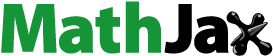
ABSTRACT
Yarn’s hairiness represents a continuous challenge for spinning technologies. To keep this aspect under control, an almost perfect combination between the construction and performance of the machines, the control of the technological processes through appropriate settings, and the experience of the producers are required. As a consequence, the researchers were preoccupied to adapt or modify the ring frame to produce yarns with a lower degree of hairiness. Spinning triangles as a very demanding area exert a crucial impact both on the distribution of fiber tension and their spatial location in the staple yarn structure. Our study encompasses yarn hairiness reduction employing a device composed of two bars. Various combinations of spindle speeds and contact angles between yarns and bars were tested to examine their effects on yarn hairiness. The study was completed with the optimization of technological parameters using a central, composite, rotating program with two independent variables (spindle speed and contact angle) to establish mathematical models and optimize technological parameters for the reduction of hairiness. The effectiveness and efficiency of this device consist of the easy execution and installation on the existing machines in spinning mills without any constructive adjustments and additional energy consumption.
摘要
纱线毛羽是纺纱技术面临的持续挑战. 为了控制这一方面,需要在机器的结构和性能、通过适当设置控制技术流程以及生产商的经验之间进行近乎完美的结合. 因此,研究人员专注于调整或修改环框,以生产毛羽程度较低的纱线. 纺纱三角形作为一个要求很高的区域,对纤维张力的分布及其在短纤维纱线结构中的空间位置都有着至关重要的影响. 我们的研究包括使用由两个杆组成的装置来减少纱线毛羽. 测试了锭子速度和纱线与棒材之间接触角的各种组合,以检验它们对纱线毛羽的影响. 这项研究是通过使用具有两个自变量(主轴速度和接触角)的中心复合旋转程序来优化工艺参数来完成的,以建立数学模型并优化减少毛羽的工艺参数. 该装置的有效性和效率包括在纺纱厂的现有机器上易于执行和安装,而无需任何结构调整和额外的能源消耗.
Introduction
Hairiness is a property that indicates the amount and length of fiber ends and loops protruding outside the yarn structure. It is a beneficial property in some situations and detrimental in others, depending on the end use and application of the textile material. The thermal insulation property of textiles is determined by the yarn hairiness which influences the amount of stationary air in the product. However, weaving preparation machines, knitting, and high-speed weaving machines require yarns with low yarn hairiness to reduce the coefficient of friction between the yarns and the working parts of the machines (Tyagi Citation2010).
Hairiness exerts a considerable effect not only on the handle, appearance of fabrics or knits, pilling (Almetwally et al. Citation2015), and comfort properties (Fangueiro et al. Citation2009; Kopitar et al. Citation2019), but also on the productivity of spinning and post-spinning processes (knitting, weaving preparation, and weaving).
Two types of hairiness are mentioned, the intrinsic hairiness is due to textile processing since acquired hairiness is a consequence of wool fiber curvature (Wan et al. Citation2014).
To diminish yarn hairiness, it is essential to understand the influence of the main parameters on it. Therefore, research has focused on studying the influence of fibers, yarns, spinning technologies, and spinning parameters on the hairiness of different yarn types (Wang, Chang, and McGregor Citation2006) Other subsequent studies have focused on testing, reducing, and predicting hairiness (Barella and Manich Citation1998).
Hairiness formation can be ascribed to several factors like fiber attributes (fiber length, fineness, short fiber content, uniformity, fiber curvature (Barella and Manich Citation1998), fiber mobility in yarn, process parameters (spindle speed, traveler selection, twist) (Niijjaawan and Niijjaawan Citation2010), relative humidity (***, “All about Yarn Hairiness,” November 5, 2021, https://www.testextextile.com/all-about-yarn-hairiness/) and spinning technology.
Commonly, the higher the yarn fineness, the lower the degree of hairiness. Nearly all hairiness is generated by short fibers (Tyagi Citation2010).
Fiber mobility is a decisive factor of yarn design as it determines the dimensional stability of both yarns and woven or knitted textile goods. Most spun yarns display a high degree of fiber mobility which, can affect the yarn performance during their processing into knitted or woven fabrics and their function in various end uses (Elmogahzy Citation2020).
Long fibers protruding from the yarn body can have a considerable negative effect on the physical-mechanical, comfort, or aesthetic characteristics of the yarn, woven or knitted structures (Elmogahzy Citation2020).
In their study, Wang, Chang, and McGregor (Citation2006) tent to establish the effect of fiber curvature on the hairiness of woolen worsted yarns. For this purpose, several carefully selected samples of wool and cashmere yarns, as well as cashmere-wool blend yarns with various participation ratios of the two components, were used to examine the effects of fiber curvature and blend ratio on yarn hairiness. Their results confirmed the significant effect of fiber curvature on yarn hairiness. Thus, yarns spun from fibers with higher curvature have lower yarn hairiness than yarns spun from comparable fibers with lower curvature (Wang, Chang, and McGregor Citation2006). Yarn properties are mainly affected by the yarn structure, which is mainly determined by the spinning technology (Tumajer et al. Citation2012). Accordingly, differences in hairiness between yarns spun on the ring spinning machine and yarns spun on the rotor spinning machine could be noticed. Although the hairiness is lower than that of conventional yarns, with differences of 20–40% being specified, the hairiness non-uniformity is much more pronounced in rotor-spun yarns (Dodu et al. Citation2002). This result is due to the different principles of the two spinning methods.
In ring spinning, the fiber migration phenomenon forces a large number of short fibers close to the yarn surface, which increases hairiness. In addition, the traveler promotes the appearance of hairiness by friction. In rotor spinning, the doubling effect in the rotor and the low fiber migration rate result in lower hairiness density (Elmogahzy Citation2009).
Each spinning process tends to produce a distinct yarn structure. In recent years innovations in spinning technologies have brought significant advances in yarn quality. Representatives in this respect are Jet ring (Ekrem, Usal, and Demet Citation2020), Solo (C. Ward, “Solospun™ – the long staple weavable singles yarn,” April 13, 2011, https://csiropedia.csiro.au/solospun/; Merati, Alamdar-Yazdi, and Farokhnia Citation2014), Siro spun processes (***, Saurer Sirospun – create two-ply yarn on one machine, 2022. C. Ward, 2011. https://saurer.com/en/news/saurersirospun#, https://www.cognetex.com/eng/scheda_com4wool.html), three-strand spinning technology as a modified version of the Siro spinning (Ling et al. Citation2022), Solo-Siro spinning system, Siro-Jet Spinning System, Sirofil Spinning System (Xia et al. Citation2015), Compact spinning technology and Compact-Siro technology (Su et al. Citation2015), ProSpin spinning approach (Yin et al. Citation2021) and Nu-Torque technology (Yin et al. Citation2021; Ying et al. Citation2015; Yin, Tao, and Xu Citation2020).
The higher hairiness values of yarns spun on ring-spinning machines compared to those spun with compact systems could be associated with the presence of a large spinning triangle, which causes a large difference in the path followed by the fibers in the outer layer of the winding and the central layer. Because of this, the fibers on the outside are not properly integrated into the yarn structure. In compact spinning, the spinning triangle is almost eliminated and nearly all fibers are incorporated into the yarn structure under the same tension. This leads to significant advantages, such as increased yarn tenacity, yarn abrasion resistance, and diminished yarn hairiness (Almetwally et al. Citation2015).
Compact spinning is one of the most important improvements of conventional ring spinning, through the implementation of a condensation device of the fibrous sliver, a device that leads to a consistent decrease of the spinning triangle, with a more uniform distribution of tensions when the fibers are embedded in the structure of the yarn, and reduced hairiness (Balasubramanian Citation2022; Su et al. Citation2015).
Various producers have developed compact spinning based on distinct variants of condensing systems. Presently, there are two fundamental principles, aerodynamically and mechanically compact spinning systems (Khan, Begum, and Sheikh Citation2020). Main compact spinning frame producers encompass Rieter (ComforSpin K-45, Compact spinning K-47, Compact-spinning K-48) (***. Compact Spinning. Highest flexibility, perfect yarn structure. Accessed November 22, 2022. https://www.rieter.com/products/systems/compact-spinning; Süessen (EliTe) (***. Suessen’s New EliTe is the most versatile, flexible, and in-demand compact spinning system in the market. Accessed November 22, 2022. https://www.suessen.com/productshttps://www.testextextile.com/all-about-yarn-hairiness/; ***. Suessen’s compact spinning system. Accessed November 10, 2022. https://indiantextilejournal.com/suessens-compact-spinning-system/) Cognetex (Com4 Wool), (***. Compact ring spinning frame. Accessed November 20, 2022.https://www.cognetex.com/eng/scheda_com4wool.html), Zinser (Air-Com-Tex, ZI 72 XL, ZI 451), (***. Zinser Systems – Compact spinning. Accessed November 17, 2022.https://saurer.com/en/systems/zinser-ring-spinning-system/zinser-systems-compact-spinning#) Toyota® RX-240, RoCoS and RoVaC of Rotorcraft (Khan, Begum, and Sheikh Citation2020). Decisive for the yarn manufacturer is that the compact spinning technology can be universally applied for short and long staple spinning division and for a full range of length densities, 100% fibers natural and synthetics and their fiber blends.
Based on the influence due to shape and size of the spinning triangle on yarn hairiness, the present study aimed at designing, testing, and implementing devices and accessories to be placed on classical ring spinning machines on the yarn path between the front rollers of the drafting system and the bar with the pigtail yarn guides. The role of these devices was to modify the spinning triangle and “force” the protruding, rebel fibers to embed themselves in the compact yarn structure, thereby reducing hairiness. Within this study, we focused on hairiness reduction by employing an innovative device consisting of two bars. The effectiveness and efficiency of this mechanism reside in the simplicity of execution and installation on the existing machines in spinning mills without any constructive adjustments and additional energy consumption. The study was completed with the optimization of technological parameters for the reduction of hairiness using this device with bars.
Materials and methods
Vertical and horizontal devices were conceived and their combination with various accessories: a fixed ball mounted above or below the device, one or two rollers, and one, two, or even three yarn guides. The devices were stationary or driven in rotational motion at lower or higher speeds, both in one direction and in the opposite direction, at various distances from the drafting system. These devices aimed to modify the spinning triangle and therefore to reduce the yarn hairiness, especially the hairiness responsible for accentuated pilling tendency.
All the tests were done under industrial conditions simultaneously with the usual yarn production at the Silvania Worsted Spinning (SWS) Company in Şimleul Silvaniei, Romania. The company is part of the Suedwolle Group Germany, a world leader in the production and commercialization of worsted wool and wool blends with various chemical fibers.
For comparison purposes, the yarns produced by the approached methods for hairiness reduction (with innovative developed devices) had fibrous compositions and length densities identical or similar to those of the yarns usually produced for customers of Silvania Worsted Spinning Company, along with the technological parameters. The technological parameters, yarn and fibers properties were as follows: 100% untreated wool fibers of 60–65 mm fiber with 19.3 μm diameter and yarn with length density Tt = 25 tex, 570 twists/m, Z direction twist. The spindle speed was set at 9000 rpm. The physical-mechanical properties of the manufactured yarns were evaluated with the Uster Zweigle HL 400 and Uster Tester 4 devices. Hairiness indexes were determined according to ASTM D5647–07 (2018).
The Uster Zweigle HL 400 device provides detailed hairiness data by length classes of fiber ends protruding from the yarn body. Individual count of fibers is done in seven length zones: the numbers of protruding fibers are measured in intervals of 1, 2, 3, 4, 6, 8, and 10 mm. This means that the measuring range is from 1 to 10 mm. The fiber ends protruding longer than 10 mm are included in the 10 mm category. The numbers of protruding fibers are given for each length zone (absolute, noncumulative) for 100 meters of yarn (***Uster Zweigle HL 400 (Citation2011). Uster Zweigle HL 400–Application handbook, hairiness length classification. Uster Zweigle).
The yarns hairiness can be evaluated with the following specific indexes: H – the total length in cm of all protruding fiber ends per unit length in cm; S1 – represents the number of all fibers of 1 mm; S3 – represents the number of all fibers of 3 mm and longer than 3 mm, including 10 mm; S10 – represents the number of all fibers of 10 mm and longer.
Experimental part
In broader research, to design the various tested devices, we grouped the research into four sections: spinning with a vertical device, a horizontal device, a combination of vertical and horizontal devices, and the use of a device with bars.
The preliminary research comprising the use of vertical and horizontal devices or their combinations with the various accessories demonstrated that their implementation in practice is neither simple nor economical. Thus, these devices would be required for each spinning unit which generates relatively high costs for their manufacture, assembly, and adjustment. Besides, to set the device in motion a motor is necessary for each unit.
Experimental part with the device with bars
To eliminate the inconveniences noticed by the use of horizontal and vertical devices, a more convenient device in terms of execution, implementation, and setting was designed. The device consists of two horizontal anodized bars to assure good friction resistance and an extremely low coefficient of friction with the yarns. The bars were mounted along the entire length of the machine between the front drafting rolls and the bar with pigtail guides.
shows the device with bars, the appearance of the bars, the adjustment area of the device and the contact angle. The contact angle is defined as the angle between the line described by the yarn without being in contact with the bars (as it is in the classical spinning situation) and the line described by the yarn when it is in contact with the bars of the presented device ().
Figure 1. a. The device with bars; b. The bars of the device; c. Adjustment of the bars device; d. The contact angle.

To test the efficiency of this device, 14 variants of the device with bars were accomplished. The position of the bars can be adjusted centrally from the end of the machine (see ), by moving them relative to each other, both vertically and horizontally, but also globally toward the front drafting rolls or the bar with pigtail guides. Subsequently, the contact angles of the yarns with the two bars or the distance between the device and the front rolls of the drafting system can be adjusted.
After several attempts, the appropriate position of the bars for a modified spinning triangle should be 45 mm from the contact line between the front rolls of the drafting system. Trials revealed that various spindle speeds and various contact angles between yarns and bars conduct at different values of the H, S1, S3, and S10 parameters indicating the hairiness of the produced yarns.
A series of combinations around average values of the contact angle (30°), and spindle speed (10000 rpm), was adopted. Therefore, a program, depicted in was conceived. For each experiment and combination, the results were compared with a reference (yarn manufactured under the same technological parameters, but without the device with bars).
Table 1. The experimental program for preliminary trials.
Optimisation of the S10 hairiness index
Regression equations with significant coefficients resulted only in the optimization of H and S10 indexes. The S10 indicator reveals severe hairiness which accentuates the piling tendency in fabrics or knits. In the frame of this study, the optimization of S10 is discussed. Classical analysis of the simultaneous action of independent variables on dependent variables requires a large number of experimental variants. A central, composite, rotating program with two independent variables was used to identify mathematical models and optimize technological parameters.
The mathematical modeling was accomplished in Mathcad 2000 and comprises 13 experiments including 5 parallel experiments performed in the center of the program, at the central values of the independent parameters. The experimental matrix is used to assess the influence of the two independent variables on the outcome of the dependent variable, the S10 hairiness index, provided by Uster Zweigle HL 400. The coded levels and corresponding actual values of the two variables chosen for the experiment (contact angle and spindle speed) along with their influence exerted upon the hairiness index S10 are depicted in .
Table 2. Experimental matrix of the optimization program.
Results and discussion
The experimental program for preliminary trials was applied for the following hairiness indexes: H, S1, S3, S10, and CV. The values for the analyzed indexes are presented in . The recorded data reveals a decrease in the hairiness of the yarn in almost tested combinations by using the device with bars compared with the variants without the device.
Table 3. H, S1, S3, S10, and CV values for the tested variants, and percentage decrease by using the device.
We observe that the S1 index registers a decrease for all 14 variants with various combinations of the spindle speed and the contact angle of the yarns with the bars of the device. The average value of the number of fiber ends decreases from 8406 to 7827, the most significant decrease is observed for V2 and V10 variants (approximately 15%) according to . Only two of the variants show that the S1 parameter does not change.
Also, the S3 index presents a reduction in 13 of the 14 variants. The most significant ones are observed in the V2, V7–V10 variants, with values among 10–20% (see ). The average value of the cumulative number of fiber ends of 3 mm, 4 mm, 6 mm, 8 mm, and 10 mm decreases from 3143 to 2900. Only the V5 variant registers an increase, of only 5%. For the length class of 10 mm, fiber ends increase from 28 to 38 ends (about 30%), under the following combination: 0°Contact angle and a 10,000 rpm spindle speed. It seems that the simple, almost imperceptible contact of the yarn with the bars at this level of spindle speed does not lead to a decrease in hairiness with 3 mm and longer ends.
The S10 index is presented in . In this case, the decreases are no longer nearly generalized as in the case of S1 and S3 indexes. The decrease in the average number of protruding ends of 10 mm fibers length and longer is about 34 to 31 ends/100 m of yarn. In 7 of the 14 variants, the S10 index decreased by 9–42%, while in the other 7, a small increase (0–10%) was noticed. The most significant decrease (42%) was achieved for the V7 variant under a 30°Contact angle and 8000 rpm spindle speed combination. In the case of the V5 variant, the combination of a 0°Contact angle and 10,000 rot/min spindle speed leads to a significative increase (36%), similar to the same variant for the S3 index. Therefore, this combination does not correspond to our purpose.
The percentage decrease values of H, S1, S3, S10, and CV indexes are presented in . We notice that in almost every variant there is a decrease of the S1, S3, and S10 parameters, by up to 15%, 18%, and 42%, respectively. For the CV values, we observe that the irregularity of the yarns made both in the classic spinning and the one with the device with bars presents close values, the values of the calculated percentage differences leading to the idea that the irregularity is not negatively affected, but it does not improve either.
For the fabricated yarns, the imperfections were also analyzed and the values are depicted in . According to the data, we observe a decrease in the number of imperfections with values between 12.6%, for thicks of +35%, and 35.5%, for neps of +200%.
Table 4. The yarn’s imperfections after classical spinning and spinning with the device with bars.
To test the efficiency of the proposed spinning procedure, we performed the winding of the yarns obtained by using the device with bars. The analysis of the yarn’s hairiness after winding revealed an increase in hairiness compared to that obtained after spinning with the bars device. Despite this, we still noticed a decrease in yarn hairiness compared to the classic spinning as there is depicted in .
Figure 2. The yarn’s hairiness after classical spinning, spinning with the device with bars and winding: a) the yarn’s hairiness values for S1 + 2, S3 and 1 mm; b) the yarn’s hairiness values for 2 mm, 3 mm and 4 mm; c) the yarn’s hairiness values for 6 mm, 8 mm and S10.

Starting from the fact that this device is very simple to implement and that in almost all the tested variants a decrease in the hairiness of the obtained yarns was noticed, further, we will address the optimization of the characteristics of the yarns according to the spindles speed and the contact angle of yarns with the bars. Based on the calculation program the following coefficients of the regression equation were obtained:
The Student’s test was applied to establish the significance of the coefficients, resulting that only part of the coefficients is significant. The significance of the coefficients: tc0 = 29.38189, so b0 significant; tc1=-5.53938 so b1 significant; tc2 = 6.19625 so b2 significant; tc11=-0.06252 so b11 insignificant; tc22=-2.77815 so b22 significant; tc12 = 0.3125 so b12 insignificant. So, the coefficients b11 and b12 are insignificant and the critical value for the test is tα,ν = t0,05;6 = 2.132. Thus, the corresponding terms for the insignificant coefficients can be neglected and the regression equation becomes:
The adequacy of the model was verified using the Fisher test and percentage deviations. The calculated value, Fc = 3.008 is higher than the tabulated critical value Fν1, ν2, α = F12;12;0,05 = 2.69 (Cojocaru, Clocotici, and Dobra Citation1986), which indicates the accuracy of the model. Calculus regarding the appropriateness of the applied mathematical model using the statistic method Fisher-Snedecor reveals the fact that the model represents the real shaped process, corresponding to a probability of 95%. Deviation percentage values (A) of up to 20–25% evaluated by the difference between the measured and model-calculated S10 Hairiness Index confirms also the assumption. Nine deviation percentage values depicted in are within the required limits of ± 10% (Cojocaru, Clocotici, and Dobra Citation1986) which proves the ability of the model to represent the influence analyzed with 95% probability (Cojocaru, Clocotici, and Dobra Citation1986). The degree of concordance of the mathematical model was verified using the statistic F’C = 2.89, which is lower compared to the tabulated critical value F’c =Fν1, ν2, α = F 5;5;0,01 = 6.59 (Cojocaru, Clocotici, and Dobra Citation1986) indicating good model fit. Further, the Fisher–Snedecor test was used to verify the deviation of the survey data from the mean value. The calculated value Fc = 11.7 is higher than the tabulated critical value Fc = F α,ν1,ν2 = F0,05;12,4 = 5.91 indicating that the deviations are due to the independent variables and not to the experimental errors.
Table 5. The dependent variable (y) values of the function of x2, for constant x1.
The approximation degree of the mathematical model expressed by the standard error indicates the scatter of the experimental values around the regression equation: 266%.
Further, the computed correlation are: rx1×2 = 0.135;rx1y = -0.554;rx2y = 0.62
The Student test was applied to verify the significance of the coefficients for the simple correlation (Cojocaru, Clocotici, and Dobra Citation1986): If tc > tα,ν, where tα,ν represents a tabulated value, it is assumed that there is a correlation between the two variables.
The calculated values are: tc x1y = -2.2093; tc x2y = 2.6217264; tc x1×2 = 0.45337149.
The calculated value is greater than the values provided by literature (Cojocaru, Clocotici, and Dobra Citation1986), the critical value tα, ν=t0,05; 11 = 2.201 for tx1y and tx2y indicating that there is a connection between the independent variables x1 and x2 and the dependent variable y.
The positive value of the correlation coefficient rx1×2 indicates a direct correlation between the independent and the dependent variable, which means an enhancement of x1 and x2 leads to an increase of the dependent variable (S10 hairiness index), and accordingly, the reduction of the independent variables generates a decrease in S10 hairiness index.
From the analysis of the correlation coefficient, rx1y = -0.554 and rx2y = 0.62 respectively, a different influence of the two independent variables can be noticed since the variable x1 influences the outcome in a proportion of 55.4% and the variable x2 influences the outcome in a proportion of 62%. Besides, the significant influence exerted by the independent variables on the dependent variable is illustrated by a lower critical value Fα, f1,f2 = F0,05; 2; 10 = 3.97 compared to the calculated value of 10.04. The coefficient of multiple determination is equal to 0.667 and illustrates that the influence of the two independent variables on the outcome represents 66.7%, and the remaining 33.3% is due to other factors. The resulting models can be viewed geometrically as hypersurfaces in the three-dimensional space of independent variables in . Canonical analysis transforms the regression equation into a more simple form and interprets the resulting expression using geometric concepts. The canonical form of the regression equation has been changed, the new axis center has the coordinates: x1 = 0, x2 = 0.96938. The value of the dependent variable in the center of the response surface is yc = 20.005718. Hence, the following equation results from the calculation of coefficients of the canonical form:
Figure 3. The regression equation representation: a) the response surface of the regression equation; b) the constant level curves of the S10 hairiness index.

The constant level curves obtained by sectioning the response surface presented in , with constant level planes have a parabolic shape. They are depicted in and allow the evaluation of the dependent variable y under the conditions imposed by the independent variables x1 and x2. Because the metric invariant of coordinate change is zero, it can be considered that there is no single center.
Figure 4. The representation of the dependent variable; a) y as a function of constant x1 and x2 within the limits [-1.414; 1.414]; b) y as a function of constant x2 and x1 within the limits [-1.414; 1.414].
![Figure 4. The representation of the dependent variable; a) y as a function of constant x1 and x2 within the limits [-1.414; 1.414]; b) y as a function of constant x2 and x1 within the limits [-1.414; 1.414].](/cms/asset/2afc2038-7d44-41d3-a7c0-3a125e8eafd3/wjnf_a_2248386_f0004_oc.jpg)
Further, the analysis of the numerical coefficients of the mathematical model is expressed in revealing that the influence of the two independent parameters, x1 - the contact angle of the yarn with the bars of the device, and x2 - the spindle speed, is not significantly different. This is because the coefficients of the two variables are not consistently different in value, with a relative difference of only 11.7% between them. But the influence of the two parameters on the dependent variable manifests itself differently as it can be concluded from the “-“ sign for coefficient b1 and the “+” sign for coefficient b2. Increasing the spindle speed leads to an increase in the value of the dependent variable S10, and increasing the contact angle between the yarn and the device bars leads to a decrease in the S10 hairiness index. Accordingly, for a decrease in the S10 hairiness index, a decrease in the spindle speed combined with the enhancement of the contact angle between the yarn and the bars of the device would be appropriate. Besides, the following conclusion can be drawn: the influence of variable x1 on y is 11.78%; the influence of variable x2 on y is 13.18%; the independent variable x2 exerts a more significant influence upon the S10 hairiness index compared to the variable x1; the absence of interaction, as measured by the coefficient of the x1x2 term, indicates that the concerted effect of the variation of the two parameters can influence the minimization process to an insignificant extent because the coefficient b12 has been eliminated, being insignificant; the ratio of the coefficients of the quadratic terms to the free term quantifies the change of the y response to the variation of the two parameters; the existence of the quadratic form only for the variable x2 leads to the conclusion that the influence of the spindle speed is more important; The analysis of the character of the variation of the S10 hairiness index requires plotting the following equations:
a. the dependent variable as a function of x1 constant and x2 values within [-1.414; 1.414]:
Figure a comprises the representation of EquationEquations 4(4)
(4) -Equation8
(8)
(8) .
b. The dependent variable as a function of x2 constant and x1 within values [-1.414; 1.414]:
depicts the values of the response function, the resultant y as a function of x2 by maintaining the variable x1 constant at the values –1.414; –1; 0; +1, and +1.414, and the values of the response function, the dependent variable y as a function of x1 at the values -1.414; -1; 0; +1, and +1.414, and the variable x2 constant. encompasses the representation of EquationEquations 9(9)
(9) -Equation13
(13)
(13) .
The analysis of the graphical representations in reveals significant changes in the hairiness index S10, under the following circumstancesː constant contact angle between the yarn and the bar of the device with variation in the spindle speed; a constant value of the spindle speed and the variation of the contact angle between the yarn and the bars of the device.
Minimum values of the S10 parameter, the hairiness index, are recorded for x2 = -1.414 corresponding to a spindle speed of 6500 rpm and x1 = 1.414 (i.e. contact angle 60°). But, to have a higher spindle speed, hence a higher theoretical production, x2 = –1 (spindle speed of 7270–7300 rpm) and a contact angle of 30–50° are recommended.
For the variant that led to the minimization of the outcome, the S10 Hairiness Index, the combination in coded values x1 = 1 and x2=-1, corresponding to the pair of natural values (x1 = 50; x2 = 7270 rpm), a combination located in quadrant IV of the experimental region, yarns were produced while keeping the other experimental conditions unchanged. In this case, an irregularity of the S10 Hairiness Index of 13.6 fiber ends/100 m yarn was acquired.
Given the value of 12.8 of the S10 Hairiness Index (see ) according to the mathematical model, the resulting deviation of 6.25% is consistent with the other deviations calculated for testing the adequacy of the model attached to the experimental program. The small deviation between the calculated and measured value, obtained after repeating the experiments, with the adjustments for the pair of values (x1 = 50°; x2 = 7270 rpm), leads to the conclusion that the optimization operation was successful.
For this purpose, a comparison of yarns produced with the device with bars and without the device, using a digital camera (YG381A) was done (see ). The differences between the two yarns in terms of yarn hairiness are obvious. The yarn appearance on the contrast board shows an improvement in yarn quality (see ). We also analyzed the pilling and found an increase of 0.5 points, from 3 to 3.5 points.
Conclusions
The yarn hairiness is a problem and a constant challenge for spinning technologies.
Our study envisages hairiness reduction via an innovative device consisting of two bars. Several pairs of combinations among the contact angle and spindle speed were tested. To evaluate the hairiness of the obtained yarns in comparison with yarns obtained without the device the H, S1, S3, and S10 indexes were employed. The use of the proposed device lead to lower values of all hairiness indexes. The benefits of the innovative device include effectiveness, efficiency, simplicity of execution, and installation on the current machines in spinning mills with no constructive adaptation and supplementary energy consumption. Through the optimization of technological parameters, the appropriate values for the independent variables (spindle speed and contact angle) for the reduction of hairiness using this bar device could be established. The results of the current study open up new opportunities for research in this field, characterized by various challenges due to the diversity of the features of natural fibers and their blends along with customer demands. With low investments, the quality of the yarns could be improved in terms of hairiness.
Key highlights of this research paper are as follows
Our study envisages yarn hairiness reduction via an innovative device consisting of two bars. Several pairs of combinations among the contact angle and spindle speed were tested on ring spinning machines. To evaluate the hairiness of the obtained yarns in comparison with yarns obtained without the device the H, S1, S3, and S10 indexes were employed. The use of the proposed device leads to lower values of all hairiness indexes.
The benefits of the innovative device include effectiveness, efficiency, simplicity of execution, and installation on the current machines in spinning mills with no constructive adaptation and supplementary energy consumption.
Through the optimization of technological parameters, the appropriate values for the independent variables (spindle speed and contact angle) for the reduction of hairiness using this bar device could be established.
The results of the current study open up new opportunities for research in this field, characterized by various challenges due to the diversity of the features of natural fibers and their blends along with customer demands. With low investments, the quality of the yarns could be improved in terms of hairiness.
Disclosure statement
No potential conflict of interest was reported by the authors.
References
- ***Suessen’s compact spinning system. Accessed November 10, 2022. https://indiantextilejournal.com/suessens-compact-spinning-system/.
- ***Zinser Systems - compact spinning. Accessed November 17, 2022. https://saurer.com/en/systems/zinser-ring-spinning-system/zinser-systems-compact-spinning#.
- ***Saurer Sirospun – create two-ply yarn on one machine. Accessed November 22, 2022. https://saurer.com/en/news/saurersirospun#https://www.cognetex.com/eng/scheda_com4wool.html.
- ***Compact ring spinning frame. Accessed November 20, 2022. https://www.cognetex.com/eng/scheda_com4wool.html.
- ***Suessen’s New EliTe is the most versatile, flexible, and in demand compact spinning system in the market. Accessed November 22, 2022. https://www.suessen.com/productshttps://www.testextextile.com/all-about-yarn-hairiness/.
- Almetwally, A. A., M. M. Mourad, A. A. Hebeish, and M. A. Ramadan. 2015. Comparison between physical properties of ring-spun yarn and compact yarns spun from different pneumatic compacting systems. Indian Journal of Fibre & Textile Research 40:43–16.
- Balasubramanian, N. 2022. Hairiness of yarns: Relative merits of various systems. Accessed November 16, 2022. https://www.fibre2fashion.com/industry-article/1629/hairiness-of-yarns-relative-merits-of-various-systems.
- Barella, A., and A. M. Manich. 1998. The effect of testing speed on the hairiness of ring-spun and sirospun yarns. The Journal of the Textile Institute 89 (3):605–07. doi:10.1080/00405009808658647.
- Cojocaru, N., V. Clocotici, and D. Dobra. 1986. Metode statistice aplicate în industria textilă [Statistical methods applied in the textile industry]. București: Ed. Tehnică.
- Dodu, A., C. Preda, R. Butnaru, E. Visileanu, D. Dan, L. Călin, V. Greavu, V. Grigoraș, V. Oceanu, and S. Gherman. 2002. Fibre textile; Prelucrarea primară a bumbacului, inului şi cânepii; Filatură; Ţesătorie [Textile fibers; Primary processing of cotton, linen and hemp; Spinning; Weaving], Vol. 1 of Manualul Inginerului textilist [Handbook of Textile Engineer]. București: Agir.
- Ekrem, G., M. R. Usal, and Y. Demet. 2020. Effects of nozzle structural parameters on yarn hairiness in jetring spinning system. The Journal of the Textile Institute 111 (11):1567–77.
- Elmogahzy, Y. E. 2009. Structure and types of yarn for textile product design. In Engineering textiles, ed. Y. E. El Mogahzy, 240–70. Elsevier. doi:10.1533/9781845695415.2.240.
- Elmogahzy, Y. E. 2020. Yarns engineering textiles. In Woodhead publishing series in textiles, engineering textiles, Y. E. Elmogahzy, ed., 2nd ed, 223–48.Woodhead Publishing. doi:10.1016/B978-0-08-102488-1.00009-5.
- Fangueiro, R., P. Gonçalves, F. Soutinho, and C. Freitas. 2009. Moisture management performance of functional yarns based on wool fibers. Indian Journal of Fibre & Textile Research 34:315–20.
- ***Compact Spinning. Highest flexibility, and perfect yarn structure. Accessed November 22, 2022. https://www.rieter.com/products/systems/compact-spinning.
- Khan, M., H. Begum, and M. Sheikh. 2020. An overview on the spinning triangle based modifications of ring frame to reduce the staple yarn hairiness. Journal of Textile Science and Technology 6 (1):19–39. doi:10.4236/jtst.2020.61003.
- Kopitar, D., Z. Pavlovic, Z. Skenderi, and Z. Vrljicak. 2019. Effect of yarn type on knitted fabric thermophysiological comfort. International Congress of Innovative Textiles ICONTEX 2:540–48.
- Ling, Y., C. Henson, A. West, and R. Yin. 2022. Systematic investigation and evaluation of modified ring yarns by feeding three-roving strands. Textile Research Journal 8 (3):2033–2041.
- Merati, A. A., A. Alamdar-Yazdi, and A. Farokhnia. 2014. Yarn properties developments with diagonally slotted roller on ring spinning machine. Indian Journal of Fibre & Textile Research 39:289–95.
- Niijjaawan, N., and R. Niijjaawan. 2010. Tips to fine tune the spinning machinery. In Modern approach to maintenance in spinning, ed. N. Niijjaawan and R. Niijjaawan, 408–34. India: Woodhead Publishing.
- Su, X., W. Gao, X. Liu, C. Xie, and B. Xu. 2015. Research on the compact-siro spun yarn structure. Fibres & Textiles in Eastern Europe 23 (111):54–57. doi:10.5604/12303666.1152447.
- Tumajer, P., P. Ursiny, M. Bilek, E. Mouckova, and M. Pokorna. 2012. Influence of structure of the yarn on mechanical characteristics of yarns exposed to dynamic stress. AUTEX Research Journal 12 (2):44–49. doi:10.2478/v10304-012-0009-5.
- Tyagi, G. K. 2010. Yarn structure and properties from different spinning techniques. In Woodhead publishing series in textiles, advances in yarn spinning technology, ed. C. A. Lawrence, 119–54. Sawston, Cambridge: Woodhead Publishing.
- ***Uster Zweigle HL 400. 2011. Uster Zweigle HL 400–Application handbook, hairiness length classification. Uster Zweigle.
- Wang, X., L. Chang, and B. McGregor. 2006. The hairiness of worsted wool and cashmere yarns and the impact of fiber curvature on hairiness. Textile Research Journal 76 (4):281–87. doi:10.1177/0040517506061964.
- Wan, A., G. Jiang, Y. Weidong, and H. Cong. 2014. Fuzzing mechanism and fiber fatigue of wool knit. Indian Journal of Fibre & Textile Research 39:238–43.
- Xia, Z. G., H. Liu, J. J. Huang, S. J. Gu, and W. L. Xu. 2015. A study on the influence of a surface-contacting spinning strand on yarn appearance during sirofil spinning. Textile Research Journal 85 (2):128–39. doi:10.1177/0040517514542863.
- Ying, G., F. Jie, Y. Rong, W. Xungai, M. Van der Sluijs, and M. T. Xiaoming. 2015. Investigation and evaluation on fine upland cotton blend yarns made by the modified ring spinning system. Textile Research Journal 85 (13):1355–66. doi:10.1177/0040517514563717.
- Yin, R., Y. L. Ling, R. Fisher, Y. Chen, M. J. Li, W. L. Mu, and X. X. Huang. 2021. Viable approaches to increase the throughput of ring spinning: A critical review. Journal of Cleaner Production 323:129116. doi:10.1016/j.jclepro.2021.129116.
- Yin, R., X. M. Tao, and B. Xu. 2020. Yarn and fabric properties in a modified ring spinning system considering the effect of the friction surface of the false-twister. Textile Research 90 (5–6):572–80.
- Yin, R., Y. F. Xiang, Z. H. Zhang, X. M. Tao, J. M. Gluck, K. Chiu, and W. Lam. 2021. Cleaner production of mulberry spun silk yarns via a shortened and gassing-free production route. Journal of Cleaner Production 278:123690. doi:10.1016/j.jclepro.2020.123690.