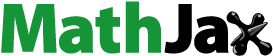
ABSTRACT
Giant goldenrod (S. gigantea Aiton) is considered an invasive weed in Europe, Asia, Australia, and New Zealand. In this research, the untreated and chitosan-treated cotton fabrics were functionalized with goldenrod inflorescences aqueous extract, at room temperature and 95°C. Zeta potential of fabrics was determined as a function of pH and the results showed an increased isoelectric point from 2.50 to 5.25 and amphoteric character of the chitosan-treated sample. The antioxidant activity (AA) of extract and functionalized fabrics was determined using DPPH analysis, while color and ultraviolet protection factor (UPF) were determined spectroscopically before and after wash and lightfastness testing. The chitosan pretreatment and dyeing of cotton at higher temperature increased AA (from 41.19 ± 0.28 to 56.08 ± 0.25), UPF (from 33.05 to 51.59) and color strength (K/S) (from 1.74 to 2.21), providing dyed cotton with excellent UV protection and a free radical inhibition activity. The samples had good wash fastness in terms of color (ΔEab* < 1), but not in terms of UV protection (UPF value decreased after washing to 23.47). The overall lightfastness was good to excellent (the UPF values of chitosan and dyed samples did not change much after lightfastness test (UPF = 51.75).
摘要
巨型一枝黄花(S.gigantea Aiton)在欧洲、亚洲、澳大利亚和新西兰被认为是一种入侵杂草. 在本研究中,在室温和95°C下,用一枝黄花花序水提取物对未处理和壳聚糖处理的棉织物进行功能化. 测定了织物的ζ电位作为pH的函数,结果表明,壳聚糖处理的样品的等电点从2.50增加到5.25,并且具有两性性质. 使用DPPH分析法测定提取物和功能化织物的抗氧化活性(AA),同时在洗涤和耐光牢度测试前后用光谱法测定颜色和紫外线保护因子(UPF). 壳聚糖预处理和高温染色提高了AA(从41.19 ± 0.28提高到56.08 ± 0.25)、UPF(从33.05提高到51.59)和色强度(K/S)(从1.74提高到2.21),为染色棉提供了良好的紫外线防护和自由基抑制活性. 样品在颜色方面具有良好的洗涤牢度(ΔEab*<1),但在紫外线防护方面没有(洗涤后UPF值降至23.47). 整体耐光性从好到优(壳聚糖和染色样品的UPF值在耐光性测试后变化不大(UPF = 51.75).
Introduction
Goldenrod (Solidago) is a perennial plant native to North America that was introduced to Europe as an ornamental plant in the 19th century (Burrows Citation2002; Stefanić et al. Citation2015). The native goldenrod species in Europe is European goldenrod (S. virgaurea L.), while the invasive species are Canadian goldenrod (S. canadensis L.) and giant goldenrod (S. gigantea Aiton) (Anžlovar, Janeš, and Dolenc Koce Citation2020). Traditionally, various goldenrod species have been widely used as remedies for the treatment of the urinary tract, kidneys, and prostate (Benelli et al. Citation2019; Demir et al. Citation2009). All of the above species are reported to have similar properties (Anžlovar, Janeš, and Dolenc Koce Citation2020), including anti-inflammatory, antimicrobial, antioxidant, antispasmodic, and diuretic effects due to the presence of different phytochemicals in the plant (Benelli et al. Citation2019; Demir et al. Citation2009; Fursenco et al. Citation2020; Topič, Gorjanc, and Kert Citation2018). These compounds are present in varying amounts depending on the type of goldenrod used to prepare the extract (Zekič, Vovk, and Glavnik Citation2021). The most abundant groups of secondary metabolites in goldenrod are flavonoids, terpenes, saponins, different phenolic acids, and essential oils (Fursenco et al. Citation2020). reported that the polyphenolic compounds are responsible for the antioxidant activity of the extracts, while flavonoids, in particular, confer spasmolytic and diuretic effects on the extracts. They also critically reviewed several studies on the antibacterial activity of goldenrod extracts, but the levels of bacterial inhibition were usually minimal or sometimes detectable only in methanol extracts. Nevertheless, antibacterial activity in these studies was attributed to many different phytochemicals (i.e., phenolic acids, flavonoids, terpenes, essential oils, etc.). A study on an extract of S. virgaurea L. suggested that the various active compounds present in the plant act synergistically (Fursenco et al. Citation2020). Since goldenrod contains dyes, it can be used for dyeing textiles. The aqueous extract of goldenrod contains several dyes, such as quercetin (C.I. 75670, Natural Yellow 10, 13), quercitrin (C.I. 75720, Natural Yellow 10), rutin (C.I. 75730, Natural Yellow 10), and kaempferol (C.I. 75640, C.I. Natural Yellow 13,10) (Leitner et al. Citation2012). The aqueous extract of giant goldenrod was found to have a higher content of quercitrin and quercetin than rutin (Čuk, Šala, and Gorjanc Citation2021; Zekič, Vovk, and Glavnik Citation2021). Inflorescences (Fröse et al. Citation2019; Topič, Gorjanc, and Kert Citation2018), stems (Thakker and Sun Citation2022), or even whole plants (Nguyen and Bechtold Citation2021) have been used to dye textiles, and yellow, gray, and pale brown shades have been obtained depending on the substrate, metal mordant, and dyeing method. Natural dyes, in contrast to synthetic dyes, are considered as green sources that allow colorful finishing of textiles (Y. Zhao and Liu Citation2021). Synthetic dyes are not biodegradable, and due to their overuse, they have entered aquatic and soil ecosystems, causing serious and significant damage to aquatic systems, long-term hazards (persistence, bioaccumulation of cancerogenic aromatic products, and generation of chlorination byproducts), and carcinogenic and teratogenic effects (Altintas Islam et al. Citation2022; Khattab, Abdelrahman, and Rehan Citation2020; Yildirim Citation2022). The greatest advantage of synthetic dyes is their better durability then that of their natural counterparts. To increase the sorption and durability of natural dyes on cellulosic fibers, textiles need to be mordanted with metal salts, which pose an ecological risk (Botteri, Miljković, and Glogar Citation2022; İ̇şmal and Yıldırım Citation2019; Repon, Islam, and Mamun Citation2017). Chitosan, a biodegradable polymer derived from crustacean shells, is an environmentally friendly alternative to metal mordants (Haji Citation2017; Ke, Zhu, and Chowdhury Citation2019) and can be used in the textile industry to improve dyeability or impart antimicrobial activity to textiles (Enescu Citation2008; Teli, Sheikh, and Shastrakar Citation2013). A literature search revealed that cotton pretreated with chitosan before dyeing with onion skin (Verma et al. Citation2021), spent coffee grounds (Mongkholrattanasit, Nakpathom, and Vuthiganond Citation2021), Ruellia tuberosa L (Kampeerapappun et al. Citation2010). Mangosteen rind (Kittinaovarat Citation2004) and pomegranate rinds (Haji Citation2017) had improved dyeability and washing fastness.
The aim of our research was to valorize giant goldenrod for dyeing cotton and to develop an environment friendly and sustainable textile with antioxidant properties and protection against UV radiation. Giant goldenrod, considered an invasive plant and usually disposed of as waste, was used as the plant material for functionalizing fabrics. The extract was prepared in an aqueous medium rather than organic and toxic solvents (Joshi and Adhikari Citation2019). In addition, chitosan was chosen for pretreatment as a bio-mordant instead of metal salts, to investigate the dyeability of cotton, its antioxidant properties, and protection against UV radiation, which has not been reported in the literature. The dyeing was performed at room temperature and at the boiling point for 60 minutes. We examined the fabrics for their color, transmittance to UV radiation, and antioxidant properties. Untreated and bio-mordanted fabrics, as well as goldenrod extract were analyzed for their surface charge (zeta potential analysis) to explain the interaction between extract and substrate. The samples were also subjected to UV irradiation and washing to evaluate the durability of the functionalization.
Materials and methods
Materials
Bleached cotton (weight: 119.2 g/m2, number of warp threads: 52 threads/cm, number of weft threads: 26 threads/cm; producer: Tekstina d.d., Ajdovščina, Slovenia) fabric was used as textile substrate, and the inflorescences of the giant goldenrod were used as a natural dye source.
Extraction of the goldenrod dye
The inflorescences of giant goldenrod (Solidago gigantea Aiton) were obtained from JP VOKA SNAGA d.o.o., Ljubljana, Slovenia, in August 2019, skimmed from the stems, placed in a polyethylene bag and frozen at − 20°C. The extract was prepared by pouring hot distilled water over the 50 g/l goldenrod inflorescences, left for 24 h, and then filtered. The pH of the extract was 5.2 and was used for dyeing cotton fabrics. This extract was analyzed for zeta potential and particle size using the Litesizer, as described elsewhere (Fras Zemljič et al. Citation2022).
Chitosan treatment and dyeing of cotton fabrics
Cotton fabrics (5 g) were bio-mordanted with 2 g/l of chitosan (ChemCruz, Santa Cruz Biotechnology, Dallas, Texas, USA) and 7.5 ml/l of CH3COOH 60% (Honeywell Fluka, Charlotte, North Carolina, USA) at a liquor-to-goods ratio of 20:1, at 80°C for 60 minutes. The fabrics were then wrung and used for dyeing as prepared. Untreated and chitosan treated textiles were dyed with goldenrod extract at a liquor-to-goods ratio of 40:1 at room temperature and 95°C for 60 minutes, using Gyrowash laboratory apparatus (James Heal, Halifax, West Yorkshire, UK). After dyeing, the textiles were washed with distilled water, wrung, and dried at room temperature.
Determination of the zeta potential of textiles as a function of pH
Using a cylindrical cell, zeta potential measurements were performed with SurPASS 3 (Anton Paar GmbH, Graz, Austria). Untreated and chitosan-treated fabrics were cut into approximately 5 × 5 cm2 pieces, randomly wrinkled, and fixed with the support discs. The permeability index of the sample was adjusted to approximately 100 by rotating the micrometer. A 1 mM KCl electrolyte solution was used, and the pH was automatically adjusted by 0.05 M NaOH and 0.05 M HCl. The pH dependence of the zeta potential was determined in the range of pH 3 − 9. A pressure gradient of 20,000–60000 Pa was applied to generate the streaming potential.
Wash and lightfastness testing
Washfastness was tested in a Gyrowash laboratory instrument (James Heal, Halifax, West Yorkshire, UK) according to the standard ISO 105-C06:2012, test method A1S. The samples were washed for 30 minutes at 40°C in 150 ml washing solution containing 4 g/l mild detergent (Perwoll Wool&Delicates, Henkel, Germany) and 10 stainless steel balls to simulate five domestic wash cycles. After washing, the samples were rinsed twice with distilled water and then air dried at room temperature. Color fastness to washing was visually assessed using gray scale (ranges 1 to 5) and spectrophotometrically by the determination of color difference between unwashed and washed samples, and by determination of UPF.
Lightfastness of the textiles was tested in a chamber equipped with a 300 W Osram® Ultra Witalux lamp emitting UVA/UVB and visible radiation, used for artificial material aging (manufacturer’s specifications (ULTRA VITALUX Citation2023). The light source has a radiation spectrum similar to that of normal sunlight and an energy output comparable to solar records at noon at midday in the northern hemisphere summer (Đokić et al. Citation2012; Heikkilä et al. Citation2017; Xu et al. Citation2016). Textile samples were placed 200 mm below the lamp and irradiated twice for 4 h at a temperature of 65°C to accelerate the aging of the material. Exposure conditions were performed according to ASTM Designation G154–06. Lightfastness was evaluated visually using blue scale (ranges 1 to 8) and spectrophotometrically by determination of color difference between samples before and after UV irradiation, and by determination of UPF.
Measurement of color and UV transmittance
Before and after fastness tests the textiles were analyzed for their color and UV protection factor (UPF).
The color (CIELAB coordinates) and reflectance (R %) to calculate the color strength (K/S) (H. Xin Citation2006) were measured using a Spectro 1050 reflectance spectrophotometer (Datacolor, Lucerne, Switzerland) with a 9 mm aperture. Five measurements were taken from each sample, and Datacolor software calculated the average value. The color difference (ΔEab*) between untreated and treated samples was calculated according to Equation 1:
where ΔL* is the difference in lightness, Δa* is the difference in red-green component, and ∆b* is the difference in blue-yellow component between two samples (untreated/treated).
The transmittance of UV radiation measurements and UV protection factor (UPF) calculations were performed according to the AATCC 183 test method (AATCC Citation2020) as in (Verbič et al. Citation2021). We performed three measurements (at 0°, 45°, and 90°) per two samples.
Antioxidant activity of extract and textiles
The antioxidant activity (AA) of goldenrod extract and cotton fabrics were analyzed using DPPH (1,1-diphenyl-2-picrylhydrazyl), a free radical scavenging method (Jadav and Gowda Citation2017; Singh and Sheikh Citation2020; Türkoğlu et al. Citation2017). The antioxidant activity was calculated according to Equation 2. Two samples per treatment were measured for AA, and the average value and standard deviation were calculated.
Here, ADPPH is the absorbance of the blank DPPH solution and ASOLUTION is the absorbance of the DPPH solution in contact with the textile or extract.
Results and discussion
Untreated and chitosan-treated cotton fabrics were dyed with an aqueous extract of giant goldenrod inflorescences. First, the surface charge of the textile substrates was analyzed by zeta potential measurements. The substrate’s surface charge defines the reactivity of the substrate and the sorption capacity of natural substances from the plant extract. shows pH-dependent zeta potential curves of untreated and chitosan-treated cotton fabrics. The zeta potential indicates the surface charge of the fabric before and after treatment or the charging behavior in an aqueous solution, i.e., surface acidic-base functional groups and their reactivity (Korica et al. Citation2021). The untreated cotton fabric exhibits a negative zeta potential over the entire pH range studied, indicating an abundance of negative functional groups in the cotton, such as such as carboxyl and hydroxyl groups (Flinčec Grgac et al. Citation2020). The isoelectric point (IEP) of untreated cotton is 2.50, which is in accordance with the literature. The chitosan-treated cotton has higher IEP value (5.25), which proves the basic character of chitosan successfully attached onto fabric surface. From the shape of ZP=f(pH) curve, it can be observed that fabric is amphoteric after chitosan bio-mordanting (Korica et al. Citation2019; Ristić, Hribernik, and Fras Zemljič Citation2015). The ability of the fabrics to be acidic (anionic character) is related to the presence of deprotonated carboxyl groups of cotton and attached chitosan residual N-acetyl groups from the parent chitin. The basic character (cationic charge) is due to the chitosan amino groups and their protonation to NH3+. The maximum positive zeta potential values are quite high (25 mV) and together with IEP, which is 5.25, one can conclude a high degree of fabric coverage by chitosan (theoretically, the IEP of chitosan is typically around 6.3–6.8) (Čakara et al. Citation2009). Comparing the plateau value of the anionic zeta potential, which is the same for both samples above pH 8, one can conclude that physical interactions are the driving force for the attachment of chitosan to cotton fabrics. This agrees with the study of (Čakara et al. Citation2009) who demonstrated the absence of electrostatic attraction between cotton and chitosan by a potentiometric titration study. However, it was proved that the irreversible adsorption of chitosan on the weakly acidic cotton fabric was predominantly due to non-electrostatic attraction, as also mentioned here. It was recognized that the additive effect of hydrogen and van der Waals interactions contributed to the irreversibility of chitosan binding onto cotton fabrics.
The CIELAB color coordinates of the samples are presented in . The undyed cotton fabric is white and therefore has high CIE L* (lightness) value, which decreases slightly after chitosan treatment. Dyeing cotton fabrics with aqueous goldenrod extract decreases the CIE L* values and changes the CIE a* and CIE b* values toward the green and yellow color axes. The textile samples become slightly greener (CIE a* is negative) when dyed at room temperature (cold dyeing) and slightly reddish (CIE a* is positive) when chitosan-treated before cold dyeing. There is a significant increase in the CIE b* value (yellower) for all dyed samples, especially for chitosan-treated samples or when dyeing was performed at 95°C (hot dyeing) due to a higher sorption of goldenrod dye in the fabrics. The ΔEab* was calculated between untreated and chitosan-treated samples (). Our results show that bio-mordanting of the fabric with chitosan influences the color change compared to untreated samples. In all cases, the ΔEab* values are > 1, meaning that a color change is visible, which can also be observed from the sample’s images.
Table 1. CIELAB color coordinates of untreated, chitosan-treated, and goldenrod extract dyed cotton fabrics, and color difference (ΔEab*) between untreated and chitosan-treated sample.
The K/S values indicate the color depth of the dyed fabric, or the amount of dye adsorbed into the fabric. The K/S maximum for all samples is in the yellow spectral region (at 400 nm wavelength) (). Chitosan bio-mordanting improves the color depth of dyed fabrics (, ). These samples are cationized due to the amino groups of chitosan, and they have a higher affinity for anionic natural compounds as goldenrod extract (Alebeid, Tao, and Seeahmed Citation2015; Gorjanc et al. Citation2019; Grancarić et al. Citation2013). Chitosan on textiles can also be electrostatically bound to goldenrod dye in water by ionic bonds or electrostatic attraction between the protonated positive amino group of chitosan and the negatively charged deprotonated hydroxyl group and carboxyl groups of the dye (Bretado Aragón et al. Citation2018; Del Valle, Díaz, and Puiggalí Citation2017). The latter depends on the pK values of these groups. Thus, if the cellulose substrate (e.g., cotton) is cationized, more sites are available for the binding of the anion groups of the natural dye (Topič, Gorjanc, and Kert Citation2018). The anionic character of goldenrod extracts was demonstrated by determining the zeta potential at its native pH (5.2). The zeta potential value of −17.2 mV clearly indicates that deprotonated acidic groups are present. Since the interactions between chitosan coating on fabric and goldenrod extract are pH-dependent, we can conclude that in addition to the low level of electrostatic interactions being hindered at higher pH values, the reason could be that the density of polar groups is higher when the chitosan is bound to the cotton fabric surface, making it more attractive to polar extracts, which may also improve the physical interactions. Under dyeing conditions, cellulose, chitosan and goldenrod dye mainly possess reactive functional groups, i.e. amino (–NH2) and hydroxyl (–OH), in addition to COOH/COO-, which enable their reactions mainly through inter- and intramolecular hydrogen bonding (Dodda et al. Citation2022) and van der Waals forces.
The dyeing temperature also greatly influences the higher sorption of goldenrod dye into cellulose substrates (, ). At higher temperatures, the dye molecules become more energetic, which increases the kinetic energy and diffusivity. In addition, the possible aggregation of the dye is reduced, which allows higher sorption of the dye into the cellulose substrate (Broadbent Citation2001).
The goldenrod dye adsorbed into the textiles affects the functionality, as evidenced by their antioxidant activity () and UV-protective properties (). The undyed samples do not exhibit antioxidant activity regardless of chitosan treatment (). The antioxidant activity of the aqueous goldenrod extract itself is 79.55 ± 0.25%. Cotton samples dyed with goldenrod showed antioxidant activity, while undyed samples showed no antioxidant activity, regardless of chitosan treatment. The lowest antioxidant activity of the dyed samples was observed for cold-dyed untreated cotton (only 27.33 ± 0.31%). Chitosan bio-mordanting improved the antioxidant activity to 36.72 ± 0.64%. Hot dyeing significantly improved the antioxidant activity of cotton, especially of chitosan-treated sample (AA = 56.08 ± 0.25%), which was attributed to the higher sorption of goldenrod dye. These results are in accordance with the previous ones. Higher sorption of the dye leads not only to higher color depth but also to better functional properties. The antioxidant activity of the goldenrod extract is not only due to the presence of dyes but also other compounds in the extract mainly poly/phenols. As previously mentioned, the antioxidant effect of extracts is due to the synergistic effects of the compounds, including chlorogenic acid, which is also present in the aqueous extract of giant goldenrod (Čuk, Šala, and Gorjanc Citation2021). Chlorogenic acid is a biologically active polyphenol with antioxidant, antibacterial, hepatoprotective, anti-inflammatory, antipyretic, and antiviral properties and is a free radical scavenger (Naveed et al. Citation2018). Antioxidant textiles act as a reservoir system that constantly supplies the skin with antioxidants and repairs damage or protects it from oxidative stress. Therefore, they play an important role in healing the skin microenvironment and preventing disorders or diseases (Alonso et al. Citation2013; Li et al. Citation2019).
Table 2. The antioxidant activity (AA) of untreated and chitosan-treated undyed and goldenrod extract dyed cotton fabrics.
Table 3. Ultraviolet protection factor (UPF), the transmittance of UVA and UVB radiation (T(UVA) and T(UVB), UVA and UVB blocking, and protection category of the samples.
Another important protection of the skin is that from UV radiation (Mavrić, Tomšič, and Simončič Citation2018). Daily and long-term exposure to UV radiation can cause skin damage, such as erythema, photoaging, DNA damage, melanoma, or other skin cancers (Tarbuk, Grancarić, and Situm Citation2016). Naturally dyed textiles can provide good protection against UV radiation, depending on the source of the natural dye and the concentration of the extract (Gorjanc et al. Citation2018). The UV protection of undyed cotton () is insufficient, regardless of chitosan treatment. Only after dyeing with goldenrod extract do the fabrics obtain their protection against UV radiation. The cold-dyed cotton samples have a UV protection factor (UPF) of about 28, which places them in the minimum protection category according to the Australian/New Zealand standard (AS/NZS Citation2017), which classifies the UPF values into three groups depending on the percentage of UV radiation that is blocked. The higher the UPF value, the better the UV-blocking ability of the sample. Therefore, a UPF value of 15–30 is considered minimal, 30–50 is considered good, and 50 and above is considered excellent. If a fabric is assigned an excellent UPF value, it means that the fabric blocks more than 98% of UVA and UVB radiation. The hot-dyed cotton sample provides good UV protection with a UPF value of 33.05. The UPF value increases when the chitosan-treated cotton is hot-dyed, falling into the excellent protection category with a UPF value of 51.59.
The dyed samples were subjected to wash- and lightfastness tests. The results of visual assessment of wash- and lightfastness () are very good to excellent. However, as the visual grading using only gray or blue scale does not provide precise evaluation of the colorfastness and protective properties after performed tests, after each test we measured color (CIELAB) and UV transmittance for UPF determination. The samples lose their UV protective ability after washing (five domestic washes), except for the sample treated with chitosan and dyed at 95°C (). The latter sample is classified in the minimum protection category with a UPF value of 23.47. Washing slightly changed the CIELAB values (), providing the color differences (ΔEab*) between unwashed and washed samples in range from 0.47 to 1.75, which means that color fastness to washing was quite good. The reason for good color fastness, but decreased UPF values after washing is the stability or instability of active compounds of goldenrod aqueous extract on cotton. In our previous research, we found high concentrations of dyestuff (e.g., quercetin, rutin) and chlorogenic acid (Čuk, Šala, and Gorjanc Citation2021) in goldenrod aqueous extract. As mentioned earlier, chlorogenic acid is a free radical scavenger, which is not stable in the presence of water (Holowinski, Dawidowicz, and Typek Citation2022; Ianni et al. Citation2022), but is not degraded under UVA or UVB irradiation (Rivelli et al. Citation2010). The poor washfastness in terms of UV protection (but not in terms of color) is due to the wash-off of chlorogenic acid. These results are consistent with the UPF results after the first and second lightfastness tests (). Our results show excellent UV stability of the samples dyed with goldenrod, especially those pretreated with chitosan, placing them in the good to excellent UV protection category. The UPF values of the untreated and dyed samples remain in the minimal UV protection category with UPF values around 25. The high UPF after lightfastness test is related to UV-stable chlorogenic acid, quercetin and rutin (Gęgotek, Domingues, and Skrzydlewska Citation2018; Rivelli et al. Citation2010; Scalia and Mezzena Citation2010; Vicentini et al. Citation2007; D.; Zhao et al. Citation2019). In addition to excellent UV protection, the samples become darker and more yellow after lightfastness test. Darkening of color after UV irradiation was also observed when dyeing polyester with the natural dye emodin (Räisänen, Nousiainen, and Hynninen Citation2001) and at higher concentration of Japanese knotweed extract when printing on paper and textiles (Klančnik Citation2021). The use of chitosan as a biomordant before dyeing with goldenrod aqueous extract enabled higher adsorption of goldenrod extract on cotton (, ), and also improved wash and lightfastness ().
Table 4. Gray and blue scale assessment of wash- and lightfastness.
Table 5. Ultraviolet protection factor (UPF) and protection category of dyed cotton fabrics after wash and lightfastness testing.
Table 6. CIELAB color coordinates of dyed cotton fabrics after wash and lightfastness testing.
Conclusions
The aqueous extract of the inflorescences of the invasive weed giant goldenrod was prepared for dyeing untreated and chitosan-treated cotton fabrics. The aim was to obtain textiles with good antioxidant and UV-protective properties. The fabrics were dyed at room temperature and at 95°C. Our results showed that higher dyeing temperatures and chitosan treatment increased the dyeability of cellulosic substrates with goldenrod dye. The samples exhibited better color strength, increased UV protection factor (from 3.76 to 51.59) and antioxidant activity (from 3.98% to 56.08%). The zeta potential analysis results showed that the reason for the higher sorption of goldenrod dye in chitosan-treated textiles was due to the increased isoelectric point, positive zeta potential values in a lower pH range, and less negative values in a higher pH range, which was due to the introduction of amino groups from the chitosan. Dyed samples showed good wash fastness in terms of color, but not in terms of UV protection. Chitosan improved wash fastness compared to untreated samples. The chitosan treated and hot dyed sample exhibited minimal protection after washing with a UPF of 23.47. The samples exhibited very good stability to UV irradiation. The research results are promising for the development of a sustainable and environmentally friendly production method of textiles with excellent UV protection (50+) and high antioxidant activity. Such textiles could be used as protective and healing garments for those who are exposed to high UV dosages daily and for long periods of time or/and have skin problems, as the antioxidant textiles support the healing of various skin infections and diseases. Further research on this topic is needed to increase the durability of textiles to wash in terms of UV protection.
Highlights
Cotton fabric with antioxidant and UV-protective properties was developed by functionalization with chitosan and giant goldenrod extract.
Bio-mordanting with chitosan changed the surface charge of cotton, making it amphoteric.
Combination of bio-mordanting and dyeing at high temperature (95°C) results in excellent UV protective properties (UPF 50+) and antioxidant activity of cotton.
The samples had good color fastness but poor UV protection fastness after washing and excellent UV protection after lightfastness test.
Author’s contributions
Conceptualization, M.G.; Formal analysis, S.Š., A.V., L.F.S.; Funding acquisition, M.G., L.F.S.; Investigation, S.Š.; Methodology, M.G., S.Š.; Supervision, M.G.; Writing – original draft, S.Š., M.G., L.F.S.; Writing – review and editing, S.Š., A.V., L.F.S. and M.G. All authors have read and agreed to the published version of the manuscript.
Acknowledgements
The authors would like to thank Tjaša Kraševac Glaser for valuable assistance with surface zeta potential measurements.
Disclosure statement
No potential conflict of interest was reported by the author(s).
Additional information
Funding
References
- AATCC. 2020. AATCC 183 : Test method for transmittance or blocking of erythemally weighted ultraviolet radiation through fabrics. NC, USA: American Association of Textile Chemists and Colorists (AATCC).
- Alebeid, O., Z. Tao, and A. Seeahmed. 2015. New approach for dyeing and UV protection properties of cotton fabric using natural dye extracted from henna leaves. Fibres & Textiles in Eastern Europe 23 (5(113)):60–13. doi:10.5604/12303666.1161758.
- Alonso, C., M. Martí, V. Martínez, L. Rubio, J. L. Parra, and L. Coderch. 2013. Antioxidant cosmeto-textiles: Skin assessment. European Journal of Pharmaceutics and Biopharmaceutics 84 (1):192–99. doi:10.1016/j.ejpb.2012.12.004.
- Anžlovar, S., D. Janeš, and J. Dolenc Koce. 2020. The effect of extracts and essential oil from invasive solidago spp. And Fallopia japonica on crop-borne fungi and wheat germination. Food Technology and Biotechnology 58 (3):273–83. doi:10.17113/ftb.58.03.20.6635.
- AS/NZS. 2017. AS/NZS 4399:2017 sun protective clothing–evaluation and classification. Wellington, New Zealand: Australian/New Zealand Standard.
- Benelli, G., R. Pavela, K. Cianfaglione, D. U. Nagy, A. Canale, and F. Maggi. 2019. Evaluation of two invasive plant invaders in Europe (Solidago canadensis and solidago gigantea) as possible sources of botanical insecticides. Journal of Pest Science 92 (2):805–21. doi:10.1007/s10340-018-1034-5.
- Botteri, L., A. Miljković, and M. I. Glogar. 2022. Influence of cotton pre-treatment on dyeing with onion and pomegranate peel extracts. Molecules 27 (14):4547. doi:10.3390/molecules27144547.
- Bretado Aragón, L., R. Jiménez Mejía, J. López-Meza, and P. Loeza-Lara. 2018. Composites of silver-chitosan nanoparticles: A potential source for new antimicrobial therapies. Revista Mexicana de Ciencias Farmaceuticas 47:7–25.
- Broadbent, A. D. 2001. Basic principles of textile coloration. Sherbrooke: Society of Dyers and Colourists.
- Burrows, R. L. 2002. Goldenrod: Plants with multipurpose potential. Hort Technology 12 (4):711–16. American Society for Horticultural Science. doi:10.21273/HORTTECH.12.4.711.
- Čakara, D., L. Fras Zemljič, M. Bračič, and K. Stana Kleinschek. 2009. Protonation Behavior of cotton fabric with irreversibly adsorbed chitosan: A potentiometric titration study. Carbohydrate Polymers 78 (1):36–40. doi:10.1016/j.carbpol.2009.04.011.
- Čuk, N., M. Šala, and M. Gorjanc. 2021. Development of antibacterial and UV protective cotton fabrics using plant food waste and alien invasive plant extracts as reducing agents for the in-situ synthesis of silver nanoparticles. Cellulose 28 (5):3215–33. doi:10.1007/s10570-021-03715-y.
- Del Valle, L. J., A. Díaz, and J. Puiggalí. 2017. Hydrogels for biomedical applications: Cellulose, chitosan, and protein/peptide derivatives. Gels 3 (3):27. doi:10.3390/gels3030027.
- Demir, H., L. Açik, E. B. Bali, L. Y. Koç, and G. Kaynak. 2009. Antioxidant and antimicrobial activities of solidago virgaurea extracts. African Journal of Biotechnology 8 (2):274–79.
- Dodda, J. M., M. G. Azar, P. Bělský, M. Šlouf, A. Brož, L. Bačáková, J. Kadlec, and T. Remiš. 2022. Biocompatible hydrogels based on chitosan, cellulose/Starch, PVA and PEDOT: PSS with high flexibility and high mechanical strength. Cellulose 29 (12):6697–717. doi:10.1007/s10570-022-04686-4.
- Đokić, V., J. Vujović, A. Marinković, R. Petrović, Đ. Janaćković, A. Onjia, and D. Mijin. 2012. A study of photocatalytic degradation of textile dye ci basic yellow 28 in water using P160 TiO2 based catalyst. Journal of the Serbian Chemical Society 77 (12):1747–57. doi:10.2298/JSC121015130D.
- Enescu, D. 2008. Use of chitosan in surface modification of textile materials. Romanian Biotechnological Letters 13 (6):4037–48.
- Flinčec Grgac, S., A. Tarbuk, T. Dekanić, W. Sujka, and Z. Draczyński. 2020. The chitosan implementation into cotton and polyester/Cotton blend Fabrics. Materials 13 (7):1616. doi:10.3390/ma13071616.
- Fröse, A., K. Schmidtke, T. Sukmann, I. Juhász Junger, and A. Ehrmann. 2019. Application of natural dyes on diverse textile materials. Optik 181:215–19. doi:10.1016/j.ijleo.2018.12.099.
- Fursenco, C., T. Calalb, L. Uncu, M. Dinu, and R. Ancuceanu. 2020. Solidago virgaurea L.: A review of its ethnomedicinal uses, phytochemistry, and pharmacological activities. Biomolecules 10 (12):1619. doi:10.3390/biom10121619.
- Gęgotek, A., P. Domingues, and E. Skrzydlewska. 2018. Proteins involved in the antioxidant and inflammatory response in rutin-treated human skin fibroblasts exposed to UVA or UVB irradiation. Journal of Dermatological Science 90 (3):241–52. doi:10.1016/j.jdermsci.2018.02.002.
- Gorjanc, M., M. Kert, A. Mujadžić, B. Simončič, P. Forte-Tavčar, B. Tomšič, and K. Kostanjšek. 2019. Cationic pretreatment of cotton and dyeing with Fallopia japonica leaves. Tekstilec 62 (3):181–86. doi:10.14502/Tekstilec2019.62.181-186.
- Gorjanc, M., M. Mozetič, A. Vesel, and R. Zaplotnik. 2018. Natural dyeing and UV protection of plasma treated cotton. The European Physical Journal D 72 (3):41. doi:10.1140/epjd/e2017-80680-9.
- Grancarić, A., N. Ristić, A. Tarbuk, and I. Ristić. 2013. Electrokinetic phenomena of cationized cotton and its dyeability with reactive dyes. Fibres & Textiles in Eastern Europe 21:106–10.
- Haji, A. 2017. Improved natural dyeing of cotton by plasma treatment and chitosan coating. Optimization by response surface methodology. Cellulose Chemistry and Technology 51 (9–10):975–82.
- Heikkilä, A., S. Kazadzis, O. Meinander, A. Vaskuri, P. Kärhä, V. Mylläri, S. Syrjälä, and T. Koskela. 2017. UV exposure in artificial and natural weathering: A comparative study. AIP Conference Proceedings 1810 (1):m:110004. doi:10.1063/1.4975566.
- Holowinski, P., A. L. Dawidowicz, and R. Typek. 2022. Chlorogenic acid-water complexes in chlorogenic acid containing food products. Journal of Food Composition and Analysis 109 (June):104509. doi:10.1016/j.jfca.2022.104509.
- Ianni, F., C. Barola, F. Blasi, S. Moretti, R. Galarini, and L. Cossignani. 2022. Investigation on chlorogenic acid stability in aqueous solution after microwave treatment. Food Chemistry 374 (April):131820. doi:10.1016/j.foodchem.2021.131820.
- Islam, S., A. Rahman, K. Nahar, S. R. Chowdhury, I. Ahmed, and K. M. Mohiuddin. 2022. Heavy metal pollution through hand loom-dyeing effluents and its effect on the community health. Environmental Science and Pollution Research International 29 (44):66490–506. doi:10.1007/s11356-022-20425-5.
- İ̇şmal, Ö. E., and L. Yıldırım. 2019. Metal mordants and biomordants. In The impact and prospects of green chemistry for textile technology, ed. Shahid-Ul-Islam and B. S. Butola, 57–82. The Textile Institute Book Series. doi:10.1016/B978-0-08-102491-1.00003-4.
- Jadav, K. M., and N. Gowda. 2017. Antibacterial and antioxidant properties of silk fabric dyed with Cichorium intybus root extract. International Journal of Pharmaceutics 4:299–304. doi:10.24327/ijrsr.2017.0807.0469.
- Joshi, D., and N. Adhikari. 2019. An overview on common organic solvents and their toxicity. Journal of Pharmaceutical Research International 1–18. doi:10.9734/jpri/2019/v28i330203.
- Kampeerapappun, P., T. Phattararittigul, S. Jittrong, and D. Kullachod. 2010. Effect of chitosan and mordants on dyeability of cotton fabrics with Ruellia Tuberosa Linn. Chiang Mai Journal of Science 38:95–104.
- Ke, G., K. Zhu, and M. Chowdhury. 2019. Dyeing of cochineal natural dye on cotton fabrics treated with oxidant and chitosan. Journal of Natural Fibers 18 (3):317–29. doi:10.1080/15440478.2019.1623742.
- Khattab, T. A., M. S. Abdelrahman, and M. Rehan. 2020. Textile dyeing industry: Environmental impacts and remediation. Environmental Science and Pollution Research 27 (4):3803–18. doi:10.1007/s11356-019-07137-z.
- Kittinaovarat, S. 2004. Using chitosan for improving the dyeability of cotton fabrics with mangosteen rind dye. Journal of Scientific Research, Chulalongkorn University 29 (2):155–64.
- Klančnik, M. 2021. Screen printing with natural dye extract from Japanese knotweed rhizome. Fibers and Polymers 22 (9):2498–506. doi:10.1007/s12221-021-0955-4.
- Korica, M., Z. Peršin, L. Fras Zemljič, K. Mihajlovski, B. Dojčinović, S. Trifunović, A. Vesel, T. Nikolić, and M. K. Mirjana. 2021. Chitosan nanoparticles functionalized viscose fabrics as potentially durable antibacterial medical textiles. Materials 14 (13):3762. doi:10.3390/ma14133762.
- Korica, M., Z. Peršin, S. Trifunović, K. Mihajlovski, T. Nikolić, S. Maletić, L. Fras Zemljič, and M. M. Kostić. 2019. Influence of different pretreatments on the antibacterial properties of chitosan functionalized viscose fabric: TEMPO oxidation and coating with tempo oxidized cellulose nanofibrils. Materials 12 (19):E3144. doi:10.3390/ma12193144.
- Leitner, P., C. Fitz-Binder, A. Mahmud-Ali, and T. Bechtold. 2012. Production of a concentrated natural dye from Canadian goldenrod (Solidago Canadensis) extracts. Dyes and Pigments 93 (1):1416–21. doi:10.1016/j.dyepig.2011.10.008.
- Li, Y., J. Guan, R. Tang, and Y. Qiao. 2019. Application of natural flavonoids to impart antioxidant and antibacterial activities to polyamide fiber for health care applications. Antioxidants. 8 (8):301. Multidisciplinary Digital Publishing Institute. doi:10.3390/antiox8080301.
- Mavrić, Z., B. Tomšič, and B. Simončič. 2018. Advances in the Ultraviolet Protection Finishing of Textiles. Textilec 61 (3):201–20. doi:10.14502/Tekstilec2018.61.201-220.
- Mongkholrattanasit, R., M. Nakpathom, and N. Vuthiganond. 2021. Eco-dyeing with biocolorant from spent coffee ground on low molecular weight chitosan crosslinked cotton. Sustainable Chemistry and Pharmacy 20:100389. doi:10.1016/j.scp.2021.100389.
- Naveed, M., V. Hejazi, M. Abbas, A. A. Kamboh, G. J. Khan, M. Shumzaid, F. Ahmad, D. Babazadeh, X. FangFang, F. Modarresi-Ghazani, et al. 2018. Chlorogenic acid (CGA): A pharmacological review and call for further research. Biomedicine & Pharmacotherapy 97:67–74. doi:10.1016/j.biopha.2017.10.064.
- Nguyen, H. L., and T. Bechtold. 2021. Thermal stability of natural dye lakes from Canadian goldenrod and onion peel as sustainable pigments. Journal of Cleaner Production 315:128195. doi:10.1016/j.jclepro.2021.128195.
- Räisänen, R., P. Nousiainen, and P. Hynninen. 2001. Emodin and dermocybin natural anthraquinones as high-temperature disperse dyes for polyester and polyamide. Textile Research Journal 71 (October):922–27. doi:10.1177/004051750107101012.
- Repon, M. R., M. T. Islam, and M. A. A. Mamun. 2017. Ecological risk assessment and health safety speculation during color fastness properties enhancement of natural dyed cotton through metallic mordants. The Impact and Prospects of Green Chemistry for Textile Technology, Fashion and Textiles 4 (1):1–17. doi:10.1186/s40691-017-0109-x.
- Ristić, T., S. Hribernik, and L. Fras Zemljič. 2015. Electrokinetic properties of fibres functionalised by chitosan and chitosan nanoparticles. Cellulose 22 (6):3811–23. doi:10.1007/s10570-015-0760-6.
- Rivelli, D. P., C. A. H. Filho, R. L. Almeida, C. D. Ropke, T. C. H. Sawada, and S. B. M. Barros. 2010. Chlorogenic acid UVA–UVB photostability. Photochemistry and Photobiology 86 (5):1005–07. doi:10.1111/j.1751-1097.2010.00776.x.
- Scalia, S., and M. Mezzena. 2010. Photostabilization effect of quercetin on the UV filter combination, butyl methoxydibenzoylmethane–Octyl methoxycinnamate. Photochemistry and Photobiology 86 (2):273–78. doi:10.1111/j.1751-1097.2009.00655.x.
- Singh, A., and J. Sheikh. 2020. Cleaner functional dyeing of wool using kigelia africana natural dye and Terminalia chebula bio-mordant. Sustainable Chemistry and Pharmacy 17:100286. doi:10.1016/j.scp.2020.100286.
- Stefanić, E., Z. Puškadija, I. Štefanić, and D. Bubalo. 2015. Goldenrod: A valuable plant for beekeeping in north-eastern croatia. Bee World 84 (2):88–92. doi:10.1080/0005772X.2003.11099581.
- Tarbuk, A., A. M. Grancarić, and M. Situm. 2016. Skin cancer and UV protection. AUTEX Research Journal 16 (1):19–28. doi:10.1515/aut-2015-0050.
- Teli, M. D., J. Sheikh, and P. Shastrakar. 2013. Exploratory investigation of chitosan as mordant for eco-friendly antibacterial printing of cotton with natural dyes. Journal of Textiles 2013 (3):1–6. doi:10.1155/2013/320510.
- Thakker, A. M., and D. Sun. 2022. Ecological application of natural biomaterial on natural fibres. Cleaner Materials 3:100038. doi:10.1016/j.clema.2021.100038.
- Topič, T., M. Gorjanc, and M. Kert. 2018. The influence of the treatment process on the dyeability of cotton fabric using goldenrod dye. Tekstilec 61 (3):192–200. doi:10.14502/Tekstilec2018.61.192-200.
- Türkoğlu, G. C., A. M. Sariişik, G. Erkan, H. Kayalar, O. Kontart, and S. Öztuna. 2017. Determination of antioxidant capacity of capsule loaded textiles. Indian Journal of Fibre & Textile Research 42:189–95.
- ULTRA VITALUX. 2023. ULTRA VITALUX 300 W 230 V E27 | OSRAM PIA. Accessed April 1. https://www.osram.com/ecat/ULTRA-VITALUX%20UV-A-UV-A-Ultraviolet%20lamps-Industry-Specialty%20Lighting/com/en/GPS01_1028569/ZMP_60829/.
- Verbič, A., M. Šala, I. Jerman, and M. Gorjanc. 2021. Novel green in situ synthesis of ZnO nanoparticles on cotton using pomegranate peel extract. Materials 14 (16):4472. doi:10.3390/ma14164472.
- Verma, M., N. Gahlot, S. S. J. Singh, and N. M. Rose. 2021. UV protection and antibacterial treatment of cellulosic fibre (Cotton) using chitosan and onion skin dye. Carbohydrate Polymers 257:117612. doi:10.1016/j.carbpol.2020.117612.
- Vicentini, F., S. Georgetti, J. Jabor, M. V. Juciene Caris, M. Bentley, M. Vincent, and M. Fonseca. 2007. Photostability of quercetin under exposure to UV irradiation. Latin American Journal of Pharmacy 26 (January):119–24.
- Xin, J. H. 2006. Total colour management in textiles. Cambridge: Woodhead Publishing.
- Xu, Y., J. Jin, X. Li, Y. Han, H. Meng, T. Wang, and X. Zhang. 2016. Simple synthesis of ZnO nanoflowers and its photocatalytic performances toward the photodegradation of metamitron. Materials Research Bulletin 76 (April):235–39. doi:10.1016/j.materresbull.2015.11.062.
- Yildirim, O. A. 2022. Detrimental effects of commonly used textile dyes on the aquatic environment and human health – a review. Fresenius Environmental Bulletin 9. 31 (October):9329–45.
- Zekič, J., I. Vovk, and V. Glavnik. 2021. Extraction and analyses of flavonoids and phenolic acids from Canadian goldenrod and giant goldenrod. Forests 12 (1):40. Multidisciplinary Digital Publishing Institute. doi:10.3390/f12010040.
- Zemljič, L. F., T. K. Glaser, O. Plohl, I. Anžel, V. Šimat, M. Čagalj, S. S. Možina, V. Malin, M. Sterniša, and S. S. Možina. 2022. Biomass-derived plant extracts in macromolecular chitosan matrices as a green coating for PLA films. Journal of Functional Biomaterials. 13 (4):4. Multidisciplinary Digital Publishing Institute:228. doi:10.3390/jfb13040228.
- Zhao, Y., and J. Liu. 2021. Extraction of tea natural dye and its dyeing properties on cotton fabrics. AATCC Journal of Research. 8 (2_suppl):86–90. SAGE Publications Ltd. doi:10.14504/ajr.8.S2.17.
- Zhao, D., L. Qiuchen, S. Runzhou, L. Yuanzuo, and M. Zhao. 2019. Light harvesting and optical-electronic properties of two quercitin and rutin natural dyes. Applied Sciences 9 (12):2567. Multidisciplinary Digital Publishing Institute. doi:10.3390/app9122567.