mechanical Engineering and Technology (IJMET) 11 (1):84–91. doi:10.34218/IJMET.11.1.2020.009.
- Manimaran, P., M. R. Sanjay, P. Senthamaraikannan, S. S. Saravanakumar, S. Siengchin, G. Pitchayyapillai, and A. Khan. 2021. Physico-chemical properties of fiber extracted from the flower of celosia argentea plant. Journal of Natural Fibers 18 (3):464–73. doi:10.1080/15440478.2019.1629149.
- Marques, F. P., L. Mara Alexandre Silva, D. Lomonaco, M. de Freitas Rosa, and R. C. Leitão. 2020. Steam explosion pretreatment to obtain eco-friendly building blocks from oil palm mesocarp fiber. Industrial Crops and Products 143:111907. doi:10.1016/j.indcrop.2019.111907.
- Martin, N., N. Mouret, P. Davies, and C. Baley. 2013. Influence of the degree of retting of flax fibers on the tensile properties of single fibers and short fiber/polypropylene composites. Industrial Crops and Products 49:755–67. doi:10.1016/j.indcrop.2013.06.012.
- Mazian, B., A. Bergeret, J. C. Benezet, and L. Malhautier. 2018. Influence of field retting duration on the biochemical, microstructural, thermal and mechanical properties of hemp fibres harvested at the beginning of flowering. Industrial Crops and Products 116:170–81. doi:10.1016/j.indcrop.2018.02.062.
- Mazian, B., A. Bergeret, J. C. Benezet, and L. Malhautier. 2019. A comparative study of the effect of field retting time on the properties of hemp fibres harvested at different growth stages. Fibers 7 (12):108. doi:10.3390/fib7120108.
- Mazian, B., A. Bergeret, J. C. Benezet, and L. Malhautier. 2020. Impact of field retting and accelerated retting performed in a lab-scale pilot unit on the properties of hemp fibres/polypropylene biocomposites. Industrial Crops and Products 143:111912. doi:10.1016/j.indcrop.2019.111912.
- Memon, A. H., M. Hussain Peerzada, K. Muhammad, S. Ahmed Memon, S. Ali Mangi, and G. Mujtaba. 2019. Recent eco-friendly developments in personal protective clothing materials for reducing plastic pollution: A review. Engineering, Technology & Applied Science Research 9 (2):4012–18. doi:10.48084/etasr.2674.
- Moudood, A., W. Hall, A. Öchsner, H. Li, A. Rahman, and G. Francucci. 2019. Effect of moisture in flax fibres on the quality of their composites. Journal of Natural Fibers 16 (2):209–24. doi:10.1080/15440478.2017.1414651.
- Muoghalu, J. I., and O. Okerinmola Okeesan. 2005. Climber species composition, abundance and relationship with trees in a Nigerian secondary forest. African Journal of Ecology 43 (3):258–66. doi:10.1111/j.1365-2028.2005.00585.x.
- Nguyen, B. M. N., T. Pirak, and F. Yildiz. 2019. Physicochemical properties and antioxidant activities of white dragon fruit peel pectin extracted with conventional and ultrasound-assisted extraction. Cogent Food & Agriculture 5 (1):1633076. doi:10.1080/23311932.2019.1633076.
- Ovalle-Serrano, S. A., C. Blanco-Tirado, and M. Y. Combariza. 2018. Exploring the composition of raw and delignified Colombian fique fibers, tow and pulp. Cellulose 25 (1):151–65. doi:10.1007/s10570-017-1599-9.
- Paschoal, G. B., C. M. Muller, G. M. Carvalho, C. A. Tischer, and S. Mali. 2015. Isolation and characterization of nanofibrillated cellulose from oat hulls. Quimica Nova 38:478–82. doi:10.5935/0100-4042.20150029.
- Pereira, P. H. F., H. L. Ornaghi, V. Arantes, M. O. H. Cioffi, and Carbohydrate Research Cioffi. 2021. Effect of chemical treatment of pineapple crown fiber in the production, chemical composition, crystalline structure, thermal stability and thermal degradation kinetic properties of cellulosic materials. Carbohydrate Research 499:108227. doi:10.1016/j.carres.2020.108227.
- Placet, V., A. Day, and J. Beaugrand. 2017. The influence of unintended field retting on the physicochemical and mechanical properties of industrial hemp bast fibres. Journal of Materials Science 52 (10):5759–77. doi:10.1007/s10853-017-0811-5.
- Rokbi, M., H. Osmani, A. Imad, and N. Benseddiq. 2011. Effect of chemical treatment on flexure properties of natural fiber-reinforced polyester composite. Procedia Engineering 10:2092–97. doi:10.1016/j.proeng.2011.04.346.
- Sadrmanesh, V., and Y. Chen. 2019. Bast fibres: Structure, processing, properties, and applications. International Materials Reviews 64 (7):381–406. doi:10.1080/09506608.2018.1501171.
- Sampathkumar, D., R. Punyamurthy, B. Bennehalli, and S. C. Venkateshappa. 2015. Physical characterization of natural lignocellulosic single areca fiber. Ciência & Tecnologia dos Materiais 27 (2):121–35. doi:10.1016/j.ctmat.2015.10.001.
- Sanjay, M. R., S. Siengchin, J. Parameswaranpillai, M. Jawaid, C. Iulian Pruncu, and A. Khan. 2019. A comprehensive review of techniques for natural fibers as reinforcement in composites: Preparation, processing and characterization. Carbohydrate Polymers 207:108–21. doi:10.1016/j.carbpol.2018.11.083.
- Seki, Y., F. Selli, Ü. H. Halis Erdoğan, M. Atagür, and M. Seydibeyoğlu. 2022. A review on alternative raw materials for sustainable production: Novel plant fibers. Journal Cellulose Seydibeyoğlu 29 (9):4877–918. doi:10.1007/s10570-022-04597-4.
- Shahedifar, V., and A. Masoud Rezadoust. 2013. Thermal and mechanical behavior of cotton/vinyl ester composites: Effects of some flame retardants and fiber treatment. Journal of Reinforced Plastics and Composites 32 (10):681–88. doi:10.1177/0731684413475911.
- Suryawan, I. G. P. A., N. P. G. Suardana, I. N. S. Winaya, I. W. B. Suyasa, and I. P. Lokantara. 2019. Study of stinging nettle fibers as a reinforcing of composite materials based on its growing region. IOP Conference Series: Materials Science and Engineering 539 (1):012006. doi:10.1088/1757-899X/539/1/012006.
- Tamanna, T. A., S. Alimuzzaman Belal, M. Abul Hasan Shibly, and A. Nabi Khan. 2021. Characterization of a new natural fiber extracted from corypha taliera fruit. Scientific Reports 11 (1):1–13. doi:10.1038/s41598-021-87128-8.
- Tarabi, A., S. Ghasemloo, and M. Mani. 2016. Experimental investigation of a variable-span morphing wing model for an unmanned aerial vehicle. Journal of the Brazilian Society of Mechanical Sciences and Engineering 38 (7):1833–41. doi:10.1007/s40430-015-0405-6.
- Totong, T., W. Wardiningsih, M. Al-Ayyuby, R. Wanti, and R. Rudy. 2022. Extraction and characterization of natural fiber from furcraea foetida leaves as an alternative material for textile applications. Journal of Natural Fibers Rudy 19 (13):6044–55. doi:10.1080/15440478.2021.1904477.
- Viju, S., and G. Thilagavathi. 2021. Effect of alkali treatment of nettle fibers on oil absorbency. Journal of Natural Fibers 18 (12):2092–101. doi:10.1080/15440478.2020.1723776.
- Vinod, A., R. Vijay, D. Lenin Singaravelu, M. R. Sanjay, S. Siengchin, Y. Yagnaraj, and S. Khan. 2021. Extraction and characterization of natural fiber from stem of cardiospermum halicababum. Journal of Natural Fibers Khan 18 (6):898–908. doi:10.1080/15440478.2019.1669514.
- Wang, Z., L. Qu, J. Qian, Z. He, and S. Yi. 2019. Effects of the ultrasound-assisted pretreatments using borax and sodium hydroxide on the physicochemical properties of Chinese fir. Ultrasonics Sonochemistry 50:200–07. doi:10.1016/j.ultsonch.2018.09.017.
- Xiao, S., R. Gao, Y. Lu, J. Li, and Q. Sun. 2015. Fabrication and characterization of nanofibrillated cellulose and its aerogels from natural pine needles. Carbohydrate Polymers 119:202–09. doi:10.1016/j.carbpol.2014.11.041.
- You, S., Y. S. Ok, S. S. Chen, D. C. W. Tsang, E. E. Kwon, J. Lee, and C. H. Wang. 2017. A critical review on sustainable biochar system through gasification: Energy and environmental applications. Bioresource Technology 246:242–53. doi:10.1016/j.biortech.2017.06.177.