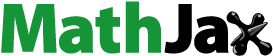
ABSTRACT
Kapok fiber has a large lumen with a natural waxy surface which endows it with distinct hydrophobic-oleophilic properties. Oil-impregnated kapok fibrous powder (KFP) has great potential as a lubricant carrier material. To study the oil wicking mechanism of KFP assemblies with different pore structures (interfibrous pores and the lumens), a dual-scale model was established based on Washburn capillary theory. The theoretical model was verified by capillary rise test of KFP assemblies in various packing densities ranging from 0.08 g/cm3 to 0.14 g/cm3. It was found that the experimental results have good consistency with theoretical values at low packing densities. The deviation between the experimental result and the theoretical value increases with the increase of packing density. This can be largely attributed to compression of the lumens at increased packing density, leading to the reduction of oil sorption capacity within the lumens. The outcomes of this research are believed to provide important references for the usage of fibrous powder materials as lubricant carrier.
摘要
木棉纤维有一个大的管腔和一个天然的蜡状表面,这赋予了它独特的疏水亲油特性. 油浸木棉纤维粉末(KFP)作为润滑剂载体材料具有很大的潜力. 为了研究具有不同孔结构(纤维间孔和管腔)的KFP集合体的吸油机理,基于Washburn毛细管理论建立了双尺度模型. 通过在0.08 g/cm3至0.14 g/cm3的各种填充密度下的KFP集合体的毛细管上升试验验证了理论模型. 结果表明,在低填充密度条件下,实验结果与理论值具有良好的一致性. 实验结果与理论值的偏差随着填料密度的增加而增大. 这在很大程度上可归因于在增加的填充密度下管腔的压缩,导致管腔内的油吸附能力降低. 本研究成果可为纤维粉末材料作为润滑剂载体的应用提供重要参考.
Introduction
Kapok fiber is a lignocellulosic fiber derived from the seedpod of Ceiba pentandra (Hori et al. Citation2000; Zheng, Wang, and Wang Citation2021). It has a large lumen and a natural waxy surface. Due to its unique features, including large hollowness, light in weight, low in density, good buoyancy, and hydrophobic characteristics (Hu et al. Citation2017; Mani, Rayappan, and Bisoyi Citation2012), kapok fiber has opened up possibilities for plenty of application fields. The applications range from stuffing materials (Zhang et al. Citation2013), sound absorption materials (Kassim, Putra, and Ramlan Citation2023; Liu et al. Citation2022), thermal insulation materials (Jabbar et al. Citation2022, Thenmozhi and Thilagavathi Citation2023) and reinforced fiber materials (Bhambure, Rao, and Senthilkumar Citation2023; Chen et al. Citation2022) to oil-absorbing materials (Abdullah, Rahmah, and Man Citation2010) and functional template materials (Cao et al. Citation2018; Xu et al. Citation2018). Among them, due to the excellent hydrophobic-oleophilic performance, various research studies on the use of kapok fiber as sorbent oil spill clean-up and oil-water separation were reported (Dong, Cao, and Xu Citation2017; Lim and Huang Citation2007; Thilagavathi, Praba Karan, and Thenmozhi Citation2020).
The sorption between oil and kapok fiber is primarily based on physical adsorption, which makes it reversible in nature. Meanwhile, the fiber assembly contains abundant capillary channels, which give it great potential for oil release (Xu et al. Citation2020). Therefore, kapok fiber has the necessary properties to serve as a lubricant carrier material. Lubricant usually needs to be pushed to the designated sites (commonly bearings) by mechanical pressing (Gordon, Gainer, and Russell Citation1978; Abel Citation1967). The powdered material facilitates transmission and can be filled into any shape, whereas long fiber is prone to tangling and clogging. The working mechanism of oil-impregnated fibrous powder in lubrication is as follows. When machine is in operation with bearing rotation, the oil-impregnated fibrous powder material in contact with the bearing surface will release the oil through capillary action. This release occurs due to contact friction and local temperature rise resulting from the heat generated by rotational friction. When the machine is not in operation, the fibrous powder material can store oil and prevent oil leakage. According to its working mechanism, studying the oil sorption characteristics and mechanism of fibrous powder assemblies is essential for understanding the roles of fibrous powder as a lubricant carrier material. Our group has conducted research on the oil sorption properties of natural cellulose powder and the oil sorption/release properties of oil-containing KFP under different temperature conditions (Xu et al. Citation2020, Citation2022). However, there is still a lack of research on the oil sorption mechanism of fibrous powders.
In this study, the oil wicking mechanism of KFP assembly was studied. Based on the pore characteristics of KFP assembly, a dual-scale model was established to analyze the oil sorption mechanism. To validate the theoretical model, the oil sorption coefficient values obtained from capillary rise tests were compared with theoretical values. The outcomes of this research are believed to provide important reference for the usage of fibrous powder materials as lubricant carrier.
Construction of oil sorption theoretical model
To study the role of pores between fibrous powders and the lumen structure of kapok fiber itself in the process of oil sorption, a two-scale model was constructed, as shown in . The model is based on the classical capillary theory, where the fibers are represented as numerous equivalent cylindrical capillary components, and the driving force for liquid transport is from the capillary pressure (Washburn Citation1921).
There are three basic hypotheses:
It is assumed that all KFP has the same length and diameter and is arranged in a parallel manner to each other.
It is assumed that the lumens of the fibrous powder assemble a bundle of parallel capillaries, with the diameter of the capillaries being the same as that of lumen.
It is assumed that the interfibrous pores are also a bundle of parallel capillaries, with their diameters represented by equivalent hydrostatic diameters. Furthermore, the wetting region of the capillary assembly is equivalent to the wetting region of the interfibrous pores, and that the porosity of the capillary assembly is the same as that of the interfibrous pores.
From hypothesis (2) it can be inferred that:
where represents the capillary diameter of lumen, and
represents the inner diameter of kapok fiber.
In order to calculate the equivalent capillary diameter between KFP, the following equation can be used based on the packing density “” of the fibrous powder assembly, the volume of the entire fibrous powder (including the lumens)
and the volume of the kapok fiber wall
at the given packing density of the fibrous powder assembly can be expressed by the following equation:
where and
represent the kapok fiber density and the density of fiber wall, respectively. The porosity
of the fibrous powder assembly, the porosity
between the interfibrous powder and the porosity
of kapok fiber itself can be expressed by the following equation:
Using to represent the number of interfibrous capillaries,
represent the corresponding hydrostatic diameter, the following relation can be derived according to hypothesis (3):
where represents the specific surface area of the fibrous powder material, which can also be expressed by the following relation:
where represents the outer diameter of kapok fiber, and the hydrostatic diameter can be obtained by combining formulas (7), (8) and (9):
According to Washburn equation, for a capillary with a diameter of , the relationship between the wicking length
and the wicking time
is as follows:
where represents the surface tension of the liquid,
represents the contact angle between solid and liquid, and
represents the viscosity of the liquid. Besides, the wicking height can also be expressed by the wicking mass:
where, represents the wicking mass,
represents the cross-sectional area of the filling medium,
represents the porosity of the filling medium, and
represents the density of the liquid. Therefore, by combining equations (10), (11) and (12), we can obtain the mass of liquid entering the fiber lumens during the capillary rise test
and the mass of liquid entering the interfibrous pores
, respectively:
The ratio between the mass square of liquid and the corresponding time is defined as sorption coefficient (Nishi et al. Citation2002). Therefore, the sorption coefficient of the fiber lumens and the sorption coefficient of the interfibrous pores
are:
For kapok fiber:
where and
are the outer diameter and inner diameter of kapok fiber, respectively,
is the density of kapok fiber,
is the density of kapok fiber wall,
is the linear density of kapok fiber,
and
represent the cross-sectional area of the lumen and kapok fiber, respectively. The basic structural parameters of kapok fiber are shown in .
Table 1. Structural parameters of kapok fiber.
Materials and methods
Materials
Kapok fiber used in the study was from Pate County, Java Tengah, Indonesia. Fibrous powder was obtained by mechanical cutting using fiber cutting machine (SBJ800E, Qinghang, China). The morphological structure of fiber powder was examined using scanning electron microscope (SEM-TM3000, Hitachi, Japan). As shown in , the length range of KFP varied from dozens of microns to 2 mm. Kapok fiber exhibited a hollow structure and smooth surface.
Test oils are two commonly used lubricants, mineral oil and Polyalphaolefin (PAO) synthetic oil. Mineral oil, also known as liquid paraffin, which is primarily composed of alkanes, is extracted from crude oil. PAO is made by polymerization of ethylene to produce alpha olefin, which is then further polymerized and hydrogenated. Oil density was tested using a dynamic Tensiometer (DCAT11, Dataphysics, Germany). The viscosity of oil was tested using an intelligent viscosity measuring instrument (DV2TLV, Brookfield, USA). The instrument is a digital display viscometer, which is driven by a motor to rotate the rotor at constant speed. When the rotor rotates in the liquid, the liquid will generate a torque due to its viscosity, which acts on the rotation of the rotor. The viscous torque acting on the rotor is detected by the sensor, and the viscosity of the liquid is obtained through computer processing. Appropriate rotor and rotation speed should be set according to the viscosity of the test liquid. For the test oils, rotor No. SC4–18 (18) was used, and the rotation speed was set at 200 rpm. The tests were conducted at 20 ± 2°C. The oil properties, including density and viscosity, are listed in .
Table 2. Basic properties of test oils at 20°C.
Capillary rise test
Capillary rise test was conducted to measure the wicking behavior of KFP. The instrument was dynamic tensiometer (DCAT11, Dataphysics, Germany), and a schematic of the instrument can be found in . A cylindrical tube was used as sample tube 3. The inner diameter of the sample tube is 12 mm, and the filling length is 20 mm. Hence, the volume of the sample tube that can be filled is constant (2.26 cm3). Various packing densities of the KFP were achieved by uniformly filling different mass of fiber materials into the tube, and then compressing the piston of the tube to a filled length. The sample preparation process involves filling the sample into the sample tube as evenly as possible to ensure the accuracy of the test results and the repeatability of the test.
Figure 3. Schematic diagram of Tensiometer device. 1 – electronic balance, 2 – sample holder, 3 – sample tube, 4 – oil, 5 – liquid reservoir, 6 – lifting platform.

During the test, the oil in liquid reservoir 5 was elevated at a speed of 0.1 mm/s until it contacted the KFP material. The change in mass over time was accurately detected by the electronic balance 1 and recorded on a computer. To ensure the repeatability and reproducibility of the results, three samples were prepared for each test; therefore, the average values along with standard deviations were obtained. The tests were conducted at 20 ± 2°C.
Results and discussion
Oil sorption behavior analysis
shows the relationship between the square of sorption mass and time at various packing densities. As can be seen from , the sorption process can be divided into two stages. At the beginning of sorption, oil entered the sample at a fast speed, and the sorption mass increased with time. After the sorption equilibrium was reached, the amount of oil entering the porous material no longer changed with time. After 1000 s and 500 s, the sorption of mineral oil and PAO synthetic oil reach sorption equilibrium, respectively. The lower the packing density of the material, the faster the sorption occurs, and the quicker the sorption equilibrium was reached. It is mainly attributed to the smaller the packing density, which creates a larger space inside the material, making it easier for the oil to penetrate into the porous material. For two different test oils, it took more time for the sorption of mineral oil to reach equilibrium under the same volume density condition. It is mainly due to the higher viscosity of mineral oil (74.7 mPa · s) compared to that of PAO synthetic oil (29.55 mPa · s).
Figure 4. Relationship between the square of oil sorption mass and time (a) mineral oil, and (b) PAO synthetic oil. Solid line represents the linear fitting at the initial stage.

To further analyze the wicking behavior of KFP under different packing densities, the saturated oil sorption capacity (M0) and sorption coefficient (D0) were obtained according to the capillary rise test. M0 was obtained by the mass of oil sorption at equilibrium. D0 was obtained by performing linear fitting of the square of the sorption mass at the initial stage over time. The linear fits of initial sorption period are depicted in , with all correlation coefficients R2 >0.98. presents the relationship between saturated oil sorption and sorption coefficient with respect to packing density. As can be seen from , with the increase of the packing density, M0 decreased linearly. The reason was that with the increase of packing density, the pores between fibrous powders decreased, resulting in the space available for oil sorption decreased. For different types of oil, M0 of mineral oil was slightly higher than that of PAO synthetic oil at the same packing density. It is mainly attributed to the oil density of mineral oil (0.85 g/cm3) was slightly higher than that of PAO synthetic oil (0.82 g/cm3). When the oil sorption volume was fixed, denser oil had greater mass. As can be seen from , decreased exponentially with the increase of the packing density, resulting from the increased flow resistance to oil. Under the same packing density, the
of mineral oil was significantly higher than that of PAO synthetic oil. Viscosity of oil was a key factor affecting the sorption speed. The higher the viscosity, the greater the viscosity resistance, the lower the fluidity, and the lower the sorption coefficient. At 20°C, the viscosity of mineral oil (74.73 mPa · s) was much higher than that of PAO synthetic oil (29.55 mPa · s). Therefore, the sorption coefficient of mineral oil was lower than that of PAO synthetic oil.
Oil sorption mechanism analysis
According to the theoretical formula of the two-scale model and the relevant parameters of kapok fiber itself, the theoretical values of the sorption coefficient of the interfibrous pores () and the sorption coefficient of the fiber lumens (
) can be calculated. The sum of the two is the total sorption coefficient (
). The model was verified by comparing the oil sorption coefficients obtained from the capillary rise test and their theoretical values, and the specific values are shown in .
Table 3. Experimental and theoretical values of sorption coefficient (mineral oil).
shows the comparison of theoretical and experimental values of sorption coefficients. As can be seen from , the experimental sorption coefficient values of the two test oils were in good agreement with the theoretical values of sorption coefficient derived from the two-scale model theory at the packing densities of 0.08 g/cm3 and 0.10 g/cm3, with a deviation of less than 6%. In other words, its sorption characteristics conformed to the predications of the two-scale oil sorption model theory. When the packing densities increased to 0.12 g/cm3 and 0.14 g/cm3, the deviation increased obviously to 15–20%. This indicates that its adaptability to the two-scale model declined sharply under these packing densities. It is largely attributed to part of the lumens of the fibrous powder squashed to different degrees for compact assembly, the volume of the fibrous powder itself became smaller. It resulted in an increase in the porosity of the interfibrous powders, and the porosity of the fibrous powder itself decreased. According to formula (15) and (16), the value of increases and the value of
decreases. For oil sorption of KFP assembly, the pores between powders played a dominant role in oil sorption, while the contribution of the internal lumen of fibrous powder to oil adsorption was secondary. The increase of
was much greater than the decrease of
. Therefore, the overall sorption coefficient of KFP material was larger compared to the theoretical value. Researchers reported a dual-scale model to study the relationship between the dual-scale pore structure (primary pores and secondary pores) of kapok/microfibrillated cellulose aerogels (Zhang et al. Citation2021). It was found that the experimental values of the porous aerogels with varying ratios of kapok and microfibrillated cellulose showed good consistency with theoretical values. However, the effect of packing density on porous aerogels was not considered.
Figure 6. Theoretical and experimental values of sorption coefficient (a) mineral oil, and (b) PAO synthetic oil.

From the above discussion, it can be concluded that for loose KFP assembly (packing density less than 0.10 g/cm3), the established dual-scale theory model could accurately describe the oil sorption behavior. Therefore, according to formula (13) and (14), the amount of oil entering the interfibrous pores and lumens can be quantitatively characterized, as shown in . shows the oil distribution in the lumens and interfibrous pores of kapok fibrous.
Figure 7. Oil sorption in lumens and interfibrous pores of KFP, represents oil sorption in lumens, and
represents oil sorption in interfibrous pores.

Table 4. Experimental and theoretical values of sorption coefficient (PAO synthetic oil).
Table 5. Theoretical values of oil sorption by interfibrous pores of KFP and kapok lumens.
This study provides valuable insights into the practical application of the findings. The results indicate that the oil sorption behavior of KFP assemblies is strongly influenced by factors such as oil density, viscosity, and packing density. The threshold packing density of 0.10 g/cm3 was identified as a crucial parameter for the potential use of KFP as a lubricant carrier. Below this threshold, KFP assembly exhibits excellent oil sorption ability and can serve as an effective potential carrier material. However, as the packing density exceeds this value, the effectiveness of KFP as a lubricant carrier diminishes significantly. These findings have important implications for the design and selection of lubricant carrier materials, highlighting the need to consider the packing density and other factors that impact the oil sorption behavior. By understanding these factors, engineers and researchers can optimize the performance of lubricant carriers for various applications, leading to enhanced efficiency and effectiveness in lubrication systems.
While this study has provided valuable insights into the oil sorption behavior of KFP assemblies, there are certain limitations that should be acknowledged. First, the investigation focused on a specific range of particle sizes for the KFP assemblies. The impact of broader particle size ranges on the oil sorption mechanism remains an area requiring further exploration. Additionally, the influence of temperature on the properties of oil and the interaction between oil and fibrous porous materials was not considered in this study. Future research should aim to investigate the oil sorption mechanism of KFP assemblies with broader particle size ranges and explore the effect of temperature on the oil sorption behavior. These investigations will provide a more comprehensive understanding of the oil sorption process and contribute to the development of optimized lubricant carrier materials.
Conclusion
In summary, a dual-scale model was developed based on the Washburn capillary theory to investigate the oil wicking mechanism of KFP assembly. Capillary rise tests were employed to verify the applicability of the model, and the results demonstrated that the experimental sorption coefficient values were in good agreement with the theoretical values at low packing densities (0.08 ~ 0.10 g/cm3). This finding confirms the high applicability of the model in describing the oil wicking behavior of KFP assembly. However, as the packing density increases, there is an observed increase in the deviation between the experimental results and the theoretical values. This can be largely attributed to the compression of the lumens with increased packing density, which reduces the oil sorption capacity into lumens, and the inferior applicability of dual-scale model. It is worth noting that a packing density of 0.10 g/cm3 was identified as the threshold value for KFP, beyond which its potential as a lubricant carrier is not recommended. The proposed oil sorption model is significant in studying the relationship of pore structure and oil sorption of KFP and other porous materials, which could provide valuable insights for their practical applications.
Highlights
Oil-impregnated kapok fibrous powder (KFP) as a potential lubricant carrier material was proposed.
According to the characteristics of KFP assembly, a dual-scale model was established based on Washburn capillary theory.
The packing density of 0.01 g/cm3 was proposed as threshold value for KFP assembly used as a potential lubricant carrier.
Acknowledgements
The authors received no financial support for the research, authorship, and/or publication of this article.
Disclosure statement
No potential conflict of interest was reported by the author(s).
References
- Abdullah, M. A., A. U. Rahmah, and Z. Man. 2010. Physicochemical and sorption characteristics of Malaysian Ceiba pentandra (L.) Gaertn. as a natural oil sorbent. Journal of Hazardous Materials 177 (1–3):683–12. doi:10.1016/j.jhazmat.2009.12.085.
- Abel, M. L. 1967. Method of making oil-impregnated wicking material for bearing. U.S. Patent No.3359614
- Bhambure, S. S., A. S. Rao, and T. Senthilkumar. 2023. Analysis of mechanical properties of kenaf and kapok fiber reinforced hybrid polyester composite. Journal of Natural Fibers 20 (1):1–10. doi:10.1080/15440478.2022.2156964.
- Cao, Y., L. Xie, G. Sun, F. Su, Q.-Q. Kong, F. Li, W. Ma, J. Shi, D. Jiang, C. Lu, et al. 2018. Hollow carbon microtubes from kapok fiber: Structural evolution and energy storage performance. Sustainable Energy & Fuels 2 (2):455–65. doi:10.1039/c7se00481h.
- Chen, X., L. Gu, C. Dang, and X. Cao. 2022. A lightweight and high-strength epoxy composites based on graphene oxide modified kapok fibers. Composites Communications 31:31. doi:10.1016/j.coco.2022.101111.
- Dong, T., S. Cao, and G. Xu. 2017. Highly efficient and recyclable depth filtrating system using structured kapok filters for oil removal and recovery from wastewater. Journal of Hazardous Materials 321:859–67. doi:10.1016/j.jhazmat.2016.10.005.
- Gordon, C., P. H. Gainer, and M. L. Russell 1978. Extrudable lubricating wicking material. U. S. Patent No.40099805
- Hori, K., M. E. Flavier, S. Kuga, T. B. T. Lam, and K. Iiyama. 2000. Excellent oil absorbent kapok Ceiba pentandra (L.) Gaertn. fiber: Fiber structure, chemical characteristics, and application. Journal of Wood Science 46:401–04. doi:10.1007/BF00776404.
- Hu, L., F. Wang, G. Xu, and B. Xu. 2017. Unique microstructure of kapok fibers in longitudinal microscopic images. Textile Research Journal 87 (18):2255–62. doi:10.1177/0040517516673334.
- Jabbar, A., M. A. Ali, A. Shahzad, M. S. Naeem, Z. Javed, M. B. Qadir, K. Rehman, M. Irfan, and Z. Ahmad. 2022. Development of kapok/recycled-PET blended needle-punched thermal waddings. Journal of Natural Fiber 19 (3):1024–32. doi:10.1080/15440478.2020.1784816.
- Kassim, D. H., A. Putra, and R. Ramlan. 2023. Enhancement of sound absorption of coir fiber using thin layer of kapok fibers. Journal of Natural Fibers 20 (1):1. doi:10.1080/15440478.2022.2164103.
- Lim, T.-T., and X. Huang. 2007. Evaluation of kapok (Ceiba pentandra (L.) Gaertn.) as a natural hollow hydrophobic-oleophilic fibrous sorbent for oil spill cleanup. Chemosphere 66 (5):955–63. doi:10.1016/j.chemosphere.2006.05.062.
- Liu, X., J. Xu, Y. Zhu, W. Ke, Z. Deng, and X. Tang. 2022. Sound absorption characteristics of agro-sourced kapok fibrous materials. Industrial Crops and Products 188:188. doi:10.1016/j.indcrop.2022.115661.
- Mani, G. K., J. B. B. Rayappan, and D. K. Bisoyi. 2012. Synthesis and characterization of kapok fibers and its composites. Journal of Applied Sciences 12 (16):1661–65. doi:10.3923/jas.2012.1661.1665.
- Nishi, Y., N. Iwashita, Y. Sawada, and M. Inagaki. 2002. Sorption kinetics of heavy oil into porous carbons. Water Research 36 (20):5029–36. doi:10.1016/S0043-1354(02)00225-7.
- Thenmozhi, R., and G. Thilagavathi. 2023. Investigations on kapok/polypropylene needle punched nonwoven for thermal resistance applications. Journal of Natural Fibers 20 (1):1. doi:10.1080/15440478.2022.2146247.
- Thilagavathi, G., C. Praba Karan, and R. Thenmozhi. 2020. Development and investigations of kapok fiber based needle punched nonwoven as eco-friendly oil sorbent. Journal of Natural Fibers 17 (1):18–27. doi:10.1080/15440478.2018.1458686.
- Washburn, E. W. 1921. The dynamics of capillary flow. Physical Review 17 (3):273–83. doi:10.1103/PhysRev.17.273.
- Xu, Y., L. Cao, H. Shen, and G. Xu. 2022. Temperature effect on oil sorption and wettability of kapok Fiber. Journal of Natural Fibers 19 (7):2555–66. doi:10.1080/15440478.2020.1820931.
- Xu, W., B. Mu, X. An, and A. Wang. 2018. Bio-template synthesis of three-dimensional microtubular nickel-cobalt layered double hydroxide composites for energy storage. Cellulose 25 (7):4121–31. doi:10.1007/s10570-018-1849-5.
- Xu, Y., H. Shen, L. Cao, and G. Xu. 2020. Oil release behavior and kinetics of oil-impregnated kapok fiber powder. Cellulose 27 (10):5845–53. doi:10.1007/s10570-020-03169-8.
- Zhang, X., W. Fu, C. Duan, H. Xiao, M. Shi, N. Zhao, and J. Xu. 2013. Superhydrophobicity determines the buoyancy performance of kapok fiber aggregates. Applied Surface Science 266 (FEB.1):225–29. doi:10.1016/j.apsusc.2012.11.153.
- Zhang, H., G. Xu, W. Fu, S. Su, J. Wang, and H. Shen. 2021. A theoretical and experimental study of oil wicking behavior via ‘‘green’’ superabsorbent. Cellulose 28 (16):10517–29. doi:10.1007/s10570-021-04218-6.
- Zheng, Y., J. Wang, and A. Wang. 2021. Recent advances in the potential applications of hollow kapok fiber-based functional materials. Cellulose 28 (9):5269–92. doi:10.1007/s10570-021-03834-6.