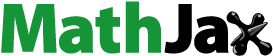
ABSTRACT
A sustainable one-bath dyeing and functionalization process of cotton fabrics with pomegranate rind and onion peel extract using chitosan (CS) biomordant was investigated. Liquid chromatography-mass spectrometry was used to verify the presence of the main colorants, ellagic acid and quercetin, in the prepared extracts of pomegranate rind and onion peel. CS mordanting improved the color strength and wash fastness of pomegranate rind and onion peel dyed fabric by up to 36% and half a rating point, respectively. CS significantly enhanced the UV protection (UPF 50+) and antimicrobial activity (ranges from 93.8 to 99.9%) of dyed fabric. Chitosan mordanting improved the bending rigidity and compression recovery of the cotton fabric, but it also reduced the handle and flexibility of the treated fabrics owing to the formation of CS layer on the cotton fabrics. The dyed fabric also showed remarkable deodorizing (above 80%) and antioxidant (above 90%) properties. Overall, the proposed process could be a sustainable and economical alternative to the conventional metal mordant process.
摘要
采用壳聚糖生物媒染剂对石榴皮和洋葱皮提取物纯棉织物进行可持续的一浴染色和功能化处理. 采用液相色谱-质谱法验证了制备的石榴皮和洋葱皮提取物中主要着色剂鞣花酸和槲皮素的存在. CS媒染使石榴皮和洋葱皮染色织物的色牢度和耐洗牢度分别提高了36%和0.5个评定点. CS显著提高了染色织物的紫外线防护(UPF50+)和抗菌活性(范围从93.8%到99.9%). 壳聚糖媒染提高了棉织物的弯曲刚度和压缩恢复性,但由于在棉织物上形成CS层,也降低了处理织物的手感和柔韧性. 染色织物还表现出显著的除臭(80%以上)和抗氧化(90%以上)性能. 总的来说,所提出的工艺可能是传统金属媒染剂工艺的可持续和经济的替代方案.
Introduction
Cotton forms the foundation of the global textile industry. It is used to make many of the fabrics we use every day and is a versatile material that can be woven, knitted, and dyed in a variety of ways. Cotton fibers provide natural comfort, visual appeal, durability, and value (Hong Citation2014). Among all the natural fibers, cotton ranks second in textiles, with a market share of approximately 23% of the global fiber production in 2019 (Magovac et al. Citation2022). Cotton is a pure cellulosic (92–97% cellulose) natural fiber that is biodegraded by microorganisms that secrete an enzyme called cellulase (Patti and Acierno Citation2022). However, the synthetic dyes commonly used in cotton fabrics influence its biodegradation rate. Zambrano et al. (Citation2021) reported that the presence of C.I. Reactive Blue 19 slowed the rate of biodegradation of cotton fabrics. In addition, the authors observed that the synthetic dyes used to dye the fibers were released into the environment as they degraded. This can cause severe environmental pollution, as these dyes are often toxic and can harm aquatic life. Consequently, many countries have imposed strict bans on goods containing these dyes (Ahlström, Eskilsson, and Björklund Citation2005). Therefore, there is a high demand for greener alternatives. Natural dyes derived from plants are promising alternatives to conventional synthetic dyes (Periyasamy and Militky Citation2020).
In recent years, there has been renewed interest in dyeing textiles with natural dyes owing to their renewability, biodegradability, low allergic reactions, and environmental compatibility (Repon et al. Citation2023; Uddin et al. Citation2022). Many studies have also confirmed that natural dyes possess diverse functionalities such as antibacterial activity, antioxidant activity, UV protection performance, and deodorization performance, which have promising prospects for the development of hygiene-related and medical textiles (Che and Yang Citation2022). The global demand for natural dyes is expected to increase in the near future. The global market share of natural dyes is projected to increase by $5 billion by 2024 (Thakker and Sun Citation2021). However, the limited availability and high cost of natural dyestuffs restrict their large-scale industrial applications (Hou et al. Citation2013). The use of waste materials from agriculture or food processing industries, which are available at little or no cost, to dye textile fibers could make natural dyeing more cost-effective and sustainable (Bechtold et al. Citation2006). Pomegranate rind and onion peel are two common organic wastes that are easily collected from food factories where pomegranates and onions are processed. These waste products contain coloring agents, and their use in the textile industry is promising for the production of naturally dyed fabrics at a lower cost.
Pomegranate (Punica granatum L.) is one of the most popular fruits cultivated and consumed worldly. The fruit is now industrially used to extract juice, which generates a lot of waste, primarily pomegranate rind. This waste accounts for 50% of the three million tons of pomegranate that are processed annually worldwide (Gosset-Erard et al. Citation2021). The pomegranate rind is considered an agro-waste. The rind is a significant source of polyphenolic compounds. Tannins are the major polyphenols in the rind, comprising up to 28% of pomegranate peels (Ben-Ali et al. Citation2018). The tannins contained in pomegranate rinds come in two types: hydrolyzable and condensed tannins. Pomegranate rind contains a lot of hydrolyzable tannins, which dissolve easily in acids and bases. The hydrolyzable tannins are composed of ellagic acid, gallic acid, granatin A, granatin B, punicalin, and punicalagin (Ben-Ali et al. Citation2018). The major colorant in pomegranate rind is ellagic acid, also called C.I. Natural Yellow 7 (Karabulut and Atav Citation2020) ().
Figure 1. Chemical structures of the main coloring components in (a) pomegranate rind and (b) onion peel.

Onions (Allium cepa L.) are one of the most important vegetable crops cultivated and consumed around the world. The production and consumption of onions have increased by approximately 70% over the last 20 years (Fredotović et al. Citation2021). Onion peel accounts for about 10–25% (%w/w basis) of the total weight of the onion and is discarded during peeling (Arroyo Figueroa et al. Citation2022). More than 500,000 tons of onion peel are generated annually in the European Union, mainly in Spain and the Netherlands (Fredotović et al. Citation2021). Papery peel mostly contains flavonoid polyphenols (i.e., quercetin, kaempferol, and myricetin), which are more abundant in the peel than in the edible parts of onions (Lee et al. Citation2014). The major colorant in onion peel is quercetin (R1 = OH and R2 = H in ), also called C.I. Natural Yellow 10 (Chen and Chang Citation2007). The use of onion peel extracts as natural dyes (Rahman, Koh, and Hong Citation2022) and dye lake has been reported (Nguyen and Bechtold Citation2021).
However, most natural dyes are not substantive, which means that they do not bind well to the fibers. This results in low dye-bath exhaustion and poor color fastness in the dyed substrates. To improve these properties, mordants – salts of heavy metals such as copper, iron, chromium, cobalt, nickel, and aluminum – are traditionally used in natural dyeing. Mordants form chemical bonds with both the dye and the fiber, resulting in higher exhaustion and improved fastness. Mordants can also be used to create different shades of color with the same dye. A mordant or dye fixative is used to set (i.e., bind) dyes on fabrics by forming a complex with the dye, which then attaches to the fabric (Zhang et al. Citation2022). Even when used sparingly, metal mordants in textile dyeing exceed environmental release limits. However, their use remains widespread in the industry. This is because metal mordants are highly effective at fixing natural dyes to textile fibers (Manian, Paul, and Bechtold Citation2016). However, handling metal mordants during the mordanting process can be hazardous to human health (Rahman et al. Citation2023). To overcome these problems, the use of biomordants, which are derived from organic materials, has recently received more attention (Che and Yang Citation2022; Periyasamy Citation2022; Zhang et al. Citation2022).
In this study, chitosan, a natural sustainable resource and polycationic biopolymer, was selected as the biomaterial. Chitosan is derived from the deacetylation of chitin, which is the second most abundant biopolysaccharide after cellulose (Dutta, Dutta, and Tripathi Citation2004). Chitin is present in the shells of crustaceans such as crabs, shrimp, and lobsters. The high biocompatibility and biodegradability of chitosan, along with its low cost, make it biologically and chemically suitable for use in various fields such as pharmaceutical and biomedical applications, textile dyeing and finishing, fiber formation, wastewater treatment, paper production, and cosmetics (Dutta, Dutta, and Tripathi Citation2004; Thambiliyagodage et al. Citation2023). The production of and demand for chitosan have increased significantly in recent years owing to its environmental friendliness. Therefore, the use of chitosan as a biomordant in the dyeing of textile fibers with natural dyes is a sustainable practice that can help reduce environmental pollution.
Mordants are chemicals that are used to improve the color and durability of natural dyes on textiles. They can be applied before, during, or after dyeing (Yusuf, Shabbir, and Mohammad Citation2017). Pre-mordanting is the most traditional method, but it requires multiple steps and can be time-consuming (Burkinshaw and Kumar Citation2009). Meta-mordanting, also known as one-bath dyeing, is a more sustainable method that combines the dyeing and mordanting steps into a single bath (Bechtold et al. Citation2003; Hou et al. Citation2013). Chitosan is a natural biomordant that has been shown to be effective in dyeing cotton fabrics with natural dyes. However, there is a lack of research on the use of chitosan to dye cotton fabrics with pomegranate rind and onion peel using a one-bath dyeing process (Grande et al. Citation2023; Haji Citation2017; Jeyakodi Moses and Venkataraman Citation2016; Silva et al. Citation2018; Verma et al. Citation2021).
In this study, the dyeing and functional properties of cotton fabrics treated with colored extracts of pomegranate rind and onion peel were investigated using a one-bath dyeing process with varying concentrations of chitosan. Chitosan was applied to the dyebath using two different methods: addition at the beginning of the dyeing and split addition (20:80) at a 30-min interval after reaching the dyeing temperature. The dyeing properties (i.e., color strength, color levelness, color parameters, color fastness to wash, and color fastness to light) and functional properties (i.e., UV protection, antimicrobial, deodorizing, and antioxidant performance) of the dyed cotton fabrics were assessed.
This study proposes a one-bath dyeing process for cotton fabrics using natural dyes from pomegranate rind, onion peel, and chitosan biomordant as a sustainable alternative to conventional textile dyeing processes. The process eliminates the use of harmful chemicals and solvents, and all components are biodegradable and recyclable. The aqueous extraction process for the dyestuff is simple and environmentally friendly, and the residue can be recycled as compost or animal feed (Bechtold et al. Citation2003). The aqueous dye extract is then applied to cotton dyeing with chitosan biomordant to improve dye fixation. The dyed cotton and spent dyebath after dyeing can also be composted into a nutrient-rich soil amendment, allowing the return of vital organic matter and nutrients to the soil (Wang Citation2010). The spent dyebath could also be reused to dye fabric and other materials. Overall, the proposed one-bath dyeing process has the potential to significantly reduce the environmental impact of the textile industry, particularly for the sustainable dyeing of cotton fabrics at the industrial level.
Materials and methods
Materials
Cotton fabric (ISO 105–F02) was purchased from Testfabrics Inc. (West Pittston, USA). Pomegranate rind (PR) and onion peel (OP) powders were obtained from Naturevibe Botanicals (Maharashtra, India) and Endo & Co., Ltd. (Tokyo, Japan), respectively. Chitosan (CS) (Low-molecular-weight deacetylated chitin) was purchased from Sigma-Aldrich (Steinheim, Germany). Acetic acid was purchased from Daejung Chemicals & Metals Co. Ltd. (Gyeonggi-do, South Korea). Methanol (99.9%) and 2, 2-diphenyl-1-picrylhydrazyl (DPPH 95%) were purchased from Samchun Pure Chemicals Co. Ltd. (Gyeonggi-do, South Korea) and Thermo Fisher Scientific (Ward Hill, MA, USA), respectively. HPLC-grade acetonitrile, dimethyl sulfoxide, methanol, water, and ammonium acetate solutions were purchased from Fisher Scientific Korea, Ltd. (Seoul, South Korea). All the chemicals were used as received. Distilled water was used to extract the plant powders.
Preparation of aqueous extracts
The PR and OP extracts were obtained using an aqueous extraction method. The extracts were obtained at 100°C by adding 0.05 kg of each plant powder to 1 L of distilled water. The extraction was performed in a closed vessel with continuous stirring for 30 min. Finally, the extracts were filtered through Whatman filter paper No. 41 (GE Healthcare Company, Buckinghamshire, UK), and the volume of each extract was adjusted to 1 L with distilled water. The obtained PR and OP extracts had pHs of 3.84 and 4.46 and were used for dyeing the cotton fabric without further adjustment.
Analysis of extracts
UV-visible spectral analysis
The absorbance spectra of the aqueous extracts of PR and OP were measured using a UV-visible spectrophotometer (Agilent 8453, USA). The spectra were obtained in the spectral region of 200–700 nm using a 1-cm quartz cuvette.
LC-MS analysis
Liquid chromatographymass spectroscopy (LC-MS) was performed using an Agilent 1260 series (LC)/6130 B (MS) system. Chromatographic separation was carried out on a Zorbax Eclipse XBD C18 column (150 mm × 2.1 mm, 3.5 pm). The mobile phase comprised 5 mM ammonium acetate (solvent A) and acetonitrile (solvent B). The flow rate of elution was 0.4 mL/min and the gradient was programmed as follows: 0
2 min, isocratic 5% solvent B; 2–4 min, linear gradient 5 to 40% solvent B; 4
7 min, isocratic 40% solvent B; 7–7.5 min linear gradient 40
95% solvent B; 7.5
10 min, isocratic solvent B; 10
10.5 linear gradient 95
5% solvent B; 10.5
15 min, isocratic 5% solvent B. PR and OP extracts were prepared in HPLC-grade water/methanol (1:1) and dimethyl sulfoxide/methanol (1:1), respectively. A 15-pL sample was injected into the column maintained at 30°C with an autosampler. The chromatograms were recorded at 240, 360, 430, 500, and 600 nm using a diode array detector (DAD). The mass spectrometer was operated with the following parameters: electron spray (ES) negative mode, dry gas temperature of 350°C, nebulizer temperature of 35 psig, gas flow of 13 L/min, and scan range of 100
1000 amu (atomic mass unit).
Dyeing
Dyeing was conducted using a one-bath exhaustion process in a laboratory dyeing machine (DLS-6000; Daelim Starlet Co., Ltd., Shiheung-si, South Korea) at a liquor ratio of 1:50. The CS biomordant was added to the dye bath using two different methods: at the beginning of dyeing and after reaching the dyeing temperature. To investigate the mordanting effect of CS on the uptake of dye extracts, the cotton fabrics were treated with different concentrations of CS solutions (0.4 × 10−3%, 2.0 × 10−3%, and 4.0 × 10−3% w/v), which were prepared by dissolving an appropriate amount of CS in a 1.0% v/v acetic acid solution and adding it to the dyebath.
Dyeing was performed at 90°C for 60 min. The dyed fabrics were then rinsed under running water, squeezed, and air-dried.
Color measurement
The color of the dyed fabrics was measured in terms of CIELAB color coordinates (L*, a*, b*, C*, and ho) and Kubelka – Munk K/S values using an illuminant D65, 10° standard observer with specular components included (SCI) on a reflection spectrophotometer (X-Rite, Grand Rapids, MI, USA). The value of L* for a given color varies between 0 (perfect black) and 100 (perfect white) and gives a measure of the lightness of the color. The value of a* is a measure of the red – green character of the color, with positive values for red shades and negative values for green. The value of b* gives the yellow – blue character with positive values for yellow shades and negative for blues. The L*, a*, and b* are calculated using the following Equations (Broadbent Citation2001b):
Where X, Y and Z are tristimulus value of the color, whereas Xn, Yn and Zn are the tristimulus value of the appropriate illuminant (here D65).
The color difference () values between two samples was calculated by using the Equation 4.
less than 1.0 unit corresponds to an acceptable color match (Broadbent Citation2001a).
Where ΔL* = , Δa* =
, and Δb* =
.
The color strength (fk) of the dyed cotton fabric was determined using Equation 5 (Baumann et al. Citation1987):
where ,
, and
indicate the color-matching functions based on a 10° visual field at each wavelength (ISO 7724/1–1984).
The levelness of the dyed fabrics was measured using the reflectance values of eight randomly selected locations on the dyed samples at 10 nm intervals across the visible spectrum (λ = 390–700 nm) with a large aperture (27 mm). The relative unlevelness index (RUI) was calculated using EquationEquation 6(6)
(6) (Chong, Li, and Yeung Citation1992):
where and
indicate the coefficient of variation of reflectance value measured at each wavelength and photopic relative luminous efficiency function, respectively. The suggested interpretations of the RUI values are presented in .
Table 1. Suggested interpretation of relative unlevelness index (RUI) values (Chong, Li, and Yeung Citation1992.).
ATR FT-IR spectroscopy analysis
A Fourier-transform infrared (FT-IR) spectrophotometer (FT-IR-4100, Jasco, Japan) was equipped with attenuated total reflectance (ATR). The spectra were obtained not only for the fabrics but also for the powder form of the extracts (PR and OP) and CS. The spectra were recorded with 32 scans and a resolution of 4 cm−1 using a single reflection horizontal ATR accessory with a diamond ATR crystal fixed at an incident angle of 45°. The energy of the hydrogen bonds (EH) was estimated using Equation 7 (Baseri Citation2020).
where v is the frequency of the bonded OH groups observed in the FTIR spectrum of the sample, vo is the standard frequency corresponding to the free OH groups (at 3650 cm−1), and K is a constant (1/K = 2.625 × 10 2 kJ).
Color fastness test
Color fastness to washing was assessed according to the ISO 105-C06:2010 (A2S, C2S, and E2S). The staining of the adjacent fabric and color changes of the dyed samples were evaluated using gray scales (rating 1–5, from 1: poor to 5: excellent). Color-fastness to light was assessed according to international standards (ISO 105–B02:2014 (E)) using blue scales (ratings 1–8, from 1: poor to 8: excellent).
Evaluation of physical properties of fabrics
The bending, compression, and surface roughness properties of the untreated and CS-mordanted fabrics were assessed by the fabric touch tester (FTT) (SDL Atlas, USA). The fabric sample was cut into an L shape (310 mm × 310 mm, letter “L” width = 110 mm, Cross Area 110 mm × 110 mm) as instructed by the machine manufacturer. The results were computed using the FTT software provided by the manufacturer. Three specimens of each fabric sample were tested to give an average result. The bending and surface roughness properties were measured in the warp (a) and weft (e) directions, respectively.
Determination of functional performances
UV protection performance
The UV transmittance rate (%), UV blocking rate (%), and UV protection factor (UPF) of the undyed, mordanted, and/or dyed cotton fabrics were measured using a UV-Vis-NIR spectrophotometer (PerkinElmer Lambda 1050 with a 150-mm InGaAs integrating sphere, Waltham, USA) in accordance with the Korean standard KS K 0850:2019. According to the standard, fabrics with a UPF value of 15–24, 25–39, and > 40 are considered to provide good, very good, and excellent protection, respectively.
Antimicrobial activity
The antimicrobial activities of the cotton fabrics were determined using the KS K 0693 standard (Hong Citation2014). The microorganisms used for the test were Staphylococcus aureus (S. aureus) (ATCC 6538; gram-positive bacteria) and Klebsiella pneumoniae (K. pneumoniae) (ATCC 4352; gram-negative bacteria). The reduction in bacteria was calculated using EquationEquation 8(8)
(8) :
where B and A represent the proliferating colony-forming units (CFU) from the control and samples after incubation (37°C for 18 h).
Deodorization performance
The deodorization performance of undyed and dyed cotton fabrics was determined against ammonia and acetic acid using the detector tube method. Each of the 1010 cm fabric samples was placed in an airtight Tedlar bag (5 L). Next, the target gases (100 ppm ammonia or 50 ppm acetic acid) were introduced into the bag with 3 L of air. The bags were stably set at ambient conditions (21 ± 5°C) for 2 h, and the gas concentration inside each bag was determined using a detector tube. Deodorization performance was calculated using EquationEquation 9
(9)
(9) :
where A and C represent the gas concentrations in the bag containing the fabric sample and the bag without the fabric sample, respectively.
Antioxidant performance
A stable DPPH free radical scavenging assay was used to measure the radical scavenging activity of the treated cotton fabrics using spectrophotometric analysis based on a previously reported method (Hong Citation2014). Radical scavenging activity was calculated using EquationEquation 10(10)
(10) :
Here, C and S refer to the values of absorbance at 517 nm after 1 h of resting in a dark room for control and test sample in DPPH·/methanol solvents.
Results and discussions
Analysis of extracts
UV-visible spectral analysis
The presence of polyphenolic coloring compounds in the aqueous extracts of the plant parts was confirmed by UV-visible spectroscopy (Shahid-Ul-Islam and Butola Citation2020). The UV – visible absorption spectra of the PR and OP dye extracts over the 200–700 nm range are depicted in . In the case of the PR extract, the maximum absorption peaks were found at 259 and 366 nm, owing to the presence of ellagic acid, as reported previously (Lee et al. Citation2013). The absorption peaks of OP were recorded at 254, 286, and 364 nm, corresponding to flavonoid content. According to existing research (Zhou and Tang Citation2017), flavonoids exhibit two main absorption bands: bands I and II in the 300–400 nm and 240–280 nm ranges, which can be attributed to cinnamoyl (B- and C-rings) and benzoyl (A- and C-rings) systems, respectively (). shows that the principal absorptions of both the PR and OP extracts lie in the UV region, indicating their reasonable UV absorption characteristics. The cotton fabric treated with PR and OP extracts was thus expected to show a reasonable level of UV-blocking performance.
LC-MS analysis
Plants are potential sources of natural colorants and bioactive compounds (Rahman, Koh, and Hong Citation2022). Liquid chromatography-mass spectrometry (LC-MS) analysis was performed to identify the main colorants in PR and OP aqueous extracts. LC-MS analyses of the PR and OP extracts for dyeing are depicted in and 4, respectively. For the PR extract, the mass spectrum () of the resolved peak collected after 5.35 min () showed the presence of ellagic acid at 301.0 m/z [M-H]− (Seeram et al. Citation2005). For the OP extract, the mass spectra () of the resolved peak collected after 6.53 min () confirmed the presence of quercetin at 301.1 m/z [M-H]− (Yang et al. Citation2016). Minor signals in the MS spectra represent contaminants or fragments of the target compounds in the extract. In conclusion, ellagic acid and quercetin colorants were successfully extracted from PR and OP using boiling water for a relatively short time of 30 min.
Dyeing properties
The color strengths of the fabrics dyed with the PR and OP extracts using different concentrations of CS are shown in . The color strength of the CS-mordanted samples was higher than that of the control dyed samples. Clearly, the color strength of the cotton fabric increased with increasing CS concentration. This result can be explained by the biomolecular effect of chitosan. Chitosan is a weak polybase with a pKa of approximately 6.5; therefore, the transition between water-soluble chitosan and water-insoluble chitosan occurs at a pH between 6 and 6.5 (). The cationic nature of chitosan at an acidic pH allows the formation of polyelectrolyte complexes with negatively charged cellulose and plant extract colorants, such as ellagic acid and quercetin, which contain hydroxyl and carbonyl groups (Sami El-Banna et al. Citation2019) (). In terms of color strength improvement by biomordanting, PR exhibited a higher increase than OP: At 4.0 × 10−3 % w/v CS concentration, PR improved by 21.8% (in case of CS addition at the beginning of dyeing) and 36% (in the case of CS addition at dyeing temperature), and OP improved by 16.6% (in the case of CS addition at the beginning of dyeing) and 20.8% (in the case of CS addition at dyeing temperature), respectively. This result can be explained by the planarity and efficiency of CS-colorant complex formation. The main colorant in PR extracts, ellagic acid, has a planar structure due to its sp2 hybridized bondings (Sato and Kataoka Citation1997), whereas the main colorant in onion peel extracts, quercetin, has a non-planar structure (Marković, Dimitrić Marković, and Doličanin Citation2010). This indicates that ellagic acid can easily sit on top of the polymer chains with an aromatic ring parallel to the glucose ring, resulting in stronger interactions. These interactions increase the efficiency of complex formation with chitosan.
Figure 5. Color strength of the cotton fabrics dyed with (a) PR and (b) OP extract using different CS concentrations.

Figure 6. Structural formulae transition of CS between water-soluble form and water-insoluble form at different pHs of the aqueous solution.

Figure 7. Plausible mode of chelation in between the CS-cotton-colorant: (a) PR extract (b) OP extract.

Adding CS at the dyeing temperature resulted in a relatively higher color strength than adding CS at the beginning of dyeing (). In general, the direct addition of a mordant at the initial stage of dyeing in one-bath dyeing causes precipitation of the dyestuff due to its complex formation with the mordant (Bechtold et al. Citation2003). Therefore, the higher color strength of the dyed fabrics when the CS mordant was added to the dyebath at the dyeing temperature can be attributed to the lower precipitation caused by the complexation or aggregation of the dyestuff with CS during the one-bath mordant dyeing process.
The color properties of the cotton fabrics dyed with PR and OP extracts at different concentrations are shown in . The CS mordant significantly influenced the color properties of the dyed fabrics. The L* values obtained for the CS-mordanted samples were lower than those for the control samples (non-mordanted), implying a darker shade for the mordanted samples. Additionally, the L* values gradually decreased with increasing CS concentrations. Interestingly, the CS addition at the dyeing temperature produced slightly darker shades than the CS addition at the beginning of the dyeing process. shows that the color saturation (C*) values of the CS-mordanted samples were higher than those of the control samples. As depicted in , the hue angles of all dyed fabrics are in the red-yellow area, and the CS-mordanted samples exhibit higher hue angles (more yellow) and chroma values than the non-mordanted ones.
Figure 8. CIELAB color coordinates of the cotton fabrics dyed with (a) PR extract and (b) OP extract using different CS concentrations.

Table 2. Color properties and colored images of the cotton fabrics dyed with PR extract and OP extracts using different concentration of CS.
From these results, it can be concluded that CS biomordanting improves the dyeing properties of cotton fabrics with PR and OP extracts. The results also showed that CS addition after reaching the dyeing temperature is preferable in the one-bath dyeing process of cotton with PR and OP extracts using the CS biomordant.
The effect of CS mordanting on the color level is shown in . As the CS concentration increases, the RUI of the dyed fabric increases. The unleveled dyeing at higher CS concentrations could be due to the aggregation of the CS-colorant complex, as described earlier. The precipitate attached to the fabric surfaces during dyeing produces a color spot on the dyed fabrics, leading to higher RUI values (Kartini, Halimah, and Rahayuningsih Citation2021). PR showed higher RUI values than OP. The RUI values of PR dyed fabrics ranged from 0.08 (excellent levelness) to 0.52 (poor levelness), while the values of OP dyed fabrics ranged from 0.03 (excellent levelness) to 0.25 (good levelness). This result can be explained by the difference in the CS mordant effect, which can be verified by the color strength improvement caused by biomordanting, as shown in . After CS biomordanting, the color strength of cotton fabrics dyed with the PR extract exhibited a significant improvement compared to that of cotton fabrics dyed with the OP extract. This implies that the CS-PR complex formation is more effective than the CS-OP complex formation in achieving the desired outcomes. Consequently, uneven spots due to precipitation on the dyed fabrics may become more prevalent. Once a large molecular dye-chitosan complex has been applied to the cotton fiber, its high affinity for the cellulose fiber makes it difficult to distribute the dye evenly; therefore, the probability of uneven dyeing is high.
Figure 9. Levelness of the cotton fabrics dyed with (a) PR and (b) OP extract using different CS concentrations.

For both PR and OP extracts, CS addition at the dyeing temperature resulted in lower RUI values than CS addition at the beginning of dyeing, regardless of the CS concentration. It is presumed that when CS was applied simultaneously at the beginning of dyeing in the one-bath mordant dyeing process, the CS-dye complex formed during the initial stage of dyeing could not penetrate the fiber and remained attached to the fiber surface, resulting in unevenness and higher RUI values. Hence, adding the biomordant during the dyeing process at the dyeing temperature is recommended over adding the mordant at the beginning of the dyeing process in the one-bath dyeing of cotton fabrics. As the dyebath temperature rises to the dyeing temperature (90°C), the dye is sufficiently distributed in the substrate. Subsequently, CS is added at the dyeing temperature to form a dye-CS complex, which can achieve levelness and dyeability.
The color reproducibility of CS-mordanted dyed samples were investigated by carrying out three times of dyeings in different batches at their highest CS mordanting concentration (). Color difference () between the dyeings varied in the range of 0.44 to 1.91 and the average color differences was 1.57 and 1.04 for PR-CS and OP-CS, respectively. In general,
less than 1.0 unit corresponds to an acceptable color match (i.e., reproducible dyeing) between two dyeing. For natural dyeing a somewhat more color difference of
2 could be argued because the use of natural resources makes wider variation due to non-synthetic raw material (Bechtold, Mahmud-Ali, and Mussak Citation2007).
Table 3. Reproducibility of CS mordanted dyed fabrics (mordanting condition: 4.0 × 10−3% w/v CS added after reaching dyeing temperature).
Color fastness
The color fastness to washing and light of the PR- and OP-dyed cotton fabrics were assessed for both control- and CS-mordanted (4.0 × 10−3% w/v) dyed samples (). Compared with the non-mordanted fabrics, the CS-mordanted samples showed a higher wash fastness in fading and staining by a half rating. This improvement in color fastness could be attributed to CS mordanting, which helps the dye molecules bind more strongly to the cotton fibers (). In general, hydrogen bonds and van der Waals forces play important role in binding polyphenolic natural dye to cellulose. However, a strong binding may take place when chitosan is used as the mordant. Chitosan is a renewable polymer with positively charged groups, where ionic interactions may also take place binding the dye (Fang et al. Citation2023; Grande et al. Citation2023). Noticeably, the PR-CS dyed fabric showed a higher washing fastness than the OP-CS dyed fabric. This suggests that more bonds may have formed between the dye, chitosan, and cellulose in the PR-CS dyed fabric. The E2S tests, which involve treating the fabric at higher temperatures (95°C) with sodium perborate, resulted in lower fastness grades than the A2S and C2S test, which involves treating the fabric at a lower temperatures (i.e., 40°C and 60°C, respectively). At higher temperatures, sodium perborate is more effective at bleaching dyes, which can lead to a lower fastness grade (Hossain et al. Citation2022). However, as with most other natural extracts, dyed cotton fabrics with PR and OP extracts exhibited poor light fastness, and CS-mordanted samples did not show any improvement compared to non-mordanted samples (Cristea and Vilarem Citation2006).
Table 4. Color fastness of PR or OP dyed cotton fabrics (with/without CS biomordanting). (mordanting condition: 4.0 × 10−3% w/v CS added after reaching dyeing temperature).
Physical properties of fabrics
The bending, compression, and surface roughness properties of the undyed, control dyed, and CS mordant-dyed fabrics are given in . The CS-mordanted fabrics exhibited higher bending average rigidity, bending work, and compression recovery rate than that of undyed and control dyed fabrics. The increase in bending properties reflects the stiffness of CS-mordanted cotton fabrics. Higher thickness and compression work were registered for the CS mordanted samples. Additionally, surface roughness properties in CS-mordanted samples were higher, showing that CS mordanting has given cotton fabrics an irregular surface covering. These observations are consistent with the results of Kim et al. (Citation2004). Overall, the CS mordanting causes the reduction in handle and flexibility of the treated fabrics owing to the formation of CS layer on the cotton fabrics (Zhao et al. Citation2020)
Table 5. Bending, compression and surface roughness properties of the undyed, control dyed, and CS mordant-dyed fabric. (mordanting condition: 4.0 × 10−3% w/v CS added after reaching dyeing temperature).
FT-IR ATR analysis
The FT-IR ATR spectra of the undyed, control-dyed, and CS-mordant-dyed fabrics with the PR and OP extracts are shown in . The typical major IR absorption bands of cellulose were observed in two wave number regions of 4000–2500 cm−1 and 2000–750 cm−1: hydrogen-bonded OH stretching vibrations at 3110–3670 cm−1, CH2 and CH3 stretching at 2800–3000 cm−1, adsorbed H2O at 1640 cm−1, NH2 deformation corresponding to proteins in cotton at 1537 cm−1, CH and CH2 symmetric bending near 1432 cm−1, OH in plane-bending at 1204 cm−1, C-O-C asymmetrical bridge stretching at 1164 cm−1, asymmetrical in-plane ring stretch at 1056 cm−1, C-O stretching at 1031 cm−1, and asymmetrical out-of-phase ring stretch of C1-O-C4 β glucosidic bonds at 900 cm−1 (Abidi et al. Citation2008; Chung, Lee, and Choe Citation2004; Davulcu et al. Citation2014; Rahman et al. Citation2023).
Figure 10. FT-IR spectra of the undyed, control dyed, and CS mordant-dyed cotton fabrics with (a) PR extract and (b) OP extract.

It was difficult to distinguish the characteristic peaks of CS from those of cellulose in the FT-IR spectrum of the dyed cotton due to the similarity in their chemical structures (Zemljič et al. Citation2014): O-H and N-H stretching at 3390 cm−1, CH2 and CH3 stretching at 2800–3040 cm−1, NH deformation vibration at 1688–1575 cm−1, CH3 symmetrical deformation at 1375 cm−1, C-O-C asymmetrical bridge stretching at 1154 cm−1, and C-O stretching at 1031 cm−1.
No significant changes in the major IR absorption bands of cellulose were observed before and after dyeing, indicating that the dyeing process did not cause noticeable damage to the chemical structure of cellulose. In contrast, new bands around 1723 and 1318 cm−1 were observed for the fabric dyed with the PR extracts, which were probably related to the C=O stretching vibration of ellagic acid in the PR extracts (Davulcu et al. Citation2014). It was also observed that the bands at 3294 (OH stretching), 2929 (CH stretching), 1613 (C=O stretching), 1445 (CH bending), 1318 (C=O stretching), and 1025 (CH bending) cm−1 corresponding to the characteristic peaks of PR extract were more dominant for dyed cotton than for undyed cotton. For the OP extract-dyed fabrics, a new band around 1730 cm−1 was observed, plausibly related to the C=O stretching vibration of quercetin in the OP extracts. Identification of the flavonoid (i.e., quercetin) structure at 1621 cm−1 (Chen and Chang Citation2007) is difficult in OP because the OH bending of absorbed water at approximately 1635 cm−1 also appears in this region (Wang et al. Citation2006). The bands at 3294 (OH stretching), 2929 (CH stretching), 1621 and 1417 (C=C stretching of quinones or conjugated ketones), and 1018 (aliphatic vibration) cm−1 corresponding to the characteristic peaks of the OP extract were more dominant for the dyed cotton than for the undyed cotton. Additionally, the PR extract-dyed fabric showed slightly higher intensities at approximately 3294, 2929, 1635, and 1031 cm−1 than the OP extract-dyed fabric. This may be due to the higher amount of polyphenolic compounds in the PR (Rahman, Koh, and Hong Citation2022) resulting in stronger interactions with the OH and NH2 groups of cellulose and chitosan. The peak intensity at 1537 cm−1 on the dyed cotton with OP-CS and PR-CS is higher than that of undyed cotton fabric. This could be attributed to the absorption band characteristics of the symmetrical bending and stretching of the amine salt owing to the electrostatic nature of the interaction between NH3+ in CS and the hydroxyl groups in cellulose and polyphenols, as shown in (Demarger-Andre and Domard Citation1994; Kumar et al. Citation2011).
shows that the stretching vibration of OH at 3296 cm−1 in the undyed cotton shifted slightly to lower wavenumbers in the dyed cotton after mordanting with CS, suggesting new intermolecular interactions as well as widely distributed H-bond geometries and distances (Zhang, Li, and Yu Citation2011). The hydrogen bond energy (EH) of this band was calculated using (EquationEquation 7(7)
(7) ) and presented in . The CS-mordant-dyed samples exhibited higher EH values than those of the dyed (without mordanting) and undyed samples. This observation is indicative of the OH group reacting with the mordant, resulting in the formation of specific hydrogen-bonding interactions among the OH groups in the cotton cellulose, CS, and phenolic compounds (present in the plant extract), as shown in (Baseri Citation2020; Rahman et al. Citation2023). The planarity of ellagic acid may be a possible reason for the relatively high EH values of the PR-dyed fabrics. Moreover, the highest EH value of the CS-PR-dyed cotton confirmed that the capability of CS-PR complex formation was higher than that of the CS-OP complex.
Table 6. Peak wavenumber and its energy attributed to hydrogen bonds for the undyed, control dyed, and CS-mordant-dyed fabrics.
Functional performances
UV protection performances
The UV blocking rate (%) and UPF of the undyed, control-dyed (sample codes: PR and OP), and CS-mordant-dyed (sample codes: PR-CS and OP-CS) fabrics are listed in .
Table 7. UV protection (%) and UPF of the undyed, control dyed, and CS mordant-dyed fabrics. (mordanting condition: 4.0 × 10−3% w/v CS added after reaching dyeing temperature).
The findings presented in indicate that the undyed cotton fabrics did not exhibit a significant increase in the UV-blocking rate (%) or UPF. However, in the control-dyed cotton fabrics, both the UV blocking rate (%) and UPF were higher than those in the undyed fabrics. Remarkably, the CS-mordant-dyed fabrics displayed a notable increase in the UV-blocking rate (%) and UPF. The following conclusions can be drawn: First, the UV protection properties of the dyed fabrics can be enhanced by both PR and OP dye extracts, owing to their inherent UV absorption properties (Rahman, Koh, and Hong Citation2022). Second, the UV protection properties can be further augmented through CS mordanting, which enhances the absorption of dye extracts in the dye bath () (Kim Citation2006). Third, CS contains two chromophoric groups, N-acetylglucosamine and glucosamine, acquired through the partial deacetylation of chitin, which contribute to the improvement of UV resistance (Mondal and Saha Citation2019). Therefore, employing CS biomordants in PR and OP dyeing processes for cotton fabrics can effectively increase their UV protection performance.
Antimicrobial activity
The bacterial reduction (%) for the undyed, control-dyed, and CS-mordant-dyed fabrics against S. aureus and K. pneumoniae are listed in and the actual images are shown in .
Table 8. Antimicrobial activities of blank, undyed, control dyed, and CS mordant-dyed fabrics. (mordanting condition: 4.0 × 10−3% w/v CS added after reaching dyeing temperature).
Table 9. Actual images of incubated S. aureus and K. pneumoniae bacteria in the blank, undyed, control dyed, and CS mordant-dyed cotton fabrics after incubating for 18 h.
As shown in , the control-dyed and CS mordant-dyed fabrics exhibited a higher bacterial reduction (%) than the undyed fabrics. The PR extract-dyed fabrics showed excellent antimicrobial activity against both S. aureus (gram-positive bacteria) and K. pneumoniae (gram-negative bacteria). This result indicated that the antimicrobial activity of the PR extract alone was sufficiently effective. Ellagic acid, a compound found in the PR extract, has been reported to have antimicrobial activity against both gram-positive and gram-negative bacteria (Lee et al. Citation2013). In contrast, fabric dyed with OP containing quercetin flavonoid is only effective against gram-positive bacteria, such as S. aureus, but not against gram-negative bacteria, such as K. pneumoniae (Rahman, Koh, and Hong Citation2022). The major difference between gram-positive and gram-negative bacteria is the composition of their cell walls. The lipopolysaccharide content in the cell walls of gram-negative bacteria plays an important role in protecting the organisms. Consequently, it is more difficult for low-effective antibacterial agents to cross the cell walls of gram-negative bacteria than that of gram-positive bacteria (Rahman, Koh, and Hong Citation2022). Interestingly, CS mordanting of the cotton fabric dyed with the less effective OP extract significantly improved its antimicrobial activity against gram-negative K. pneumoniae. This can be explained in two ways. First, the mordant effect of CS increased the uptake of the OP extract in the dye bath (). Second, the presence of CS in the dyed fabrics can help inhibit the growth of bacteria. The antimicrobial activity of CS is due to the interaction of the amino group of CS with the cell walls of microorganisms. This interaction is effective against both gram-positive and gram-negative bacteria (Park and Park Citation2010). This indicates that CS can be used to improve the antimicrobial properties of natural dyes that are not as effective against gram-negative bacteria.
Deodorizing and antioxidant performance
shows the deodorizing performance (odor reduction (%)) and antioxidant performance (%) of the undyed, control-dyed, and CS-mordant-dyed fabrics.
Table 10. Deodorizing and antioxidant performance (%) of the undyed, control dyed, and CS mordanted/dyed fabrics. (mordanting condition: 4.0 × 10−3% w/v CS added after reaching dyeing temperature).
The deodorizing performance of the control dyed and CS mordant-dyed fabrics against ammonia gas was significantly higher than that of the undyed fabric. Ammonia is a common foul-smelling gas often used as a model to test the deodorizing properties of fabrics dyed with natural dyes (Wang et al. Citation2020). The higher deodorizing performance of these fabrics against ammonia may be due to the acidic nature of the dyes, which can react with basic ammonia gas to neutralize it and remove odors (Lee et al. Citation2018). This is especially evident for fabrics dyed with the PR extract, which has a higher acidity than the OP extract. The main colorant in the PR extract, ellagic acid, contributes to the deodorizing properties of the fabric (Hwang, Lee, and Kim Citation2008; Lee et al. Citation2013). However, the deodorizing activities of the fabrics dyed with PR and OP against acetic acid were slightly lower than those of the undyed fabric. This is likely because acetic acid molecules are already effectively adsorbed on the surface of the fibrous structures of the cotton fabrics, regardless of whether the fabrics have been treated (Hong Citation2021). Although many natural dyes have been used to deodorize fibers, their deodorization mechanism remains unclear (Wang et al. Citation2020). Further research is required to investigate the deodorization mechanisms of natural dyes. It is also evident from that the dyed fabrics exhibited significantly enhanced antioxidant performance, as measured by the DPPH free radical scavenging assay. The deodorant and antioxidant performances of the PR and OP extracts were so effective that the benefits of CS mordanting were not noticeable. The results demonstrate that PR and OP extracts can be used to dye fabrics with outstanding deodorant and antioxidant properties, regardless of whether CS mordant is used.
Conclusions
Chitosan mordanting of pomegranate rind and onion peel dyed cotton fabric resulted in a significant improvement in color strength (36.01% and 20.80%, respectively), color fastness to washing (half rating improvement), UV protection (UPF 50+), and antimicrobial properties (ranges from 93.8 to 99.9%). The chitosan mordanted and dyed fabric also exhibited excellent deodorizing (above 80%) and antioxidant (above 90%) properties. In addition, chitosan mordanting improved the bending rigidity and compression recovery of the cotton fabric, but it also reduced the handle and flexibility of the treated fabrics owing to the formation of CS layer on the cotton fabrics.
Overall, the chitosan mordanting process was found to be an effective way to improve the dyeing and functional properties of pomegranate rind and onion peel extract dyed cotton fabric, without significantly affecting the fabric’s physical properties. These improvements can be attributed to the effective formation of dye-chitosan complexes on the cotton fabric. The details results suggest that the proposed one-bath dyeing, and functionalization process can be a sustainable alternative to conventional cotton dyeing processes, as it uses natural colorants from agro-waste materials and a biomordant and avoids the use of excess energy, water, and toxic metal mordants. The dyed cotton fabrics with improved functional properties could be used in a variety of applications, such as antimicrobial clothing, sportswear, and medical textiles. The proposed process could be extended to other natural colorants and biomordants, to develop a wider range of sustainable and functional cotton textiles. The dyeing and functional properties of the dyed cotton fabrics could be further improved by optimizing the dyeing process parameters.
Author’s contributions
Md Morshedur Rahman designed the study, conducted the experiments, analyzed the data, participated in the sequence alignment, and drafted the manuscript. Joonseok Koh verified the overall reproducibility of the experiments and participated in the data analysis. Kyung Hwa Hong conceived the study, participated in its design and coordination, and helped to draft the manuscript. All the authors have read and approved the final manuscript.
Highlights
A sustainable natural dyeing and functionalization of cotton fabric has been investigated.
Cotton fabric has been successfully dyed with pomegranate rind and onion peel extract using chitosan as biomordant.
Improved dyeing and functional properties were obtained.
Acknowledgments
This work was supported by the National Research Foundation of Korea (NRF) grant funded by the Korean Government (MSIT) (2020R1A2C1006693). This paper was supported by Konkuk University Researcher Fund in 2023.
Disclosure statement
No potential conflict of interest was reported by the author(s).
Additional information
Funding
References
- Abidi, N., E. Hequet, L. Cabrales, J. Gannaway, T. Wilkins, and L. W. Wells. 2008. “Evaluating Cell Wall Structure and Composition of Developing Cotton Fibers Using Fourier Transform Infrared Spectroscopy and Thermogravimetric Analysis.” Journal of Applied Polymer Science 107 (1): 476–25. https://doi.org/10.1002/app.27100.
- Ahlström, L. H., C. S. Eskilsson, and E. Björklund. 2005. “Determination of Banned Azo Dyes in Consumer Goods.” TrAc Trends in Analytical Chemistry 24 (1): 49–56. https://doi.org/10.1016/j.trac.2004.09.004.
- Arroyo Figueroa, G., J. G. Dzul Cauich, T. Medina Saavedra, and M. I. García Vieyra. 2022. “Dyeing and Colour Fastness in Cotton and Wool, Using Natural Extracts of Sunflower Petals, Onion Peel and Cocoa Shell.” Journal of Natural Fibers 19 (14): 7470–7479. https://doi.org/10.1080/15440478.2021.1950097.
- Baseri, S. 2020. “Eco-Friendly Production of Anti-UV and Antibacterial Cotton Fabrics via Waste Products.” Cellulose 27 (17): 10407–10423. https://doi.org/10.1007/s10570-020-03471-5.
- Baumann, W., B. Groebel, M. Krayer, H. Oesch, R. Brossmann, N. Kleinmeier, and A. T. Leaver. 1987. “Determination of Relative Color Strength and Residual Color Differences by Means of Reflectance Measurements.” Journal of the Society of Dyers and Colourists 103 (2): 100–105.
- Bechtold, T., A. Mahmud-Ali, and R. Mussak. 2007. “Natural Dyes for Textile Dyeing: A Comparison of Methods to Assess the Quality of Canadian Golden Rod Plant Material.” Dyes and Pigments 75 (2): 287–293. https://doi.org/10.1016/j.dyepig.2006.06.004.
- Bechtold, T., R. Mussak, A. Mahmud‐Ali, E. Ganglberger, and S. Geissler. 2006. “Extraction of Natural Dyes for Textile Dyeing from Coloured Plant Wastes Released from the Food and Beverage Industry.” Journal of the Science of Food and Agriculture 86 (2): 233–242. https://doi.org/10.1002/jsfa.2360.
- Bechtold, T., A. Turcanu, E. Ganglberger, and S. Geissler. 2003. “Natural Dyes in Modern Textile Dyehouses—How to Combine Experiences of Two Centuries to Meet the Demands of the Future?” Journal of Cleaner Production 11 (5): 499–509. https://doi.org/10.1016/S0959-6526(02)00077-X.
- Ben-Ali, S., A. Akermi, M. Mabrouk, and A. Ouederni. 2018. “Optimization of Extraction Process and Chemical Characterization of Pomegranate Peel Extract.” Chemical Papers 72 (8): 2087–2100. https://doi.org/10.1007/s11696-018-0427-5.
- Broadbent, A. D. 2001a. “Color Differences and Colorant Formulation.” In Basic Principles of Textile Coloration, 465–492. England: Society of Dyers and Colorists.
- Broadbent, A. D. 2001b. “Color measurement.” In Basic Principles of Textile Coloration, 427–464. England: Society of Dyers and Colorists.
- Burkinshaw, S. M., and N. Kumar. 2009. “The Mordant Dyeing of Wool Using Tannic Acid and FeSo4, Part 1: Initial Findings.” Dyes and Pigments 80 (1): 53–60. https://doi.org/10.1016/j.dyepig.2008.05.008.
- Chen, C., and W. Y. Chang. 2007. “Antimicrobial Activity of Cotton Fabric Pretreated by Microwave Plasma and Dyed with Onion Skin and Onion Pulp Extractions.” Indian Journal of Fibre & Textile Research 32:122–125.
- Che, J., and X. Yang. 2022. “A Recent (2009–2021) Perspective on Sustainable Color and Textile Coloration Using Natural Plant Resources.” Heliyon 8 (10): e10979. https://doi.org/10.1016/j.heliyon.2022.e10979.
- Chong, C. L., S. Q. Li, and K. W. Yeung. 1992. “An Objective Method for the Assessment of Levelness of Dyed Materials.” Journal of the Society of Dyers and Colourists 108 (12): 528–530. https://doi.org/10.1111/j.1478-4408.1992.tb01405.x.
- Chung, C., M. Lee, and E. K. Choe. 2004. “Characterization of Cotton Fabric Scouring by FT-IR ATR Spectroscopy.” Carbohydrate Polymers 58 (4): 417–420. https://doi.org/10.1016/j.carbpol.2004.08.005.
- Cristea, D., and G. Vilarem. 2006. “Improving Light Fastness of Natural Dyes on Cotton Yarn.” Dyes and Pigments 70 (3): 238–245. https://doi.org/10.1016/j.dyepig.2005.03.006.
- Davulcu, A., H. Benli, Y. Şen, and M. İ. Bahtiyari. 2014. “Dyeing of Cotton with Thyme and Pomegranate Peel.” Cellulose 21 (6): 4671–4680. https://doi.org/10.1007/s10570-014-0427-8.
- Demarger-Andre, S., and A. Domard. 1994. “Chitosan Carboxylic Acid Salts in Solution and in the Solid State.” Carbohydrate Polymers 23 (3): 211–219. https://doi.org/10.1016/0144-8617(94)90104-X.
- Dutta, P. K., J. Dutta, and V. S. Tripathi. 2004. “Chitin and Chitosan: Chemistry, Properties, and Applications.” Journal of Scientific & Industrial Research 63:20–31.
- Fang, J., C. Meng, Y. Wang, Y. Yang, L. Han, S. Wang, G. Zhang, Z. Xu, and J. Min. 2023. “Eco-Dyeing and Functional Finishing of Cotton Fabric by Natural Dye Derived from Lotus Seedpod Waste with Chitosan-Assistance.” Fibers and Polymers 24 (4): 1367–1377. https://doi.org/10.1007/s12221-023-00158-z.
- Fredotović, Ž., J. Puizina, M. Nazlić, A. Maravić, I. Ljubenkov, B. Soldo, E. Vuko, and D. Bajić. 2021. “Phytochemical Characterization and Screening of Antioxidant, Antimicrobial and Antiproliferative Properties of Allium× Cornutum Clementi and Two Varieties of Allium Cepa L. Peel Extracts.” Plants 10 (5): 832. https://doi.org/10.3390/plants10050832.
- Gosset-Erard, C., M. Zhao, S. Lordel-Madeleine, and S. Ennahar. 2021. “Identification of Punicalagin as the Bioactive Compound Behind the Antimicrobial Activity of Pomegranate (Punica Granatum L.) Peels.” Food Chemistry 352:129396. https://doi.org/10.1016/j.foodchem.2021.129396.
- Grande, R., R. Raisanen, J. Dou, S. Rajala, K. Malinen, P. A. Nousiainen, and M. Osterberg. 2023. “In situ Adsorption of Red Onion (Allium Cepa) Natural Dye on Cellulose Model Films and Fabrics Exploiting Chitosan as a Natural Mordant.” ACS Omega 8 (6): 5451–5463. https://doi.org/10.1021/acsomega.2c06650.
- Haji, A. 2017. “Improved Natural Dyeing of Cotton by Plasma Treatment and Chitosan Coating; Optimization by Response Surface Methodology.” Cellulose Chemistry and Technology 51 (9–10): 975–982.
- Hong, K. H. 2014. “Preparation and Properties of Multi-Functional Cotton Fabric Treated by Gallnut Extract.” Textile Research Journal 84 (11): 1138–1146. https://doi.org/10.1177/0040517513519007.
- Hong, K. H. 2021. “Sustainable Functional Finishing for Cotton Fabrics Using Screen-Printing Process and Gallotannin.” Fashion and Textiles 8 (1): 1–11. https://doi.org/10.1186/s40691-021-00268-3.
- Hossain, M. Y., T. Jiang, W. Zhu, S. Sarker, M. N. Pervez, M. I. U. Hoque, Y. Cai, and V. Naddeo. 2022. “Green and Sustainable Method to Improve Fixation of a Natural Functional Dye Onto Cotton Fabric Using Cationic Dye-Fixing Agent/D5 Microemulsion.” Journal of Natural Fibers 19 (15): 11283–11298. https://doi.org/10.1080/15440478.2021.2024933.
- Hou, X., X. Chen, Y. Cheng, H. Xu, L. Chen, and Y. Yang. 2013. “Dyeing and UV-Protection Properties of Water Extracts from Orange Peel.” Journal of Cleaner Production 52:410–419. https://doi.org/10.1016/j.jclepro.2013.03.004.
- Hwang, E. K., Y. H. Lee, and H. D. Kim. 2008. “Dyeing, Fastness, and Deodorizing Properties of Cotton, Silk, and Wool Fabrics Dyed with Gardenia, Coffee Sludge, Cassia Tora. L., and Pomegranate Extracts.” Fibers and Polymers 9 (3): 334–340. https://doi.org/10.1007/s12221-008-0054-9.
- Jeyakodi Moses, J., and V. K. Venkataraman. 2016. “Study of Chemical Treated Cotton Fabric for Functional Finishes Using Chitosan.” Journal of Textile and Apparel, Technology and Management 10 (1).
- Karabulut, K., and R. Atav. 2020. “Dyeing of Cotton Fabric with Natural Dyes without Mordant Usage Part I: Determining the Most Suitable Dye Plants for Dyeing and UV Protective Functionalization.” Fibers and Polymers 21 (8): 1773–1782. https://doi.org/10.1007/s12221-020-9365-2.
- Kartini, I., S. N. Halimah, and E. Rahayuningsih. 2021. “Enhanced Wash-Fastness of Cotton Fabric Dyed with a Composite of Chitosan-Natural Dyes Extract of Ceriops Tagal.” IOP Conference Series: Materials Science & Engineering 1053 (1): 012022. https://doi.org/10.1088/1757-899X/1053/1/012022.
- Kim, S. H. 2006. “Dyeing Characteristics and UV Protection Property of Green Tea Dyed Cotton Fabrics-Focusing on the Effect of Chitosan Mordanting Condition.” Fibers and Polymers 7 (3): 255–261. https://doi.org/10.1007/BF02875682.
- Kim, M. J., J. W. Park, and S. H. Lee. 2004. “A Study on the Change of Hand of Chitosan Crosslinked Cotton Fabrics-Effect of Concentration of Epichlorohydrin and Chitosan-.” Fashion & Textile Research Journal 6 (5): 660–666.
- Kumar, S., J. Koh, D. K. Tiwari, and P. K. Dutta. 2011. “Optical Study of Chitosan-Ofloxacin Complex for Biomedical Applications.” Journal of Macromolecular Science, Part A 48 (10): 789–795. https://doi.org/10.1080/10601325.2011.603623.
- Lee, Y. H., E. K. Hwang, Y. M. Baek, M. S. Lee, D. J. Lee, Y. J. Jung, and H. D. Kim. 2013. “Deodorizing and Antibacterial Performance of Cotton, Silk and Wool Fabrics Dyed with Punica Granatum L. Extracts.” Fibers and Polymers 14 (9): 1445–1453. https://doi.org/10.1007/s12221-013-1445-0.
- Lee, K. A., K. T. Kim, H. J. Kim, M. S. Chung, P. S. Chang, H. Park, and H. D. Pai. 2014. “Antioxidant Activities of Onion (Allium Cepa L.) Peel Extracts Produced by Ethanol, Hot Water, and Subcritical Water Extraction.” Food Science & Biotechnology 23 (2): 615–621. https://doi.org/10.1007/s10068-014-0084-6.
- Lee, Y. H., A. L. Kim, Y. G. Park, E. K. Hwang, Y. M. Baek, S. Cho, and H. D. Kim. 2018. “Colorimetric Assay and Deodorizing/Antibacterial Performance of Natural Fabrics Dyed with Immature Pine Cone Extract.” Textile Research Journal 88 (7): 731–743. https://doi.org/10.1177/0040517516688633.
- Magovac, E., B. Vončina, I. Jordanov, J. C. Grunlan, and S. Bischof. 2022. “Layer-By-Layer Deposition: A Promising Environmentally Benign Flame-Retardant Treatment for Cotton, Polyester, Polyamide and Blended Textiles.” Materials 15 (2): 432. https://doi.org/10.3390/ma15020432.
- Manian, A. P., R. Paul, and T. Bechtold. 2016. “Metal Mordanting in Dyeing with Natural Colourants.” Coloration Technology 132 (2): 107–113. https://doi.org/10.1111/cote.12199.
- Marković, Z. S., J. M. Dimitrić Marković, and Ć. B. Doličanin. 2010. “Mechanistic Pathways for the Reaction of Quercetin with Hydroperoxy Radical.” Theoretical Chemistry Accounts 127 (1–2): 69–80. https://doi.org/10.1007/s00214-009-0706-x.
- Mondal, M. I. H., and J. Saha. 2019. “Antimicrobial, UV Resistant and Thermal Comfort Properties of Chitosan-And Aloe Vera-Modified Cotton Woven Fabric.” Journal of Polymers and the Environment 27 (2): 405–420. https://doi.org/10.1007/s10924-018-1354-9.
- Nguyen, H. L., and T. Bechtold. 2021. “Thermal Stability of Natural Dye Lakes from Canadian Goldenrod and Onion Peel as Sustainable Pigments.” Journal of Cleaner Production 315:128195. https://doi.org/10.1016/j.jclepro.2021.128195.
- Park, S. J., and Y. M. Park. 2010. “Eco-Dyeing and Antimicrobial Properties of Chlorophyllin Copper Complex Extracted from Sasa Veitchii.” Fibers and Polymers 11 (3): 357–362. https://doi.org/10.1007/s12221-010-0357-5.
- Patti, A., and D. Acierno. 2022. “Towards the Sustainability of the Plastic Industry Through Biopolymers: Properties and Potential Applications to the Textiles World.” Polymers 14 (4): 692. https://doi.org/10.3390/polym14040692.
- Periyasamy, A. P. 2022. “Natural Dyeing of Cellulose Fibers Using Syzygium Cumini Fruit Extracts and a Bio-Mordant: A Step Toward Sustainable Dyeing.” Sustainable Materials and Technologies 33:e00472. https://doi.org/10.1016/j.susmat.2022.e00472.
- Periyasamy, A. P., and J. Militky. 2020. “Sustainability in Textile Dyeing: Recent Developments.” In Sustainable Textiles: Production, Processing, Manufacturing & Chemistry, edited by S. S. Muthu and M. A. Gardetti, 37–79, Springer Nature Switzerland.
- Rahman, M. M., M. Kim, K. Youm, S. Kumar, J. Koh, and K. H. Hong. 2023. “Sustainable One-Bath Natural Dyeing of Cotton Fabric Using Turmeric Root Extract and Chitosan Biomordant.” Journal of Cleaner Production 382:135303. https://doi.org/10.1016/j.jclepro.2022.135303.
- Rahman, M. M., J. Koh, and K. H. Hong. 2022. “Coloration and Multi-Functionalization of Cotton Fabrics Using Different Combinations of Aqueous Natural Plant Extracts of Onion Peel, Turmeric Root, and Pomegranate Rind.” Industrial Crops and Products 188:115562. https://doi.org/10.1016/j.indcrop.2022.115562.
- Repon, M. R., A. E. Islam, T. Ghorab, M. M. Rahman, and M. M. Rahman. 2023. “Cleaner Pathway for Developing Bioactive Textile Materials Using Natural Dyes: A Review.” Environmental Science and Pollution Research 30 (17): 48793–48823. https://doi.org/10.1007/s11356-023-26131-0.
- Sami El-Banna, F., M. E. Mahfouz, S. Leporatti, M. El-Kemary, and N. A. Hanafy. 2019. “Chitosan as a Natural Copolymer with Unique Properties for the Development of Hydrogels.” Applied Sciences 9 (11): 2193. https://doi.org/10.3390/app9112193.
- Sato, T., and M. Kataoka. 1997. “Molecular Structure and Electronic Spectrum of Ellagic Acid: Semiempirical Molecular Orbital Calculations.” Journal of Heterocyclic Chemistry 34 (2): 665–667. https://doi.org/10.1002/jhet.5570340253.
- Seeram, N., R. Lee, M. Hardy, and D. Heber. 2005. “Rapid Large Scale Purification of Ellagitannins from Pomegranate Husk, a By-Product of the Commercial Juice Industry.” Separation and Purification Technology 41 (1): 49–55. https://doi.org/10.1016/j.seppur.2004.04.003.
- Shahid-Ul-Islam, and B. S. Butola. 2020. “A Synergistic Combination of Shrimp Shell Derived Chitosan Polysaccharide with Citrus sinensis Peel Extract for the Development of Colourful and Bioactive Cellulosic Textile.” International Journal of Biological Macromolecules 158:94–103. https://doi.org/10.1016/j.ijbiomac.2020.04.209.
- Silva, M. G., M. A. S. D. Barros, R. T. R. Almeida, E. J. Pilau, E. Pinto, A. J. S. Ferreira, N. T. Vila, G. Soares, and J. G. Santos. 2018. “Multifunctionalization of Cotton with Onion Skin Extract.” In IOP Conference Series: Materials Science and Engineering 460:012032. https://doi.org/10.1088/1757-899X/460/1/012032.
- Thakker, A. M., and D. Sun. 2021. “Sustainable Plant-Based Bioactive Materials for Functional Printed Textiles.” The Journal of the Textile Institute 112 (8): 1324–1358. https://doi.org/10.1080/00405000.2020.1810474.
- Thambiliyagodage, C., M. Jayanetti, A. Mendis, G. Ekanayake, H. Liyanaarachchi, and S. Vigneswaran. 2023. “Recent Advances in Chitosan-Based Applications—A Review.” Materials 16 (5): 2073. https://doi.org/10.3390/ma16052073.
- Uddin, M. A., M. M. Rahman, A. N. M. A. Haque, S. A. Smriti, E. Datta, N. Farzana, S. Chowdhury, J. Haider, and A. S. M. Sayem. 2022. “Textile Colouration with Natural Colourants: A Review.” Journal of Cleaner Production 349:131489. https://doi.org/10.1016/j.jclepro.2022.131489.
- Verma, M., N. Gahlot, S. S. J. Singh, and N. M. Rose. 2021. “UV protection and antibacterial treatment of cellulosic fibre (cotton) using chitosan and onion skin dye.” Carbohydrate Polymers 257:117612. https://doi.org/10.1016/j.carbpol.2020.117612.
- Wang, Y. 2010. “Fiber and Textile Waste Utilization.” Waste and Biomass Valorization 1 (1): 135–143. https://doi.org/10.1007/s12649-009-9005-y.
- Wang, Q., X. Fan, W. Gao, and J. Chen. 2006. “Characterization of Bioscoured Cotton Fabrics Using FT-IR ATR Spectroscopy and Microscopy Techniques.” Carbohydrate Research 341 (12): 2170–2175. https://doi.org/10.1016/j.carres.2006.04.048.
- Wang, J., B. Tang, W. Bai, X. Lu, X. Wang, and X. Wang. 2020. “Deodorizing for Fiber and Fabric: Adsorption, Catalysis, Source Control and Masking.” Advances in Colloid and Interface Science 283:102243. https://doi.org/10.1016/j.cis.2020.102243.
- Yang, L. L., N. Xiao, X. W. Li, Y. Fan, R. N. Alolga, X. Y. Sun, S. L. Wang, P. Li, and L. W. Qi. 2016. “Pharmacokinetic Comparison Between Quercetin and Quercetin 3-O-β-Glucuronide in Rats by UHPLC-MS/MS.” Scientific Reports 6 (1): 35460. https://doi.org/10.1038/srep35460.
- Yusuf, M., M. Shabbir, and F. Mohammad. 2017. “Natural Colorants: Historical, Processing, and Sustainable Prospects.” Natural Products and Bioprospecting 7 (1): 123–145. https://doi.org/10.1007/s13659-017-0119-9.
- Zambrano, M. C., J. J. Pawlak, J. Daystar, M. Ankeny, and R. A. Venditti. 2021. “Impact of Dyes and Finishes on the Aquatic Biodegradability of Cotton Textile Fibers and Microfibers Released on Laundering Clothes: Correlations Between Enzyme Adsorption and Activity and Biodegradation Rates.” Marine Pollution Bulletin 165:112030. https://doi.org/10.1016/j.marpolbul.2021.112030.
- Zemljič, L. F., J. Volmajer, T. Ristić, M. Bracic, O. Sauperl, and T. Kreže. 2014. “Antimicrobial and antioxidant functionalization of viscose fabric using chitosan–curcumin formulations.” Textile Research Journal 84 (8): 819–830. https://doi.org/10.1177/0040517513512396.
- Zhang, S., F. Li, and J. Y. Yu. 2011. “Novel Cellulose/SPI Blend Bio-Fibers Prepared via Direct Dissolving Approach.” Journal of Engineered Fibers and Fabrics 6 (1): 31–37. https://doi.org/10.1177/155892501100600105.
- Zhang, Y., L. J. Rather, Q. Li, and Q. Li. 2022. “Recent Advances in the Surface Modification Strategies to Improve Functional Finishing of Cotton with Natural Colourants-A Review.” Journal of Cleaner Production 335:130313. https://doi.org/10.1016/j.jclepro.2021.130313.
- Zhao, Z., C. Hurren, M. Zhang, L. Zhou, J. Wu, and L. Sun. 2020. “In situ Synthesis of a Double-Layer Chitosan Coating on Cotton Fabric to Improve the Color Fastness of Sodium Copper Chlorophyllin.” Materials 13 (23): 5365. https://doi.org/10.3390/ma13235365.
- Zhou, Y., and R. C. Tang. 2017. “Natural Flavonoid-Functionalized Silk Fiber Presenting Antibacterial, Antioxidant, and UV Protection Performance.” ACS Sustainable Chemistry & Engineering 5 (11): 10518–10526. https://doi.org/10.1021/acssuschemeng.7b02513.