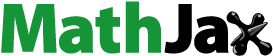
ABSTRACT
In order to compare the degradation of PLA composites by different microorganisms, and reveal the degradation mechanism. Actinomyces israelii, Aspergillus niger, Trichoderma harzianum and Phanerochaete chrysosporium were selected to degrade PLA composites. The weight loss, functional groups, thermal properties and crystallinity of the PLA composites before and after degradation were analyzed. The results indicated that A. israelii and A. niger had the strongest ability to degrade the composites, and the weight loss of the PLA composites reached 7.14% and 6.99% respectively after degradation for 28 days, which were significantly higher than that of the control treatment. According to the characterizations, it was found that the degradation was mainly hydrolysis by the microorganisms and their protease and lipase. Microbial degradation would lead to cracks and dents on the surface of the samples, and increase their contact area with microorganisms, thus accelerating the degradation rate. A. israelii and T. harzianum were more inclined to degrade the PLA in the composites during microbial degradation. Microbial degradation destroyed the crystalline region of the composites, which made they easier to degrade. The results would be helpful to explore the microbial degradation mechanism of PLA composites, and promotes its application in different fields.
摘要
为了比较不同微生物对聚乳酸复合材料的降解,揭示降解机理. 选择以色列放线菌、黑曲霉、哈茨木霉和黄孢Phanerochete降解聚乳酸复合物. 分析了降解前后PLA复合材料的重量损失、官能团、热性能和结晶度. 结果表明,以色列曲霉和黑曲霉对复合材料的降解能力最强,降解28天后PLA复合材料的失重率分别达到7.14%和6.99%,明显高于对照处理. 根据表征,发现降解主要是微生物及其蛋白酶和脂肪酶的水解. 微生物降解会导致样品表面出现裂纹和凹痕,并增加其与微生物的接触面积,从而加快降解速度. A.israelii和T.harzianum在微生物降解过程中更倾向于降解复合材料中的PLA. 微生物降解破坏了复合材料的结晶区域,使其更容易降解. 研究结果有助于探索聚乳酸复合材料的微生物降解机理,促进其在不同领域的应用.
Introduction
Polylactic acid (PLA) is mainly produced by corn, cassava and other agricultural resources (Papong et al. Citation2014; Singhvi, Zinjarde, and Gokhale Citation2019), which can completely degrade into water and carbon dioxide under certain circumstances (Chai et al. Citation2022; Da Silva et al. Citation2018), therefore PLA is considered as a renewable degradable polymer material (Dubey et al. Citation2016; Salazar-Sanchez et al. Citation2019). PLA has good mechanical properties and biocompatibility (Armentano et al. Citation2013), in which case it has developed rapidly. At present, the global annual demand for PLA has reached 30 million tons (Huang et al. Citation2021). And as countries and regions around the world implement “plastic prohibition orders,” PLA will be used more. Currently, PLA has been used in many fields such as medical treatment, construction and food packaging (Li et al. Citation2022). However, the poor toughness limited its development (Burrola-Nunez et al. Citation2019), while this shortcoming can be overcome by the addition of plasticizers (Armentano et al. Citation2013). The preparation of composites of PLA and natural fibers by injection molding, molding and additive manufacturing is also one of the important ways to solve its poor toughness (Chougan et al. Citation2022; Chougan, Ghaffar, and Al-Kheetan Citation2023). This can also realize the high-value utilization of natural fibers such as crop straw (Ghaffar and Fan Citation2017; Ghaffar, Fan, and McVicar Citation2015). With the deepening of the research on PLA, it would be gradually popularized to various fields, especially to disposable plastic products. The consequent problem is the production of a large amount of PLA waste, if not timely consumption of these waste will also cause environmental pollution. It is meaningful to study the degradation microorganism of PLA composites for its rapid degradation.
The degradation of PLA in natural environment is very slow, and its complete disappearance may take several decades (Pattanasuttichonlakul, Sombatsompop, and Prapagdee Citation2018). At present, the research of PLA degradation mainly focuses on hydrolytic degradation, photodegradation, enzymatic degradation and microbial degradation (Zaaba and Jaafar Citation2020). Microorganism is the key to the degradation of PLA in the natural environment (Xu et al. Citation2022). Protease and lipase produced by microorganisms can cause the degradation of PLA from polymer to small molecules and monomer lactic acid (Stepczynska and Rytlewski Citation2018). One of the earliest microorganisms found to degrade PLA was actinomycetes (Kawashima et al. Citation2022). Some studies found that the soil contained more PLA degrading microorganisms, 79 microorganisms (67 actinomycetes, 7 bacteria and 5 fungi) were isolated from the soil, and it was found that 32.9% (26 strains) of microorganisms can degrade PLA (Penkhrue et al. Citation2015). After the PLA composites were buried in the soil for 100 days, the proportion of Bionectriaceae in the soil was found to be significantly increased, which means that Bionectriaceae is highly likely to have the ability to degrade the PLA composites (Chai et al. Citation2023). Some scholars used Aspergillus flavus to degrade PLA and PLA/jute composites for 7 months, and found that the thickness of treated PLA and PLA/jute composites decreased by 52% and 63%, respectively (Karimi-Avargani et al. Citation2020). A Pleurotus ostreatus be used to degrade the PLA composites, and the mass loss of the treated PLA composites reached 48% after 6 months (Hidayat and Tachibana Citation2012). A strain of Bordetella petrii was isolated from the soil and found that it could degrade not only the non-crystalline region of PLA but also the crystalline region (Kim and Park Citation2010). In a nutshell, the degradation microorganisms of PLA composites can effectively improve its degradation rate. Therefore, research on the degradation of PLA composites by different microorganisms would help promote the replacement of non-degradable plastics by the PLA composites in different fields.
In order to compare the degradation of PLA composites by different microorganisms, and reveal the degradation mechanism. In this study, the wheat straw/PLA composites were prepared by injection molding, and a small amount of sodium alginate was added to improve their degradation performance. Four microorganisms (Actinomyces israelii, Aspergillus niger, Trichoderma harzianum and Phanerochaete chrysosporium) were selected to degrade the PLA composites. The weight loss of the PLA composites under different treatments was compared after degradation. And the characterization (microstructure, Fourier transform infrared spectroscopy, thermal properties and XRD) was tested to analyze the degradation mechanism. The results would be helpful to explore the microbial degradation mechanism of PLA composites, and promotes the application of PLA composites in different fields.
Experimental
Materials
The A. israelii, A. niger, T. harzianum and P. chrysosporium used in this experiment were all from the bioresource laboratory of the College of Engineering, Nanjing Agricultural University, China. The wheat straw was taken from Lianyungang, China, in June 2022, and the wheat straw was crushed and passed through a 100-mesh screen before the molding test. The PLA type Ingeo 4032 D from NatureWorks LLC. The sodium alginate is food grade, purchased from Mingyue Seaweed Co., Ltd., Qingdao, China. The peptone was purchased from Shanghai Bio-way technology Co., Ltd., China. The yeast extraction was acquired from Oxoid (Basingstoke, Hampshire, UK). And the other chemical reagents used in this study were analytically pure and purchased from Sinopharm Chemical Reagent Co., Ltd., China.
Media
Gause’s liquid medium was used to culture A. israelii, which included 10 g starch, 1 g peptone, 1 g potassium nitrate, 0.2 g dipotassium hydrogen phosphate, 0.3 g magnesium sulfate, 0.5 g sodium chloride, 1 g calcium carbonate, 0.5 g yeast extraction, and up to 1 Liter of H2O. The pH was adjusted to 7.8, and the prepared medium was sterilized at 120°C for 20 min.
PDA liquid medium was used to culture A. niger, T. harzianum and P. chrysosporium, the recipe for PDA liquid medium (1 L) is as follows: 200 g of potatoes were cut into 1 cm3 pieces and boiled in deionized water for 30 min. Eight layers of gauze were used to filter out the residue, and 20 g of glucose was added. Finally, the PDA liquid medium was sterilized at 121°C for 20 min.
MM liquid medium was used for degradation of PLA composites, which included 12.8 g disodium hydrogen phosphate, 3 g potassium dihydrogen phosphate, 0.5 g sodium chloride, 1 g ammonium chloride, and 5 g dextrose per liter, and after autoclaving, two milliliters of 1 M magnesium sulfate and 0.1 mL 1 M calcium chloride were added.
Methods
Sample preparation
The wheat straw and PLA were weighed at 3:7 ratio, and 5% sodium alginate (the objective is to accelerate the degradation of PLA composites) was added. A mixer (SBH-5 L, Nanjing Xinbao Electromechanical Equipment Co., Ltd., China) was used to blend the raw materials thoroughly. Finally, the PLA composites were formed by an injection molding machine (WZS10D, Xinshuo Precision Machinery Co., Ltd., China). The screw and barrel temperature were set as 180°C, the screw speed was set as 36 rpm, the injection pressure was set as 0.6 MPa, the mold temperature was indoor temperature. The length, width and height of the finished PLA composites were 80, 10 and 4.5 mm, respectively.
Culture conditions of microorganisms and degradation
A. israelii was cultured in 250 mL Gause’s liquid medium, and A. niger, T. harzianum and P. chrysosporium were cultured in 250 mL PDA liquid medium. After the microorganisms had overgrown in the medium, and the cultured microorganisms were centrifuged (10000 rpm) and the supernatant was removed. These cultures were washed 3 times with sterile water, and then the clean cultures were transferred to 500 mL MM liquid medium. Finally, the PLA composites were added into MM liquid medium for degradation test. The PLA composites were added to MM liquid medium without any microorganism as the control treatment. The PLA composites were sampled at 7, 14, 21 and 28 days for further analysis.
Weight loss
The PLA composites were dried, numbered and weighed before being added to MM liquid medium. After degradation, the samples were taken out from the MM liquid medium, and the residual microorganisms and medium on the surface of the samples were cleaned with water. The PLA composites were dried in a dryer (80°C for 24 h). The PLA composites were weighed and the weight loss rate were calculated (formula 1):
Where the Wt% was weight loss rate of PLA composites after degradation, W0 was the weight before degradation and W1 was the weight after degradation.
Morphological observation
An ion sputtering instrument (E-1010, Hitachi, Japan) was used to spray gold on the surface of PLA composites. Then the microstructure of PLA composites was observed by a field emission scanning electron microscope (S-4800, Hitachi, Japan). The accelerating voltage of SEM was set to 15 kV, and the magnification is 2000 times.
Fourier transform infrared spectroscopy (FTIR)
The PLA composites were filed into powder by a file, and the powder of PLA composites was placed in an oven at 80°C for 6 h. Then 0.002 g of sample and 0.2 g of potassium bromide were weighted into a crucible and grind carefully. A tablet press was used to laminate the mixed samples. The samples were tested with a Fourier transform infrared spectrometer (Nicoleis10, Shanghai DM Chemical Technology Co., Ltd., Shanghai China) over a range from 4000 to 400 cm-1, with a resolution of 4 cm-1 and 16 scans.
Thermogravimetry
A chip analyzer (STA449 F3, Netzsch, China) was used to test the effects of microbial degradation on the thermal properties of PLA composites. The sample mass was 5 to 20 mg. The temperature range was 30 to 800°C, and the heating rate was set to 20°C/min. The purge gas and protective gas rate were set as 60 and 20 mL/min, respectively.
Xrd
X-ray diffraction (XRD) patterns were obtained with a diffractometer (X’Pert’PRO, PANalytical B.V., Netherlands) using Cu/Kα radiation at 40 KV and 40 mA over a range of 10–35°.
Data processing
Data processing was performed using Excel 2020 (Microsoft Corporation), SPSS Statistics 25.0 (IBM Corporation, New York, NY, USA) and Adobe Photoshop (Adobe Corporation, SJ). Significance was assessed using Duncan’s test (α = 0.05) for multiple comparisons. Graphics were drawn with OriginPro 2023 (OriginLab).
Results and discussion
Weight loss
Weight loss can directly reflect the degradation of PLA composites by different microorganisms (Behera, Mohanty, and Mohanty Citation2021; Kong et al. Citation2018; Luo et al. Citation2020). The PLA composites would fade and crack after microbial degradation (). The color of the PLA composites is mainly derived from the wheat straw. These pigments dissolve in water during microbial degradation, but it is not that the more PLA composites degradation, the more pigment loss. The weight loss of the PLA composites after microbial degradation were shown in . A. Niger showed good degradation ability for the PLA composites in the early stage of degradation. After degradation for 7 days, the weight loss rate of the PLA composites treated by A. Niger was 6.08%, which was significantly higher than those of the other treatments (P < .05). The weight loss of PLA composites treated by A. israelii increased to 5.95% after degradation for 14 days, second only to the treatment of A. Niger, and significantly higher than that of the control treatment (P < .05). However, the weight loss rate of PLA composites treated by A. israelii reached 7.14% after degradation for 28 days, which was higher than that of the treatment of A. Niger (6.99%). Combined with fading of PLA composites after degradation, it was found that PLA composites treated by A. israelii and A. Niger had less fading but high weight loss. This means that A. israelii and A. Niger are more inclined to degrade other components except pigments in the PLA composites. The results indicated that all the four microorganisms could accelerate the degradation of the PLA composites. Among them, A. israelii and A. Niger had the strongest ability to degrade the PLA composites.
Morphological properties
The microbial degradation of the PLA composites would fracture the polymer and cause cracks and dents on their surface (Behera, Sen, and Adhikari Citation2020; Behera, Srivastava, and Das Citation2022; Turco et al. Citation2021). The dents and cracks on the surface of the PLA composites would increase the contact area with microorganisms and accelerate the degradation of the PLA composites (Lima et al. Citation2020). The microstructures of the PLA composites before and after degradation were shown in . The surface of the PLA composites without degradation was smooth, but cracks and dents appear on their surface after degradation by different microorganisms. These cracks and dents proved that the PLA composites were degraded.
FTIR analysis
FTIR can analyze the changes of functional groups in PLA composites after microbial degradation (Behera, Manna, and Das Citation2021; Debeli et al. Citation2019; Lv et al. Citation2017; Wang et al. Citation2022). At present, hydroxyl group and carbonyl group are considered to have the most obvious changes in the degradation of PLA composites (Fortunati et al. Citation2012; He et al. Citation2021). The FTIR spectra of the PLA composites degraded by different microorganisms were shown in . After degradation for 28 days, the hydroxyl peak (3500–3000 cm−1) of the PLA composites treated by T. harzianum was increased, while the hydroxyl peaks of other treatments were decreased. However, hydrolysis of polymers usually leads to an increase in the number of hydroxyl groups, this means that degradation of the PLA composites in this study cannot be demonstrated by changes in hydroxyl groups. The number of carbonyl group (1758 cm−1) in PLA composites degraded by A. israelii, A. Niger and T. harzianum was significantly increased. The end of the fractured polymer was oxidized to form a new carbon-oxygen double bond, which results in an increase in the number of carbonyl groups in the PLA composites after degradation. These results were consistent with the conclusion that A. israelii, A. Niger and T. harzianum had stronger ability to degrade PLA composites. After degradation, a new peak appeared at the position of 668 cm−1, which represented C-Cl. In the degradation test, Cl element was contained in the medium. These Cl− replaced H+ on the unsaturated carbon atoms in the PLA to form halogenated hydrocarbons (). The peaks at 1680–1580 cm−1 split into multiple peaks after microbial degradation. We believe that these peaks represent carboxylate. The two oxygen atoms were almost equivalent after the carboxylic acid became carboxylate during the degradation of the PLA composites, and the properties of the original carbon-oxygen double bond and carbon-oxygen single bond tend to be the same. The two carbon-oxygen bonds would have a strong coupling, resulting in peak splitting. The medium used in this study contained a variety of metal cations (Na+, Ca2+, Mg2+, K+). We speculated that different metal cations would induce different changes in the carbon-oxygen double bond and carbon-oxygen single bond in carboxylate, which would lead to single peak splitting into multiple peaks(). It was found that the functional groups of the degraded composites have obvious changes compared with the original samples. However, there was no significant difference between the experimental and the control groups. This result further verified that the biodegradation of the PLA composites is mainly hydrolysis by the microorganisms and their secreted enzymes.
Thermal properties analysis
The TG and DTG curves of treated PLA composites by different microorganisms were shown in and . The thermal properties of the three components of PLA composites are different. The pyrolysis temperature of PLA is higher than those of wheat straw and sodium alginate, but the residual weight of PLA is lower than those of wheat straw and sodium alginate (Chai et al. Citation2022). Therefore, the thermal properties of the PLA composites before and after degradation can be used to analyze the loss of three components during microbial degradation. The initial temperature, peak temperature and final temperature of the original PLA composites were 297.9°C, 311.3°C and 325.6°C, respectively, and the residual weight at 800°C was 16.65%. After degradation, the pyrolysis temperature of all treatments increased and the residual mass decreased. This indicated that the loss of wheat straw and/or sodium alginate in PLA composites was higher than that of PLA during degradation. The pyrolysis temperature of the treated PLA composites by A. Niger was higher than that of the control treatment. However, the treatments of A. israelii and T. harzianum showed opposite results to the treatment of A. Niger. This result meant that the PLA content in the composites of the A. Niger treatment was higher than that of the control treatment after degradation, while the PLA content of the composites treated by A. israelii and T. harzianum was lower than that of the control. In another word, A. israelii and T. harzianum were more inclined to degrade the PLA in the composites.
Figure 6. TG and DTG curves of the PLA composites before and after degradation for 28 days by different microorganisms.

Table 1. Thermogravimetric analysis data of the PLA composites before and after degradation for 28 days by different microorganisms.
XRD analysis
The crystallinity is one of the factors affecting the degradation of PLA composites. Generally, the crystalline region of PLA composites is more difficult to degrade than the non-crystalline region (Zhang et al. Citation2008). The XRD curves of the PLA composites before and after microbial degradation were shown in . The 2θ values of 16.5 and 18.7 were characteristically peaks of PLA. The intensity of PLA peak decreased significantly after microbial degradation. In this study, the degradation of the PLA composites was mainly hydrolysis by the microorganisms and their proteases and lipases. This meant that hydrolysis can reduce the crystallinity of the PLA composites. The intensity of the crystal peak of the A. israelii treatment and the control treatment was the lowest after degradation. The results indicated that A. Niger, T. harzianum and P. chrysosporium degraded more amorphous regions in the PLA composites than the control. Yet A. israelii can better destroy the crystalline region in the PLA composites during the degradation. The reduction of the proportion of crystalline region in the PLA composites would further accelerate its degradation.
Conclusion
A. israelii and A. niger had strong ability to degrade PLA composites. After 28 days of degradation by A. israelii and A. niger, the weight loss of PLA composites reached 7.14% and 6.99%, respectively.
According to the thermal properties of PLA composites before and after microbial degradation, A. israelii and T. harzianum were more inclined to degrade PLA in PLA composites.
The crystallinity of the PLA composites of all treatments decreased significantly after microbial degradation. The results indicated that hydrolysis by microorganisms and their proteases and lipases can destroy the crystallization region of the PLA composites, and then promote the degradation rate.
Author contributions
Xicun Chai performed the experiments and wrote the manuscript. Chunxia He and Yutao Liu conceived the idea and designed the experiments, and revised the manuscript. Other authors helped to correct the manuscript.
Highlights
The degradation of PLA composites by four kinds of microorganisms was compared in this study.
Actinomyces israelii and Aspergillus niger had strong ability to degrade PLA composites.
Actinomyces israelii and Trichoderma harzianum were more inclined to degrade the PLA in the composites.
Hydrolysis of the PLA composites by microorganisms and their proteases and lipases can significantly reduce the crystallinity of the PLA composites.
Disclosure statement
No potential conflict of interest was reported by the author(s).
Additional information
Funding
References
- Armentano, I., N. Bitinis, E. Fortunati, S. Mattioli, N. Rescignano, R. Verdejo, M. A. Lopez-Manchado, and J. M. Kenny. 2013. Multifunctional nanostructured PLA materials for packaging and tissue engineering. Progress in Polymer Science 38 (10–11):1720–12. doi:10.1016/j.progpolymsci.2013.05.010.
- Behera, A. K., S. Manna, and N. Das. 2021. Effect of soy waste/cellulose on mechanical, water sorption, and biodegradation properties of thermoplastic starch composites. Starch-Starke 74 (1–2):2100123. doi:10.1002/star.202100123.
- Behera, A. K., C. Mohanty, and A. Mohanty. 2021. Development and characterization of biodegradable jute reinforced sapling pot. Journal of Natural Fibers 19 (14):7738–49. doi:10.1080/15440478.2021.1958409.
- Behera, A. K., R. Sen, and B. Adhikari. 2020. Environment-friendly fully biodegradable jute-poly(vinyl alcohol) modified soy composite development as plastic substitute. Journal of Natural Fibers 19 (3):905–14. doi:10.1080/15440478.2020.1764440.
- Behera, A. K., R. Srivastava, and A. B. Das. 2022. Mechanical and degradation properties of thermoplastic starch reinforced nanocomposites. Starch-Starke 74 (3–4):2100270. doi:10.1002/star.202100270.
- Burrola-Nunez, H., P. Herrera-Franco, H. Soto-Valdez, D. E. Rodriguez-Felix, R. Melendrez-Amavizca, and T. J. Madera-Santana. 2019. Production of biocomposites using different pre-treated cut jute fibre and polylactic acid matrix and their properties. Journal of Natural Fibers 18 (11):1604–17. doi:10.1080/15440478.2019.1693473.
- Chai, X. C., C. X. He, Y. T. Liu, E. Niyitanga, L. Y. Wang, and W. X. Zhang. 2023. Degradation of wheat straw/polylactic acid composites with and without sodium alginate in natural soil and the effects on soil microorganisms. Journal of Applied Polymer Science 140 (6):53447. doi:10.1002/app.53447.
- Chai, X. C., C. X. He, Y. T. Liu, E. Niyitanga, Y. Zhou, L. Y. Wang, and W. X. Zhang. 2022. Degradation of wheat straw/polylactic acid composites by Aspergillus niger. Polymer Composites 43 (3):1823–31. doi:10.1002/pc.26500.
- Chougan, M., S. H. Ghaffar, and M. J. Al-Kheetan. 2023. Graphene-based nano-functional materials for surface modification of wheat straw to enhance the performance of bio-based polylactic acid composites. Materials Today Sustainability 21:100308. doi:10.1016/j.mtsust.2022.100308.
- Chougan, M., S. H. Ghaffar, E. Mijowska, W. Kukulka, and P. Sikora. 2022. High-performance polylactic acid compressed strawboard using pre-treated and functionalised wheat straw. Industrial Crops and Products 184:114996. doi:10.1016/j.indcrop.2022.114996.
- Da Silva, D., M. Kaduri, M. Poley, O. Adir, N. Krinsky, J. Shainsky-Roitman, and A. Schroeder. 2018. Biocompatibility, biodegradation and excretion of polylactic acid (PLA) in medical implants and theranostic systems. The Chemical Engineering Journal 340:9–14. doi:10.1016/j.cej.2018.01.010.
- Debeli, D. K., Z. Zhang, F. S. Jiao, and J. S. Guo. 2019. Diammonium phosphate-modified ramie fiber reinforced polylactic acid composite and its performances on interfacial, thermal, and mechanical properties. Journal of Natural Fibers 16 (3):342–56. doi:10.1080/15440478.2017.1423255.
- Dubey, S. P., H. A. Abhyankar, V. Marchante, J. L. Brighton, K. Blackburn, C. Temple, B. Bergmann, G. Trinh, and C. David. 2016. Modelling and validation of synthesis of poly lactic acid using an alternative energy source through a continuous reactive extrusion process. Polymers 8 (4):164. doi:10.3390/polym8040164.
- Fortunati, E., D. Puglia, C. Santulli, F. Sarasini, and J. M. Kenny. 2012. Biodegradation of phormium tenax/poly(lactic acid) composites. Journal of Applied Polymer Science 125 (S2):E562–E72. doi:10.1002/app.36839.
- Ghaffar, S. H., and M. Z. Fan. 2017. An aggregated understanding of physicochemical properties and surface functionalities of wheat straw node and internode. Industrial Crops and Products 95:207–15. doi:10.1016/j.indcrop.2016.10.045.
- Ghaffar, S. H., M. Z. Fan, and B. McVicar. 2015. Bioengineering for utilisation and bioconversion of straw biomass into bio-products. Industrial Crops and Products 77:262–74. doi:10.1016/j.indcrop.2015.08.060.
- He, J., T. Yu, S. K. Chen, and Y. Li. 2021. Soil degradation behavior of ramie/thermoset poly(lactic acid) composites. Journal of Polymer Reserrch 28 (10):379. doi:10.1007/s10965-021-02715-7.
- Hidayat, A., and S. Tachibana. 2012. Characterization of polylactic acid (PLA)/kenaf composite degradation by immobilized mycelia of Pleurotus ostreatus. International Biodeterioration & Biodegradation 71:50–54. doi:10.1016/j.ibiod.2012.02.007.
- Huang, S. Y., Y. F. Xue, B. Yu, L. M. Wang, C. Zhou, and Y. H. Ma. 2021. A review of the recent developments in the bioproduction of polylactic acid and its precursors optically pure lactic acids. Molecules 26 (21):6446. doi:10.3390/molecules26216446.
- Karimi-Avargani, M., F. Bazooyar, D. Biria, A. Zamani, and M. Skrifvars. 2020. The special effect of the Aspergillus flavus and its enzymes on biological degradation of the intact polylactic acid (PLA) and PLA-Jute composite. Polymer Degradation and Stability 179:109295. doi:10.1016/j.polymdegradstab.2020.109295.
- Kawashima, N., J. Tokuda, T. Yagi, and K. Takahashi. 2022. Isolation of a Nocardiopsis chromatogenes strain that degrades PLA (polylactic acid) in pig waste-based compost. Archives of Microbiology 204 (10):599. doi:10.1007/s00203-022-03144-w.
- Kim, M. N., and S. T. Park. 2010. Degradation of Poly(L-lactide) by a Mesophilic Bacterium. Journal of Applied Polymer Science 117 (1):67–74. doi:10.1002/app.31950.
- Kong, X., D. C. Jin, S. L. Jin, Z. G. Wang, H. Q. Yin, M. Y. Xu, and Y. Deng. 2018. Responses of bacterial community to dibutyl phthalate pollution in a soil- vegetable ecosystem. Journal of Hazardous Materials 353:142–50. doi:10.1016/j.jhazmat.2018.04.015.
- Li, X. R., Y. Lin, M. L. Liu, L. P. Meng, and C. F. Li. 2022. A review of research and application of polylactic acid composites. Journal of Applied Polymer Science 140 (7). doi:10.1002/app.53477.
- Lima, E. M. B., R. N. Oliveira, A. Middea, I. M. De Castro, M. D. Mattos, T. T. M. Neves, L. F. Da Costa, R. Neumann, and M. I. B. Tavares. 2020. Degradation of PLA biocomposites containing mango seed and organo montmorillonite minerals. Journal of Natural Fibers 19 (5):1783–91. doi:10.1080/15440478.2020.1788488.
- Luo, Y. Y., Y. Y. Zhang, Y. B. Xu, X. T. Guo, and L. Y. Zhu. 2020. Distribution characteristics and mechanism of microplastics mediated by soil physicochemical properties. Science of the Total Environment 726:138389. doi:10.1016/j.scitotenv.2020.138389.
- Lv, S. S., X. J. Liu, J. Y. Gu, Y. Jiang, H. Y. Tan, and Y. H. Zhang. 2017. Microstructure analysis of polylactic acid-based composites during degradation in soil. International Biodeterioration & Biodegradation 122:53–60. doi:10.1016/j.ibiod.2017.04.017.
- Papong, S., P. Malakul, R. Trungkavashirakun, P. Wenunun, T. Chom-In, M. Nithitanakul, and E. Sarobol. 2014. Comparative assessment of the environmental profile of PLA and PET drinking water bottles from a life cycle perspective. Journal of Cleaner Production 65:539–50. doi:10.1016/j.jclepro.2013.09.030.
- Pattanasuttichonlakul, W., N. Sombatsompop, and B. Prapagdee. 2018. Accelerating biodegradation of PLA using microbial consortium from dairy wastewater sludge combined with PLA-degrading bacterium. International Biodeterioration & Biodegradation 132:74–83. doi:10.1016/j.ibiod.2018.05.014.
- Penkhrue, W., C. Khanongnuch, K. Masaki, W. Pathom-Aree, W. Punyodom, and S. Lumyong. 2015. Isolation and screening of biopolymer-degrading microorganisms from northern Thailand. World Journal of Microbiology & Biotechnology 31 (9):1431–42. doi:10.1007/s11274-015-1895-1.
- Salazar-Sanchez, M. D. R., S. D. Campo-Erazo, H. S. Villada-Castillo, and J. F. Solanilla-Duque. 2019. Structural changes of cassava starch and polylactic acid films submitted to biodegradation process. International Journal of Biological Macromolecules 129:442–47. doi:10.1016/j.ijbiomac.2019.01.187.
- Singhvi, M. S., S. S. Zinjarde, and D. V. Gokhale. 2019. Polylactic acid: Synthesis and biomedical applications. Journal of Applied Microbiology 127 (6):1612–26. doi:10.1111/jam.14290.
- Stepczynska, M., and P. Rytlewski. 2018. Enzymatic degradation of flax-fibers reinforced polylactide. International Biodeterioration & Biodegradation 126:160–66. doi:10.1016/j.ibiod.2017.11.001.
- Turco, R., D. Zannini, S. Mallardo, G. Dal Poggetto, R. Tesser, G. Santagata, M. Malinconico, and M. Di Serio. 2021. Biocomposites based on poly(lactic acid), Cynara Cardunculus seed oil and fibrous presscake: A novel eco-friendly approach to hasten PLA biodegradation in common soil. Polymer Degradation and Stability 188:109576. doi:10.1016/j.polymdegradstab.2021.109576.
- Wang, J. F., J. H. Bai, H. Hua, B. Tang, W. L. Bai, and X. G. Wang. 2022. Characterization and scalable production of industrial hemp fiber filled PLA bio-composites. Journal of Natural Fibers 19 (16):13426–37. doi:10.1080/15440478.2022.2095549.
- Xu, B., Y. P. Chen, J. He, S. X. Cao, J. W. Liu, R. Xue, F. X. Xin, X. J. Qian, J. Zhou, and W. L. Dong. 2022. New insights into the biodegradation of polylactic acid: From degradation to upcycling. Environmental Reviews 30 (1):30–38. doi:10.1139/er-2020-0117.
- Zaaba, N. F., and M. Jaafar. 2020. A review on degradation mechanisms of polylactic acid: Hydrolytic, photodegradative, microbial, and enzymatic degradation. Polymer Engineering and Science 60 (9):2061–75. doi:10.1002/pen.25511.
- Zhang, X. Q., M. Espiritu, A. Bilyk, and L. Kurniawan. 2008. Morphological behaviour of poly(lactic acid) during hydrolytic degradation. Polymer Degradation and Stability 93 (10):1964–70. doi:10.1016/j.polymdegradstab.2008.06.007.