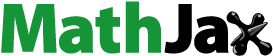
ABSTRACT
Totora is a fast-growing plant with a production potential of up to 58 t of dry mass/ha/year. However, totora has an underappreciated industrial potential. Currently, it is mainly used by indigenous people and occasionally by designers for various design works. If totora is to be used more industrially, we need to thoroughly investigate its properties, especially the mechanical properties of its stems. Therefore, this study presents a comprehensive study of totora stems, namely presents its chemical composition, mechanical properties, and also correlations between dimensions of totora stem and its mechanical and chemical properties. The results showed that totora stems are composed mainly of cellulose (49.62% in totora pith and 40.97% in totora rind). Lignin content of totora rind is 20.14%, whereas totora pith consists only of 6.93% lignin. Totora stems exhibited relatively high mechanical properties, tensile strength was 10.52 MPa, compressive strength 1.65 MPa, and bending strength was 7.75 MPa. It was found that mechanical properties nor chemical properties are not correlated with the area of the stem cross section at a level of 0.05. Only one correlation was found at a level of 0.1.
摘要
托托拉是一种快速生长的植物,其生产潜力高达58吨干物质/公顷/年. 然而,托托拉的工业潜力被低估了. 目前,它主要由土著人使用,偶尔也会被设计师用于各种设计作品. 如果Totora要在工业上得到更多的应用,我们需要彻底研究它的性能,尤其是茎的机械性能. 因此,本研究对Totora茎进行了全面的研究,即介绍了其化学成分、力学性能以及Totora柄的尺寸与其力学和化学性能之间的相关性. 结果表明,托拉茎主要由纤维素组成(托拉髓49.62%,托拉皮40.97%). Totora果皮的木质素含量为20.14%,而Totora髓的木质素仅为6.93%. Totora茎表现出相对较高的机械性能,拉伸强度为10.52MPa,压缩强度为1.65MPa,弯曲强度为7.75MPa. 研究发现,在0.05的水平上,机械性能和化学性能都与茎横截面的面积无关. 在0.1的水平上只发现了一种相关性.
Introduction
Totora (Schoenoplectus californicus (C.A. Mey) Soják) is an emergent macrophyte that grows in lakes and marshlands from California to Chile and some of the Pacific Islands (de Lange et al. Citation1998; Hidalgo-Cordero and García-Navarro Citation2018; Hýsková et al. Citation2020). This plant has been used by several cultures such as the Ohlone in North America, the Incas, and Mapuche in South America, and the Rapanui in Eastern Island, among others (Hidalgo-Cordero Citation2019; Hidalgo-Cordero and García-Navarro Citation2018; Mardorf Citation1985), for making handicrafts, balsas, and in the case of the Uros Islands in Lake Titicaca, their huts, fishing boats, and even the artificial floating islands where they live (Heiser Citation1978; Heyerdahl Citation1971; Mardorf Citation1985).
The long tradition of the use of this plant by several cultures indicates its versatility, resistance, and convenience. Recent studies have pointed out some of its desirable properties from a sustainable point of view such as its fast-growing rate, its resistance against some wood decaying organisms, its low bulk density, restoration, and phytoremediation capabilities, among others, that make this plant an interesting option to be studied for contemporary applications as a sustainable material (Blanco Citation2018; López, Sepúlveda, and Vidal Citation2016; E.; Mejía, Ojeda, and Goyos Citation2019; Pabón et al. Citation2019; Sloey and Hester Citation2018).
Some studies have been conducted about the feasibility of using totora stems to produce contemporary materials such as binderless boards (Hidalgo-Cordero, de Troya, and García-Navarro Citation2019; Hidalgo-Cordero, García-Ortuño, and García-Navarro Citation2020), insulation material (Aza-Medina Citation2016), particle boards (Mejía, Ojeda, and Goyos Citation2019), design objects (Hidalgo-Cordero and García-Navarro Citation2018), among others. These studies have shown the possibilities of using this plant as a sustainable construction material. However, to harness the full potential of this kind of resource, it is necessary to understand its characteristics and how some factors may affect its properties.
Studies have been conducted on the physical and chemical properties of the plant which have identified differences between the plant’s tissues, namely, the rind and the pith, which have different cellulose and lignin content depending on their function within the plant’s structure (Hidalgo-Cordero, Revilla, and García-Navarro Citation2020; Sloey, Howard, and Hester Citation2016). The rind tissue showed a much denser structure and higher lignin concentration than the pith which is a tissue made by air chambers called aerenchyma, which has lower extractives and lignin concentration than the external rind (López, Sepúlveda, and Vidal Citation2016; Pabón et al. Citation2019). Additionally, the physical and mechanical properties of totora stems have been studied. Among the main physical properties is its low density with an average of 80 kg/m3, an average tensile strength of 88 MPa (Hidalgo-Cordero, Revilla, and García-Navarro Citation2020; Mejía, Ojeda, and Goyos Citation2019), and the possibility of acquiring considerable compression and flexion strength when several stems are arranged together using the same techniques used in the totora boat making process (Hidalgo-Castro, Hidalgo-Cordero, and García-Navarro Citation2019).
Additionally, some studies have identified the influence that the location in which totora plants grow may have on their physical characteristics (Pabón et al. Citation2019). Additionally, if we could understand the influence of the chemical characteristics of the plant on its physical properties, we could be able to determine the best uses for this plant depending on its location and other factors.
Totora is a perspective plant, however, the stems are not described sufficiently in the scientific literature so far. In this study, totora stems collected in Ecuador have been studied on their chemical and mechanical properties. The goal of this study was to determine the correlation between the concentration of different compounds and the stems’ mechanical properties. The novelty of this study lies in assessing relationships between totora stems’ mechanical properties and lignin, cellulose, ash, and extractives content both in totora ring and pith.
Materials and methods
Materials
Totora stems () were collected from the Paccha lagoon (2.8832°S, 78.9387°W; 2570 m.a.s.l.) in the Andean region of Ecuador. Stems were cut in June 2018 and immediately dried using the traditional method which consists of leaving the stems to sun dry for 3 weeks and once they have turned yellowish, they were transported to a covered area to complete their drying process for three more weeks at ambient temperature. Once the drying process was completed, they were packed and sent to the Czech Republic via airmail for chemical and mechanical testing.
Methods
Mechanical properties
Compressive strength was measured using a TIRAtest 2850 universal testing machine, 2-cm-long samples of totora stems were used, and compressive strength was calculated as follows:
where Fmax is the maximum force applied to the sample, and S is cross-section of the test specimen.
Tensile strength (depicted on ) was measured using a TIRAtest 2850 universal testing machine, the gauge length was set at 80 mm, and the constant extension rate was 5 mm·min−1. Soft polyurethane foam was used when mounting the totora stems into the gauges in order to minimalize strain concentration in the surrounding gauges. Tensile strength was calculated as follows:
Figure 2. Sample testing figures: compressive strength (a), tensile strength (b), bending strength (c).

where Fmax is the maximum force applied to the sample, and S is cross-section of the test specimen.
A three-point bending test of totora stems was conducted using a TIRAtest 2850 universal testing machine, the distance between the supports (l) was 240 mm. The cross section of the totora stem is in the shape of an equilateral triangle (Hidalgo-Cordero and García-Navarro Citation2018), and therefore bending strength was calculated as follows:
where Fmax is the maximum force applied to the sample, l is the distance between the supports mm) and S is cross-section of the test specimen.
Optical methods
Scanning electron microscopy (SEM) of the totora stems was conducted using a MIRA 3 electron microscope (Tescan Orsay Holding, Brno, Czech Republic) with a secondary electron detector operated at 10 kV acceleration voltage.
The area of the cross section of individual stems was measured by an optical method using ImageJ software, where the cross-section of the stems were scanned at a resolution of 600 DPI.
Chemical properties
Samples for chemical analysis of the essential components were obtained from ruptured samples after measuring mechanical properties. Samples were first ground on an IKA MF 10 BASIC knife mill (Staufen, Germany) and sifted through sieves according to the TAPPI T 11 (2015) . The fraction that passed through the 3.15 mm sieve and remained on the 0.25 mm sieve was used for chemical analyses. In the samples, the content of mineral substances, extractives, lignin, and cellulose were determined. Mineral substances were analyzed in the form of ash according to TAPPI T 211 (2007). The extractable organic part was determined according to TAPPI T 280 (2015) by extraction using a Soxhlet apparatus when a mixture of ethanol and toluene in a ratio of 7:3 was used as the extraction solvent. Seifert cellulose (Seifert Citation1956) and Klason lignin were determined from the extracted remaining part according to TAPPI T 222 om-01.
Statistical analysis
Descriptive statistics, namely arithmetic mean and standard deviation, were calculated in order to characterize measured data of totora stem chemical composition and mechanical properties. Analysis of variance followed by Tukey posthoc test was employed in order to identify statistical difference between the individual arithmetical means, p-value of 0.05 was used. Linear regression models were calculated in order to determine the correlations between the observed characteristics. The regression equations, Pearson correlation coefficients, coefficients of determination and p-values were calculated using Statistica13 software.
Results and discussion
Since totora stems are composed of two different tissues (rind and pith), chemical composition of totora stems was monitored individually for rind and pith. From it can be seen that the highest difference between totora rind and pith is observed in lignin content. While rind lignin content is 20.14% (substantially higher than wheat straw (Zhang et al. Citation2022)), pith lignin content is only 6.93%. On the other hand, the soft tissue of totora pith is characterized by a higher content of cellulose (49.62%). In comparison with wood, a relatively high content of extractives was found in both totora rind and pith (Hill Citation2006; Santos et al. Citation2022).
Table 1. Chemical composition of totora rind and pith.
Other previous study conducted by Hidalgo-Cordero, Revilla, et al., (Citation2020) addressed the chemical differences between totora pith and rind and have obtained similar results, identifying a lignin content of 16.42% in totora rind and 8.9% lignin content in totora pith (). On the other hand, the holocellulose content of totora rind was 57.13% and for the totora pith was 61.03%. Studies about these differences in plant species from the same Cyperaceae family such as papyrus conducted by Rosado et al., (Citation2021) about the characteristics of pith and rind of papyrus stems showed that lignin content is also higher in the rind, with a percentage of 27%, than in the pith, with a percentage of 15.7%. On the other hand, the cellulose content of papyrus pith was much higher, with a percentage of 29.3%, than in the rind, with a percentage of 18.4%.
Table 2. Comparison of chemical properties obtained by different studies.
These studies might indicate that these kinds of plants show higher lignin contents in the rind and higher cellulose content in the pith.
In this study, totora stems with a different area of the cross section were measured. Therefore, it was possible to evaluate the influence of stem cross section on the chemical composition of totora rind () and chemical composition of totora pith (). From these tests, it was observed that for both totora rind and pith with a higher cross section of the stem, the lignin content decreased, whereas ash content increased. In the case of ash content in the rind tissue, the p-value of 0.0543 indicates that the correlation between these two values is significant at a significance level of α = 0.1. Ash content in pith tissue showed a p-value of 0.1206 which could be considered as not statistically significant. A slight decrease in the lignin and extractives content was detected in larger stem cross-section, while a slight increase in cellulose content was detected for both tissues in larger cross-sections.
The increase of ash content in both tissues in relation to the cross section of the plant may indicate a higher concentration of silica content in the base of the plant. Silica has been identified by (Honaine et al., Citation2013) as a product of biomineralization that the plant uses to reinforce its tissues. In that study, older plants showed higher silica content, which may indicate that the biomineralization process occurs progressively in the plant according to its structural reinforcement needs. In this case, the larger cross sections correspond to the base of the plant which is the part that has to withstand most of the physical stresses on the stems. Therefore, higher ash content in larger cross sections may indicate the occurrence of this kind of biomineralization processes.
Lignin and cellulose are the main constituents of plant tissues, while cellulose has been identified as the responsible for the fibrous structure of plants, lignin acts as a binder between these fibers and other plant parts (Fang et al., Citation2022; Zhang et al. Citation2022). In this study, the influence of lignin and cellulose on the mechanical properties of totora stems was analyzed. present correlations between the two main natural fiber biomacromolecules and the mechanical properties of totora stems. It can be seen that with higher content of lignin both in totora rind and pith, tensile strength, compressive strength as well as bending strength of totora stems are increasing. This is not the case for cellulose, where the influence of cellulose content on mechanical properties is unclear. However, none of the monitored correlations could be evaluated as statistically significant at a significance level of α = 0.05. The most significant correlations with p-values of 0.2508 and 0.2145 were found for the correlations between lignin content in totora pith and tensile and compressive strength of totora stem. Although the highest lignin content occurs in the rind of the plant, the mechanical strength of this tissue may be influenced by other factors such as the ash content which may indicate the higher amount of minerals and biomineralizations, which tend to occur on the plants epidermis (Honaine et al., Citation2013), that confers this tissue with more structural strength. In the case of the pith tissue, lignin may have a clearer influence on the structural strength of the stem.
Figure 5. Influence of lignin and cellulose content of totora rind on mechanical properties of totora stems.

Figure 6. Influence of lignin and cellulose content of totora pith on mechanical properties of totora stems.

Other studies have addressed the mechanical strength of totora stems showing different results (). In the case of the study conducted by E. C. Mejía et al., (Citation2017) stems were previously compressed to simulate the way they are used for weaving mats. In this case, the reported average tensile strength was 88.5 MPa. This value is higher because the stem cross section is reduced by the previous compression process.
Table 3. Mean values of mechanical properties of totora stems.
The absolute values of totora stem tensile strength, compressive strength and bending strength are presented in .
Table 4. Comparison of tensile strength properties obtained by different studies.
presents morphology of totora rind, whereas on the cross-section of totora pith is depicted using Scanning Electron Microscopy. It can be seen that totora rind presents pores () that are characteristics for rind of annual plants (Hýsek et al. Citation2018; Rodríguet-Sanz et al. Citation2022). The illustrates completely different structures of totora rind and pith that is also characterized by different chemical composition and mechanical properties (Hidalgo-Cordero and García-Navarro Citation2018).
Conclusions
The present study shows a characterization of totora stems considering the influence of their cross section on the chemical characteristics of each tissue, namely the rind and pith. Important differences were detected between the lignin content of rind (20.14%) and pith (6.93%), indicating the potential benefits that can be obtained when separating these tissues for applications such as biomass refinery where tissues with higher lignin contents are preferred. Ash content in the pith showed a direct correlation with the stem’s cross section at a significance level of α = 0.1. This may indicate that older stems tend to accumulate more minerals, which may help to determine the best harvesting times depending on the desired biomass properties. Mechanical properties of totora stems did not show correlations with the chemical characteristics of rind or pith with significance levels of α = 0.25.
Highlights
Totora stem mechanical and chemical properties were characterized
Totora stems are composed mainly of cellulose (49.62% in pith and 40.97% in rind)
Lignin content of rind is 20.14%, whereas pith consists only of 6.93% lignin
Mechanical nor chemical properties are not correlated with stem cross section
Author’s contribution
Juan Fernando Hidalgo-Cordero: formal analysis, investigation, data curation, writing – original draft preparation, visualization; Miroslav Němec: methodology, validation, formal analysis, investigation, data curation, writing – original draft preparation, visualization; Patricio Hidalgo Castro: formal analysis, investigation, data curation, writing – original draft preparation, visualization; Kateřina Hájková: methodology, formal analysis, investigation, data curation, writing – original draft preparation; Alfredo Ordóñez Castro: investigation, data curation, writing – original draft preparation, visualization; Štěpán Hýsek: conceptualization, methodology, writing – review and editing, resources, supervision
Disclosure statement
No potential conflict of interest was reported by the author(s).
Additional information
Funding
References
- Aza-Medina, L. C. 2016. “La totora como material de aislamiento térmico : Propiedades y potencialidades (Master`s thesis).” Master’s thesis, Universidad Politécnica de Catalunya. http://hdl.handle.net/2117/88419.
- Blanco, J. 2018. “Suitability of Totora (Schoenoplectus Californicus (C.A. Mey.) Soják) for Its Use in Constructed Wetlands in Areas Polluted with Heavy Metals.” Sustainability 11 (1): 19. https://doi.org/10.3390/su11010019.
- de Lange, P. J., R. O. Gardner, P. D. Champion, and C. C. Tanner. 1998. “Schoenoplectus Californicus (Cyperaceae) in New Zealand.” New Zealand Journal of Botany 36 (3): 319–11. https://doi.org/10.1080/0028825X.1998.9512573.
- Fang, Y., L. Chen, J. Wu, and X. Liu 2022. “Fire-Resistant and Antibacterial Chinese Xuan Paper by Fully Bio-Based Chitosan/Phytic Acid Coating on Pulp Fibers.” Industrial Crops and Products 187 (PB): 115456. https://doi.org/10.1016/j.indcrop.2022.115456
- Heiser, C. 1978. “The Totora (Scirpus Californicus) in Ecuador and Peru.” Economic Botany 32 (3): 222–236. https://doi.org/10.1007/BF02864698.
- Heyerdahl, T. 1971. The Ra Expeditions. edited by P. Crampton, Gerge Alle. London: George Allen & Unwin Ltd.
- Hidalgo-Castro, P., J. Hidalgo-Cordero, and J. García-Navarro. 2019. “Estudio del comportamiento físico-mecánico de rollos de totora amarrados: influencia de la tensión de amarre, diámetro y longitud.” DAYA Diseño, Arte y Arquitectura 1 (6): 53–84. https://doi.org/10.33324/daya.vi6.219.
- Hidalgo-Cordero, J. F. 2019. “Constructive applications of totora (Schoenoplectus californicus) in binderless boards (PhD. Thesis).” Doctoral thesis, Universidad Politécnica de Madrid. https://doi.org/10.20868/UPM.thesis.56706
- Hidalgo-Cordero, J. F., M. T. de Troya, and J. García-Navarro. 2019. “Influence of the Hot-Pressing Process on the Durability of Totora (Schoenoplectus Californicus C.A. Mey. Soják) Binderless Boards Against Wood-Decaying Organisms.” Journal of Natural Fibers 18 (11): 1882–1892. https://doi.org/10.1080/15440478.2019.1701611.
- Hidalgo-Cordero, J. F., and J. García-Navarro. 2018. “Totora (Schoenoplectus Californicus (C.A. Mey.) Soják) and Its Potential as a Construction Material.” Industrial Crops and Products 112:467–480. https://doi.org/10.1016/j.indcrop.2017.12.029.
- Hidalgo-Cordero, J. F., T. García-Ortuño, and J. García-Navarro. 2020. “Comparison of Binderless Boards Produced with Different Tissues of Totora (Schoenoplectus Californicus (C.A. Mey) Soják) Stems.” Journal of Building Engineering 27 (June 2019): 100961. https://doi.org/10.1016/j.jobe.2019.100961.
- Hidalgo-Cordero, J. F., E. Revilla, and J. García-Navarro. 2020. “Comparative Chemical Analysis of the Rind and Pith of Totora (Schoenoplectus Californicus) Stems.” Journal of Natural Fibers 17 (7): 954–965. https://doi.org/10.1080/15440478.2018.1541773.
- Hill, C. A. S. 2006. Wood Modification - Chemical, Thermal, and Other Processes. Chichester: John Wiley and Sons Ltd. 260 s., ISBN 0-470-02172-1.
- Honaine, M. F. N. L. Borrelli, M. Osterrieth, and L. Del Rio 2013. “Amorphous Silica Biomineralizations in Schoenoplectus Californicus (Cyperaceae): Their Relation with Maturation Stage and Silica Availability.” Boletin de la Sociedad Argentina de Botanica 48: 247–259.
- Hýsek, Š., M. Podlena, H. Bartsch, C. H. Wenderdel, and M. Böhm. 2018. “Effect of Wheat Husk Surface Pre-Treatment on the Properties of Husk-Based Composite Materials.” Industrial Crops and Products 125:105–113. https://doi.org/10.1016/j.indcrop.2018.08.035.
- Hýsková, P., M. Gaff, J. F. Hidalgo-Cordero, and Š. Hýsek. 2020. “Composite Materials from Totora (Schoenoplectus Californicus. C. A. Mey, Sojak): Is It Worth It?” Composite Structures 232 (September 2019). https://doi.org/10.1016/j.compstruct.2019.111572.
- López, D., M. Sepúlveda, and G. Vidal. 2016. “Phragmites Australis and Schoenoplectus Californicus in Constructed Wetlands: Development and Nutrient Uptake.” Journal of Soil Science and Plant Nutrition 16 (3): 763–777. http://www.scielo.cl/pdf/jsspn/v16n3/aop5516.pdf.
- Mardorf, M. C. 1985. “Artesanía y ecología de la totora (Scirpus sp.) en la provincia de lmbabura, Ecuador.” Sarance Revista Del Instituto Otavaleño de Antropología 10 (10): 11–78. ht tps://do i.org/hdl.handle.net/10469/6697.
- Mejía, C., D. Ojeda, and L. Goyos. 2019. “Mechanical Behavior Under Tension of Schoenoplectus Californicus Fiber.” Journal of Natural Fibers 16 (1): 68–76. https://doi.org/10.1080/15440478.2017.1401506.
- Pabón, G., R. Rodés, L. Pérez, L. Vásquez, and E. Ortega. 2019. “Morphological Relationships in Schoenoplectus Californicus (Cyperaceae) in High-Andean Lakes of Ecuador.” Revista Del Jardin Botanico Nacional 40 :109–119. https://revistas.uh.cu/rjbn/article/view/6655.
- Rodríguet-Sanz, A., C. Fucinos, A. M. Torrado, and M. L. Rua. 2022. “Extraction of the Wheat Straw Hemicellulose Fraction Assisted by Commercial Endo-Xylanases. Role of the Accessory Enzyme Activities.” Industrial Crops and Products 179:s. 114655. https://doi.org/10.1016/j.indcrop.2022.114655.
- Rosado, M. J., F. Bausch, J. Rencoret, G. Marques, A. Gutiérrez, T. Rosenau, A. Potthast, and J. C. Del Río. 2021. “Differences in the Content, Composition and Structure of the Lignins from Rind and Pith of Papyrus (Cyperus Papyrus L.) Culms.” Industrial Crops and Products 174:114226. https://doi.org/10.1016/j.indcrop.2021.114226.
- Santos, M. B., L. Sillero, D. A. Gatto, and J. Labidi. 2022. “Bioactive Molecules in Wood Extractives: Methods of Extraction and Separation, a Review.” Industrial Crops and Products 186. https://doi.org/10.1016/j.indcrop.2022.115231.
- Seifert, K. 1956. “Uber ein neues Verfahren zur Schnellbestimmung Der Rein-Cellulose.” Das Papier 10:301–306y.
- Sloey, T. M., and M. W. Hester. 2018. “Impact of Nitrogen and Importance of Silicon on Mechanical Stem Strength in Schoenoplectus Acutus and Schoenoplectus Californicus: Applications for Restoration.” Wetlands Ecology and Management 26 (3): 459–474. https://doi.org/10.1007/s11273-017-9586-3.
- Sloey, T. M., R. J. Howard, and M. W. Hester. 2016. “Response of Schoenoplectus Acutus and Schoenoplectus Californicus at Different Life-History Stages to Hydrologic Regime.” Wetlands 36 (1): 37–46. https://doi.org/10.1007/s13157-015-0713-8.
- Tappi Test Methods. 2007. “Tappi T 211 Om-02. Ash in Wood, Pulp, Paper and Paperboard: Combustion at 525 °C Georgia: Tappi Press Atlanta.” 7. ISBN 1-930657-33-1.
- Tappi Test Methods. 2015a. Tappi T 11 Wd-76. Sampling and Preparing Wood for Analysis. Georgia: Tappi Press Atlanta. ISBN 1-930657-33-1.
- Tappi Test Methods. 2015d. Tappi T 280 Wd-06. Acetone Extractives of Wood and Pulp. Georgia: Tappi Press Atlanta. ISBN 1-930657-33-1.
- Zhang, L., A. Larsson, A. Moldin, and U. Edlund. 2022. “Comparison of Lignin Distribution, Structure, and Morphology in Wheat Straw and Wood.” Industrial Crops and Products 187. https://doi.org/10.1016/j.indcrop.2022.115432.