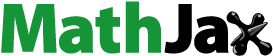
ABSTRACT
This work is focused on characterization and comparative study of agave americana and agave sisalana leaf fibers which can be used as potential alternative for synthetic fibers in engineering applications. Physico-chemical and mechanical properties of agave fibers were determined using different analytical procedures and instruments. According to the experimental data, the mean length and diameter of agave americana fibers were maximum of 1.76 m and 411 μm, respectively. Furthermore, the particular fibers possess larger amount of hemicellulose (17.4%) and moisture (~8%) which confirmed they are relatively hydrophilic in nature. Conversely, agave sisalana fibers possess larger amount of cellulose (66.4%) and ligni10.1080/10942912.2023.2246677n (~16%) compared to agave americana fibers. Meanwhile, SEM microstructure also proved surface of agave americana fibers was comparably rough, full of cracks and linings. EDAX analysis showed presence of significant amount of C and O, including trace amount of Ca, K, Si and S. Similarly, the semicrystalline agave americana fibers are longer and thicker possessing greater mean linear density (29.68 Tex). Agave sisalana fibers recorded maximum breaking tensile strength and elongation at break (512 MPa) and (~6%), respectively. These findings strongly suggested that agave fibers possess interesting features which can be used as potential reinforcement fibers for composite engineering.
本工作主要对美洲龙舌兰和剑麻叶纤维进行了表征和比较研究,这两种纤维可作为工程应用中合成纤维的潜在替代品. 采用不同的分析方法和仪器测定了龙舌兰纤维的理化性能和力学性能. 根据实验数据,美洲龙舌兰纤维的平均长度和直径分别为1.76 m和411 μm. 此外,特定纤维具有更大量的半纤维素(17.4%)和水分(~8%),这证实了它们在性质上是相对亲水的. 相反,与美洲龙舌兰纤维相比,剑麻纤维含有更多的纤维素(66.4%)和木质素(~16%). 同时,扫描电镜微观结构也证实了美洲龙舌兰纤维表面比较粗糙,充满了裂纹和衬里. EDAX分析显示存在大量的C和O,包括微量的Ca、K、Si和S. 类似地,半结晶美洲龙舌兰纤维更长、更厚,具有更大的平均线密度(29.68Tex). 剑麻纤维的最大断裂抗拉强度和断裂伸长率分别为(512MPa)和(~6%). 这些发现有力地表明,龙舌兰纤维具有有趣的特性,可作为复合材料工程的潜在增强纤维.
Introduction
Synthetic fibers (such as aramid, glass and carbon fibers) have been widely used as reinforcement in engineering composites replacing metallic and ceramic materials. However, they are nonrenewable and endanger the environment posing health risks during production (Pappua et al. Citation2016). Natural fibers have attracted considerable attention of scientists and industrialists for their wonderful characteristics, including light weight, biorenewable and locally available (Teklu Citation2021) with comparable strength. Moreover, processing of natural fibers is energetically viable and economically reasonable. However, natural fibers are usually used in low-performance applications, namely as automotive manufacturing and construction industry (Placet et al. Citation2012). Thus, it is inevitable to understand the micro-mechanical behavior of natural fibers for durable applications in high-performance arenas such as aerospace, marine, hostile thermal and chemical conditions (Periyasamy Citation2021).
Though agaves are native to Mexico, they are cultivated dominantly in tropical and sub-tropical regions all over the world (Debnath et al. Citation2010). Among the Agavaceae family, agave americana and agave sisalana plants are the most abundant varieties (Ortega et al. Citation2019) () and comfortably grow in Ethiopia. They are common plants which grow along roadsides, fence for farm lands and even in abandoned farming areas. They are potentially used as source of fibers in the form of rope and cordage (Asfaw Citation2011) and homogenously available in the local markets. These fibers possess moderate mechanical property, high purity, low molecular weight and resistance to deterioration in brackish water (Gurram and Rao Citation2016).
Agave fibers possess high variability in physico-chemical and mechanical properties (such as diameter, length and strength) even within the same leaf. Though they are susceptible to variations emanating from origin, climate conditions, soil properties, maturity of the leaf and extraction techniques (Mansouri et al. Citation2020), people mistakenly used them synonymously. However, properties and performances of technical materials depend on characteristics of constituent fibers (Haque et al. Citation2015). Moreover, agave fibers are hygroscopic in nature which display high affinity toward moisture (~9% moisture regain) and water (Oladele, Omotoyinbo, and Adewara Citation2010). Such shortcomings can hinder their scope, versatility, and multidisciplinary applications of materials.
Method of extraction greatly affects performance of agave fibers. The method used can alter appearance, composition of constituents or structural features while others can lessen the strength; thereby reducing the life time of technical materials (Teklu Citation2021). Extraction can be achieved through retting (soil and water), chemical and mechanical process though all methods show certain shortcomings (Msahli et al. Citation2015). Soil retting is a well-practiced method of extraction of cellulose fibers by using microorganisms from lignocellulosic biomass leading to better mechanical properties (Debnath et al. Citation2010). However, soil retting should be cautiously optimized and monitored (including; depth, number of days, amount of moisture and weather conditions) to avoid excessive degradation of cellulosic materials which can further reduce quality and strength of the fiber (Ebisike et al. Citation2013).
Despite a lot of research was conducted on separate characters of agave americana or agave sisalana fibers envisioned for different applications, an exclusive work remains to understand similarities and variations on physico-chemical, structural and mechanical features of both types of leaf fibers. Therefore, the main goal of the current research was to investigate and compare their aforementioned properties.
Materials and methods
Chemicals
The following analytical grade reagents were used without further purification: sodium hydroxide (99%), sulfuric acid (99%), nitric acid (67.1%), acetic acid, ammonia, ammonium oxalate, ethanol (99%) and sodium chlorite obtained from Merck Chemical Co. (Germany) through local supplier.
Experimental methods
Matured agave (agave americana and agave sisalana) leaves were separately collected from the Mountain of Endayesus, Ethiopia. After the sharp edges were removed, agave leaves were buried about 50 cm to the ground for 21 days (Oladele and Agbabiaka Citation2015; Teklu Citation2021). The fibers were washed in warm water (~60°C) followed by distilled water three times to remove residues leftover the extraction process. The fibers were dried in open shade for two weeks.
Determination of physico-chemical properties
Fifty fibers were randomly selected from each sample and flattened over a meter ruler to estimate their mean length (in meter). The values were noted in meter. In characterizing fiber fineness, linear density was measured (in terms of weight per unit length) using the gravimetric method according to ASTM D1577–79 (Msahli et al. Citation2007). So, individual length of 50 fibers were measured and weighed; the linear density of each sample was calculated using the following formula (EquationEquation 1(1)
(1) ):
Fibres were photographed using optical microscope which is fitted with a high-resolution Leica camera, which is computerized with “Mesdan Image Analyser,” allowing capture longitudinal direction images (Teklu, Wangatia, and Alemayehu Citation2019). The mean diameter was an average of 50 measurements for each type randomly taken at four locations along the fiber length.
In addition, moisture content was measured in compliance with the TAPPI T 212 om-02 standard. 5 g of fiber sample was taken under the standard atmospheric conditions for testing fibers at 202°C and 65
2% relative humidity. These samples were placed in an oven at 105°C and weighed after 15 minutes interval until 0.1% less change in weight recorded between successive measurements. Moisture content was calculated by using the following formula (EquationEquation 2
(2)
(2) ) (Kestur et al. Citation2013):
Where W1 and W2 denote the weight before oven dry and after oven dry in grams.
Agave fibers are chemically composed of lignin, pectin, hemicellulose and cellulose which gave coarse, rough and irregular surface. Therefore, these chemical components were analyzed; the amount of lignin was determined in compliance with the TAPPI T 222 os-76 standard (Amel et al. Citation2013) by dissolving 2 g of agave fibers (W3) in 80% sulfuric acid for 24 hrs by stirring gently. Then, 150 mL of distilled water was added to the content and boiled for one hr before the solution was cooled in desiccator. Finally, lignin was filtered, collected and weighed (W4). The amount of lignin was calculated using the following formula (EquationEquation 3(3)
(3) ).
Where, W3 is the mass of agave fibers reserved for analysis while W4 represents weight after dissolution.
Similarly, the amount of pectin was determined by dissolving in ammonium oxalate solution. Closely, 2 g of agave fibers (W5 was boiled for 3 hrs in sufficient amount of ammonium oxalate solution. The residue was left in desiccator for 30 minutes and weighed (W6). The amount of pectin was calculated using the following formula (EquationEquation 4(4)
(4) ) (Mbugua Citation2014):
Where, W5 is the amount of agave fibers taken for analysis while W6 represents weight after boil.
Likewise, the amount of cellulose which exists within the fibers is determined by using acidified sodium chlorite solution. The method works based on the insolubility of cellulose in aqueous solution and tolerating the action of acids and bases. A fixed amount of crushed fibers (W7) was mixed with 1.72% sodium chlorite; subjected to acidification with few drops of sulfuric acid. It was, then, refluxed for one hr. Ammonia was added to the resulting concoction solution and washed repeatedly using distilled water. Finally, the residue material was oven dried and weighed (W8) (Mylsamy and Rajendran Citation2010). The amount of cellulose was determined using the below equation (EquationEquation 5(5)
(5) ):
Where, W7 is the weight of agave fibers considered for analysis while W8 represents dried weight after chemical degradation.
Finally, the amount of hemicellulose was analyzed by dissolving agave fibers in sodium hydroxide solution in such a way that 2 g of agave fibers (W9) was boiled in sodium hydroxide solution for 3 hrs. The remaining part was transferred to desiccator for 30 minutes and weighed (W10). The amount of hemicellulose was computed using the following formula (EquationEquation 6(6)
(6) ) (Mbugua Citation2014):
Where, W9 is the weight of agave fibers considered for analysis while W10 represents weight after boil. All the measured values are reported as mean ± standard deviation (SD).
Instrumental analysis
Agave fibers were characterized by using Fourier transform infrared (FT-IR) spectroscopy to study the chemical functionalities via KBr disk in a spectral range of 4000 cm−1 to 400 cm−1. To measure the weight loss and heat flow as a function of temperature, the specimen was subjected to thermal analysis on the simultaneous thermal analyzer (DSC-TGA SDT-Q600) from 25°C to 600°C at a continuous nitrogen flow of 20 mL/min and a heating rate of 20°C/min. Furthermore, surface microstructures were examined by scanning electron microscope, SEM (JSM-IT300LV, JEOL, USA) at 500 × magnifications.
In understanding the fibers’ crystalline nature, X-ray diffraction (XRD) study was performed under ambient condition on X-ray diffractometer (XRD-7000, Shimadzu) equipped with a Cu-Kα (λ = 1.5418 Ǻ) monochromatic radiation with 40.0 kV and 30 mA on rotation between 10° to 80° at 2θ scale. Their crystallinity index (CI), degree of crystallinity, was calculated using Segal equation (EquationEquation 7(7)
(7) ) (Segal et al. Citation1959).
Where and
belong to the crystalline part and amorphous form at 2θ scale, respectively.
Mechanical properties
The breaking tensile strength of agave fibers was measured according to ASTM D 3822–07 at room temperature using Tinius Olsen H10KS single fiber strength testing machine, with crosshead speed of 5 mm/min and original test length of 25 mm at 68 ± 3% relative humidity (Teklu, Wangatia, and Alemayehu Citation2019). Furthermore, elongation at break could be evaluated by considering the increment of a test length up on application of a tensile force (EquationEquation 8(8)
(8) ).
Wherebelong to the original test length and change in test length of the fiber after application of the external load, respectively.
Results and discussion
Physico-chemical properties of agave fibers
Fiber length and diameter are essential parameters used to compare diverse variation of natural fibers and select for specific applications. In this regard, Agaves, considered for this study, have produced very long fibers which fall in the range of 1.43 m to 1.76 m; all registered lengths are beyond the factory standard, ≥ 90 cm (Srinivasakumar et al. Citation2013). Such findings of the present study are congruent to previously reported values from the same region which lay in the range of 1.22 m to 1.78 m (Asfaw Citation2011).
Meanwhile, the mean diameter of agave americana and agave sisalana fibers is 411 µm and 367 µm, respectively. It is noted that the mean diameter of agave americana fibers is comparably larger than literature values reported from Tunisia (~263 µm) (Msahli, Sakli, and Drean Citation2006). shows the diameter measurement and microscopic image of agave fibers measured by “Mesdan Image Analyser” software. Due to variations in environment and local conditions, fiber diameter is not uniform throughout a given length which limits versatility of natural fibers (Hulle, Kadole, and Katkar Citation2015).
Figure 2. The optical microscopic image of (a) agave americana fibers and (b) agave sisalana leaf fibers.

Agaves like other natural fibers are naturally occurring composites which contain wax, pectin, lignin, hemicellulose and cellulose. Experimental results revealed that about 90% of both types of fibers are composed of lignin, hemicellulose and cellulose (), which are in line with report of elsewhere (Karimah et al. Citation2021). Of course, composition of each chemical constituent varied from one species to another. Agave sisalana fibers possess greater amount of cellulose (66.44%) compared to agave americana fibers (59.5%). Conversely, agave americana fibers showed greater amount of hemicellulose compared to agave sisalana fibers (17.4% versus 10.9%). Different scholars suggested that proportion of chemical composition determines property and performance of a material, specially bond interactions and strength (Bai et al. Citation2002).
Table 1. Physico-chemical properties of agave americana and agave sisalana fibers extracted by soil retting (Mean ±SD, n = 3).
Nevertheless, due to discrepancies in the origin of the leaf, maturity of the plant, source and climatic conditions, and method of extraction, comparing the chemical composition proportion of agave fibers with reported values are challenging (Mbugua, Tumuti, and Nguku Citation2021; Razali et al. Citation2015). Evidently, published research outputs have commonly shown variations in chemical compositions. However, the experimental values for lignin, hemicellulose and cellulose are either approximately same or lay in the range of literature values ().
Table 2. Comparison of physico-chemical properties of agave americana and agave sisalana fibers.
Meanwhile, moisture content is an important parameter in textile industry which requires special attention while selecting a fiber for specific application. Fibers which absorb too much amount of moisture are designated as hygroscopic. From a practical point of view, moisture assists degradation of chemical entities which can weaken mechanical strength, thereby shortening the life span of engineered materials (Razali et al. Citation2015). Agave americana fibers possess larger amount of moisture (7.94%) relative to its counterpart, sisal fibers (5.31%). In open literatures, it is stated that a fiber with large amount of hemicellulose shows greater affinity toward moisture absorption. Consequently, it paves the way for further thermal and biological degradation (Amior et al. Citation2023).
FT-IR spectroscopy
FT-IR spectroscopy is a powerful tool that ensures functional groups based on chemical changes in vibrational frequencies. The FT-IR spectra () of agave americana and agave sisalana fibers possess several bands in common. The peak around 3500 cm−1 is characteristic to the O-H stretching vibration of hydrogen bonds originated from cellulose and allied chemical entities, and moisture (Hajiha, Sain, and Mei Citation2014). However, agave sisalana () showed a little broader and stronger peak which might be due to the nature and interaction of H bonding. A very weak band at 2900 cm−1 represents the C-H symmetric stretching vibration of aliphatic hydrocarbons. A strong absorption peak at 1730 cm−1 represents carbonyl (C=O) stretching vibration of acetyl functionalities from pectin and waxes (Jasti and Biswas Citation2023) with a slight variation in peak intensity between the species. Another broader and stronger peak which appeared at 1200 cm−1 belongs to the C-O stretching vibration of aryl groups derived from hemicellulose, lignin and cellulose (Kim et al. Citation2013).
Thermal analysis (DSC-TGA)
Thermal properties of fibers are dependent on interactions and chemical compositions. In evaluating moisture and degradation of chemical entities in the fibers, thermal analysis was conducted for both fibers and their differential scanning calorimetry (DSC) and thermogravimetric analysis (TGA) are depicted in . The first endothermic stage which extends up to 107°C inferred to mass loss due to evolution of adsorbed moisture (Ganan et al. Citation2005) which accounts very small portion (~6%) from the total weight.
The second endothermic stage which ranges from 180°C to 400°C comprises a mass loss due to depolymerization and cleavage of glycosidic linkage of lignin, hemicellulose and cellulose (Madera-Santana, Valdez, and Richardson Citation2013) which accounts approximately 60% of the total weight lost during the process. The residue leftover in the form of char at 600°C was significantly greater for agave sisalana fibers (~33%) compared to agave americana fibers (~25%). The greater amount of char residue for agave sisalana fibers may be resulted from condensation of lignin which subsequently formed aromatic hydrocarbons with little variation in thermal stability (Martin et al. Citation2010).
XRD analysis
XRD studies of agave americana and agave sisalana fibers were carried out to examine the crystalline nature of the fibers, as shown in . The XRD traced two distinguished peaks at 16° and 22° which represent cellulose (200) and (202) crystallographic planes, respectively (Hajiha, Sain, and Mei Citation2014). Relativity, the broader peak at the lower angle reflection (16°), and sharper and stronger peak at the higher angle reflection (22°) are referred to the characteristic amorphous and crystalline parts of the fibers (Krishnaiah, Ratnam, and Manickam Citation2017). However, there is no marked variation in crystal nature between the difractograms of both fibers.
Furthermore, the Crystallinity Index (CI) of agave americana fibers is slightly smaller than that of agave sisalana fibers. The calculated average crystallinity index values (computed using EquationEquation 7(7)
(7) ) of both types of fibers range from 0.663 to 0.875 which infers they possess semicrystalline nature. This further proved agreement of the result with literature reports (Bai et al. Citation2002; Hajiha, Sain, and Mei Citation2014). Additionally, it is noted that the absence of a doublet peak around 22°C confirms existence of hemicellulose abundantly while cellulose exists in the type I cellulose (Mora´n et al. Citation2008).
SEM analysis
There were clear variations on surface morphologies between the microstructures of both types of fibers. It can be observed that surface roughening, longitudinal cracks and linings appeared on the surface structures () (Bai et al. Citation2002). Such surface phenomenon is resulted probably due to the method of extraction employed which suggested further optimizations and modifications are required. The damage is more severe on agave americana fibers. This surface appearance can be considered as an opportunity to enhance surface adhesion between constituent species during composite preparation (Mansouri et al. Citation2020). However, imperfections resulted from cracks and linings may facilitate phase separation (Li, Mai, and Ye Citation2000) which can reduce the intended performance and subsequently shortens the life span of a material.
EDAX analysis
EDAX measurements revealed that carbon (C) and oxygen (O) are dominant chemical species (). The mean O/C ratio of agave americana and agave sisalana fibers was 0.63 and 0.60, respectively; which is a little bit deviated from 0.83, the O/C ratio of pure natural fibers (Rajkumar et al. Citation2015). This further proved the surface and interface of component fibers possess significant amount of lignin, wax and other constituents. Furthermore, trace amounts of calcium (Ca), potassium (K), silicon (Si) and sulfur (S) were also appeared.
Linear density, breaking tensile strength and elongation at break
Results for linear density of fibers considered under this study fall in the range of 24.28 to 30.39 Tex (). However, the mean linear density of agave americana fibers recorded the highest (29.68 Tex) compared to the agave sisalana fibers (27.14 Tex). Agave americana fibers are both longer and thicker () which was proved by the values of length and diameter. The variability in linear density is due to the inconsistency of fiber diameter which is further associated with variation in climate, cultivation and extraction conditions of the leaf (Samouh et al. Citation2021). The linear density values for agave americana fibers are significantly higher than reported values from Tunisia (24 Tex) (Msahli, Sakli, and Drean Citation2006) and South Africa (26.9 Tex) (Boguslavsky et al. Citation2007). It is obvious that due to higher linear density (Tex), the fiber is coarser and thicker which meant strong enough to resist bending (Suvitha Citation2021).
Table 3. Comparison of mechanical properties of agave americana and agave sisalana fibers (Mean ±SD, n = 50).
Meanwhile, tensile strength and elongation are other forms of fiber property determinants which govern performance of engineered materials. For both types of fibers, the evaluation revealed a significant variation in their mechanical properties. Agave sisalana fibers show greater average tensile strength values (~510 MPa) compared to agave americana fibers (~487 MPa) may be due to the presence of more cellulose content (~66%). Mechanical strength of natural fibers is highly susceptible to variations in chemical compositions (lignin, hemicellulose and cellulose), microstructures emanated from method of extractions and other modifications (Karimah et al. Citation2021). In this context, the value of breaking tensile strength and elongation at break of agave fibers is measured and presented in .
Furthermore, different scholars declared the pivotal role of cellulose content to govern crystalline nature and mechanical features of a fiber (Chokshi et al. Citation2022). This was further proved by research findings that the amount of cellulose has a direct effect on the tensile strength of natural fibers (Komuraiah, Kumar, and Prasad Citation2014). Moreover, greater amount of cellulose enhanced crystallinity index by reducing moisture and voids which in turn complements mechanical strength of natural fibers (Ismojo et al. Citation2020). Consequently, sisal fibers are preferably advantageous over their counter parts for the intended applications. However, they relatively possess smooth surfaces which might create poor interlocking during composite manufacturing (Latif et al. Citation2019).
Elongation at break is the amount of stretch to withstand an applied load before it breaks (Widyasanti, Napitupulu, and Thoriq Citation2020). From the results, it is evident that agave sisalana fibers showed the highest elongation at break (~6%) compared to agave americana fibers (~4%). Such increment in elongation resistance may be caused by the effectiveness of the extraction method to remove the amount of hemicellulose on the fibers. This can further enhance crystallinity of the fiber, leading to better extensibility. Greater stretching or elongation before breaking shows greater toughness and durability than a stiffer fiber that breaks at the same load but at low elongation (Samouh et al. Citation2021).
Comparison of mechanical properties of agave fibers with the existing literature
Comparison of mechanical properties of agave fibers (considered for this study) with literature reported values is quite difficult due to aforementioned variations. However, agave americana and agave sisalana fibers, which are roughly evaluated based on the values of tensile strength, from the current study have shown outstanding performances as compared to the open literature. This could further proved sisal fibers possessing variable tensile strength which emerge from the same region (Ethiopia) acquired by different extraction techniques. Average performances of mechanical properties are shown in .
Table 4. Summary of comparison of mechanical properties of agave americana and agave sisalana fibers.
Conclusion
Based on the findings of this study, the following conclusions were made:
The values of physico-chemical properties of agave americana and agave sisalana fibers show variability which depends on the origin, climate conditions, soil properties and maturity of the leaf.
Experimental values proved that more than 90% of the fibers are composed of lignin, hemicellulose and cellulose with marked variations in chemical composition of each constituent.
Extraction of sisal leaves using soil retting facilitates comparatively smooth, fine and mechanically strong fibers which is an evidence for the efficiency of the method that supports good mechanical properties for strong composite products.
To sum up, the findings of the physico-chemical and mechanical analyses showed that sisal (agave sisalana) fibers had better characteristics for the intended composite applications that require fine and strong features.
Due to the hygroscopic nature of natural fibers, agave fibers possess relatively low mechanical properties compared to synthetic fibers while using in engineering applications such as composite reinforcements. Hence, to overcome these drawbacks, further surface modifications are required.
Highlights
Extraction of longer and stronger agave fibers.
Comparison of physico-chemical and mechanical properties of agave sisalana and agave americana fibers
Agave sisalana fibers possess superior tensile strength compared to agave americana fibers.
Author’s contribution
All authors contribute their share starting from sample collection, characterization, analysis and write-up.
Acknowledgements
The first author gratefully acknowledges the financial support from International Foundation of Science (IFS) {scheme number: I-2-W-6248-1} and Mekelle University, Department of Chemistry for the access of laboratory facilities.
Disclosure statement
No potential conflict of interest was reported by the author(s).
Data availability statement
No additional supplemental material is available for this paper.
Additional information
Funding
References
- Amel, B. A., M. T. Paridah, R. Sudin, U. M. K. Anwar, and A. S. Hussein. 2013. Effect of fiber extraction methods on some properties of kenaf bast fiber. Industrial Crops and Products 46:117–15. doi:10.1016/j.indcrop.2012.12.015.
- Amior, A., H. Satha, F. Laoutid, A. Toncheva, and P. Dubois. 2023. Natural cellulose from Ziziphus jujuba fibers: Extraction and characterization. Materials 16 (1):385. doi:10.3390/ma16010385.
- Asfaw, K. G. 2011. Investigation of the reasons for the unique growth and development of agave species (agave sisalana and agave americana) crop plants at the Southern, Central, North Western and Eastern parts of Tigray, Ethiopia. Current Research Journal of Biological Sciences 3:273–81.
- Bai, S.-L., R. K. Y. Li, Y.-W. Mai, and C. M. L. Wu. 2002. Morphological study of sisal fibres. Advanced Composites Letters 11 (3):123–29. doi:10.1177/096369350201100304.
- Boguslavsky, A., F. Barkhuysen, E. Timme, and R. N. Matsane. 2007. Establishing of agave americana industry in South Africa. University of Cape Town. South Africa. 5th International Conference on New Crops. Southampton.
- Chokshi, S., V. Parmar, P. Gohil, and V. Chaudhary. 2022. Chemical composition and mechanical properties of natural fibers. Journal of Natural Fibers 19 (10):3942–53. doi:10.1080/15440478.2020.1848738.
- Debnath, M., M. Pandey, R. Sharma, G. S. Thakur, and P. Lal. 2010. Biotechnological intervention of Agave sisalana: A unique fiber yielding plant with medicinal property. Journal of Medicinal Plants Research 4:177–87.
- Ebisike, K., B. E. AttahDaniel, B. Babatope, and S. O. O. Olusunle. 2013. Studies on the extraction of naturally-occurring banana fibers. The International Journal of Engineering and Science 2:95–99.
- El Oudiani, A., R. Ben Sghaier, Y. Chaabouni, S. Msahli, and F. Sakli. 2012. Physico-chemical and mechanical characterization of alkali-treated Agave americana L. fiber. The Journal of the Textile Institute 103 (4):349–55. doi:10.1080/00405000.2011.578358.
- Fávaro, S. L., T. A. Ganzerli, A. G. V. de Carvalho Neto, O. R. R. F. da Silva, and E. Radovanovic. 2010. Chemical, morphological and mechanical analysis of sisal fiber-reinforced recycled high-density polyethylene composites. Express Polymer Letters 4 (8):465–73. doi:10.3144/expresspolymlett.2010.59.
- Ganan, P., S. Garbizu, R. Llano-Ponte, and I. Mondragon. 2005. Surface modification of sisal fibers: Effects on the mechanical and thermal properties of their epoxy composites. Polymer Composites 26 (2):121–27. doi:10.1002/pc.20083.
- Gurram, A. M., and D. N. Rao. 2016. Synthesis and characterization of sisal fibers. International Journal of Engineering and Technical Research 6:81–83.
- Hajiha, H., M. Sain, and L. H. Mei. 2014. Modification and characterization of hemp and sisal fibers. Journal of Natural Fibers 11 (2):144–68. doi:10.1080/15440478.2013.861779.
- Haque, R., M. Saxena, S. C. Shit, and P. Asokan. 2015. Fibre-matrix adhesion and properties evaluation of sisal polymer composite. Fibers and Polymers 16 (1):146–52. doi:10.1007/s12221-015-0146-2.
- Hulle, A., P. Kadole, and P. Katkar. 2015. Agave americana leaf fibers-review. Fibers 3 (4):64–75. doi:10.3390/fib3010064.
- Ismojo, R. Hadiwibowo, E. Yuanita, E. Kustiyah, and M. Chalid. 2020. Study of crystallinity index and thermal properties of sweet sorghum fiber after pressurized-cooker treatment. Macromolecular Symposia 391 (1):1900129. doi:10.1002/masy201900129.
- Jasti, A., and S. Biswas. 2023. Characterization of elementary industrial hemp (Cannabis Sativa L.) fiber and its fabric. Journal of Natural Fibers 20 (1):2158982. doi:10.1080/15440478.2022.2158982.
- Karimah, A., M. R. Ridho, S. S. Munawar, D. S. I. Adi, B. Damayanti, R. Subiyanto, A. Fatriasari, W. Fudholi, and A. Fudholi. 2021. A review on natural fibers for development of eco-friendly bio-composite: Characteristics, and utilizations. Journal of Materials Research and Technology 13:2442–58. doi:10.1016/j.jmrt.2021.06.014.
- Kestur, S. G., T. H. S. Flores-Sahagun, L. P. Dos Santos, J. Dos Santos, I. Mazzaro, and A. Mikowski. 2013. Characterization of blue agave bagasse fibers of Mexico. Composites Part A 45:153–61. doi:10.1016/j.compositesa.2012.09.001.
- Kim, H., K. Okubo, T. Fujii, and K. Takemura. 2013. Influence of fiber extraction and surface modification on mechanical properties of green composites with bamboo fiber. Journal of Adhesion Science and Technology 27 (12):1348–58. doi:10.1080/01694243.2012.697363.
- Komuraiah, A., N. S. Kumar, and B. D. Prasad. 2014. Chemical composition of natural fibers and its influence on their mechanical properties. Mechanics of Composite Materials 50 (3):509–28. doi:10.1007/s11029-014-9422-2.
- Krishnadev, P., K. S. Subramaian, G. J. Janavi, S. Ganapathy, and A. Lakshmanan. 2020. Synthesis and characterization of nano-fibrillated cellulose derived from green agave americana L. fiber. BioResources 15 (2):2442–58. doi:10.15376/biores.15.2.2442-2458.
- Krishnaiah, P., C. T. Ratnam, and S. Manickam. 2017. Enhancements in crystallinity, thermal stability, tensile modulus and strength of sisal fibres and their PP composites induced by the synergistic effects of alkali and high intensity ultrasound (HIU) treatments. Ultrasonics Sonochemistry 34:729–42. doi:10.1016/j.ultsonch.2016.07.008.
- Latif, R., S. Wakeel, N. Z. Khan, A. N. Siddiquee, S. L. Verma, and Z. A. Khan. 2019. Surface treatments of plant fibers and their effects on mechanical properties of fiber-reinforced composites: a review. Journal of Reinforced Plastics and Composites 38 (1):15–30. doi:10.1177/0731684418802022.
- Li, Y., Y.-W. Mai, and L. Ye. 2000. Sisal fibre and its composites: a review of recent developments. Composites Science and Technology 60 (11):2037–55. doi:10.1016/S0266-3538(00)00101-9.
- Madera-Santana, T. J., H. S. Valdez, and M. O. W. Richardson. 2013. Influence of surface treatments on the physicochemical properties of short sisal fibers: Ethylene vinyl acetate composites. Polymer Engineering and Science 53 (1):59–68. doi:10.1002/pen.23232.
- Mansouri, A., J. BenNasr, M. BenAmar, and F. Elhalouani. 2020. Characterization of fiber extracted from Agave Americana after burial in soil. Fibers and Polymers 21 (4):724–32. doi:10.1007/s12221-020-8666-9.
- Martin, A. R., M. A. Martins, O. R. R. F. da Silva, and L. H. C. Mattoso. 2010. Studies on the thermal properties of sisal fiber and its compounds. Thermochimica Acta 506 (1–2):14–19. doi:10.1016/j.tca.2010.04.008.
- Mbugua, G. W. 2014. Properties of decorticated agave americana “marginata” fibres of different leaf levels from Lanet and Tigoni, Kenya. PhD diss., Kenyatta University.
- Mbugua, G. D., C.-T. Tumuti, and E. Nguku. 2021. Characterization of decorticated agave americana ‘marginata’ fibres of different leaf levels from Lanet and Tigoni, Kenya. International Journal of Innovative Research and Knowledge 6:86–96.
- Mora´n, J. I., V. A. Alvarez, V. P. Cyras, and A. Va´zquez. 2008. Extraction of cellulose and preparation of nanocellulose from sisal fibers. Cellulose 15 (1):149–59. doi:10.1007/s10570-007-9145-9.
- Moshi, A. A. M., D. Ravindran, B. S. R. Sundara, V. Suganthan, and S. S. G. Kennady. 2019. Characterization of new natural cellulosic fibers – a comprehensive review. IOP Conference Series: Materials Science & Engineering 574 (1):012013. doi:10.1088/1757-899X/574/1/012013.
- Msahli, S., Y. Chaabouni, F. Sakli, and J. Y. Drean. 2007. MechanicaL behavior of Agave Americana L. Fibres: correlation between fine structure and mechanical properties. Journal of Applied Science 7 (24):3951–57. doi:10.3923/jas.2007.3951.3957.
- Msahli, S., M. Jaouadi, F. Sakli, and J. Y. Drean. 2015. Study of the mechanical properties of fibers extracted from Tunisian agave americana L. Journal of Natural Fibers 12 (6):552–60. doi:10.1080/15440478.2014.984046.
- Msahli, S., F. Sakli, and J. Y. Drean. 2006. Fibres extracted from Tunusia agave americana L fibre. Textile Journal 6:9–13.
- Mylsamy, K., and I. Rajendran. 2010. Investigation on physio-chemical and mechanical properties of raw and alkali-treated agave americana fiber. Journal of Reinforced Plastics and Composites 29 (19):2925–35. doi:10.1177/0731684410362817.
- Oladele, I. O., and O. G. Agbabiaka. 2015. Investigating the influence of mercerization treatment of sisal fibre on the mechanical properties of reinforced polypropylene composites and modeling of the properties. Fibers and Polymers 16 (3):650–56. doi:10.1007/s12221-015-0650-4.
- Oladele, I. O., J. A. Omotoyinbo, and J. O. T. Adewara. 2010. Investigating the effect of chemical treatment on the constituents and tensile properties of sisal fibre. Journal of Minerals and Materials Characterization and Engineering 9 (6):569–82. doi:10.4236/jmmce.2010.96041.
- Ortega, Z., J. Castellano, L. Suárez, R. Paz, N. Díaz, A. N. Benítez, and M. D. Marrero. 2019. Characterization of agave americana L. plant as potential source of fibres for composites obtaining. SN Applied Sciences 1 (9):987. doi:10.1007/s42452-019-1022-2.
- Pappua, A., M. Saxena, V. K. Thakur, A. Sharma, and R. Haque. 2016. Facile extraction, processing and characterization of biorenewable sisal fibers for multifunctional applications. Journal of Macromolecular Science, Part A: Pure and Applied Chemistry 53 (7):424–32. doi:10.1080/10601325.2016.1176443.
- Periyasamy, A. P. 2021. Recent developments in high performance fibers. Last modified February 11, 2021. Accessed May 25, 2023. ht tp://ht tp:/textilelearner.net/Recent/developments/in/high/performance/fibers/
- Phologolo, T., C. Yu, J. I. Mwasiagi, N. Muya, and Z. F. Li. 2012. Production and characterization of Kenyan sisal. Asian Journal of Textile 2 (2):17–25. doi:10.3923/ajt.2012.17.25.
- Placet, V., F. Trivaudey, O. Cisse, V. Gucheret-Retel, and M. L. Boubakar. 2012. Diameter dependence of the apparent tensile modulus of hemp fibers: A morphological, structural or ultrastructural effect. Composites Part A 43 (2):275–87. doi:10.1016/j.compositesa.2011.10.019.
- Rajkumar, S., J. Tjong, S. K. Nayak, and M. Sain. 2015. Wetting behavior of soy-based resin and unsaturated polyester on surface-modified sisal fiber mat. Journal of Reinforced Plastics and Composites 34 (10):807–18. doi:10.1177/0731684415580630.
- Razali, N., M. S. Salit, M. Jawaid, M. R. Ishak, and Y. Lazim. 2015. A study on chemical composition, physical, tensile, morphological and thermal properties of Roselle fibre: Effect of fibre maturity. BioResources 10 (1):1803–24. doi:10.15376/biores.10.1.1803-1824.
- Samouh, Z., O. Cherkaoui, D. Soulat, A. R. Labanieh, F. Boussu, and R. E. Moznine. 2021. Identification of the physical and mechanical properties of Moroccan sisal yarns used as reinforcements for composite materials. Fibers 9 (2):13. doi:10.3390/fib9020013.
- Segal, L., J. Creely, A. Martin Jr., and C. Conrad. 1959. An empirical method for estimating the degree of crystallinity of native cellulose using the X-ray diffractometer. Textile Research Journal 29:786–94. doi:10.1177/004051755902901003.
- Srinivasakumar, P., M. J. Nandan, C. U. Kiran, and K. P. Rao. 2013. Sisal and its potential for creating innovative employment opportunities and economic prospects. IOSR Journal of Mechanical and Civil Engineering 8 (6):1–8. doi:10.9790/1684-0860108.
- Suvitha, L. 2021. Fabrication and evaluation of agave americana leaf fiber. Chennai, India: ICCAP. December 07-08. doi: 10.4108/eai.7-12-2021.2314639.
- Teklu, T. 2021. Characterization of physico-chemical, thermal, and mechanical properties of Ethiopian sisal fibers. Journal of Natural Fibers 19 (10):3825–36. doi:10.1080/15440478.2020.184873.
- Teklu, T., L. M. Wangatia, and E. Alemayehu. 2019. Effect of surface modification of sisal fibers on water absorption and mechanical properties of polyaniline composite. Polymer Composites 40 (S1):E46–52. doi:10.1002/pc.24462.
- Widyasanti, A., L. O. B. Napitupulu, and A. Thoriq. 2020. Physical and mechanical properties of natural fiber from sansevieria trifasciata and agave sisalana. IOP Conference Series: Earth and Environmental Science 462:012032. doi:10.1088/1755-1315/462/1/012032.