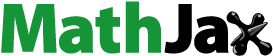
ABSTRACT
The surface roughness of the textile materials is one of the crucial features influencing the appearance and functionality of fabrics and products made of them. The surface roughness of textile materials can be measured using the contact or non-contact methods. In the presented work, the non-contact method using the MicroSpy® Profile profilometer was applied to assess the quality of surface of the cotton woven fabrics of plain and 3/1S twill weave. Determination of surface geometry parameters and functions was done using the Mark III software according to the DIN EN ISO 4287 standard. Results showed that the twill woven fabric is characterized by greater roughness than the plain woven fabric. The profiles created along the warp and weft have different shapes dependably on fabric weave. The autocorrelation function reflects the weave pattern of the woven fabrics. Performed investigations confirmed that the non-contact method of measurement of fabric surface geometry by means of the profilometer provides very detailed and complex data about the fabrics’ surface quality.
摘要
纺织材料的表面粗糙度是影响织物及其制品外观和功能的关键特征之一. 纺织材料的表面粗糙度可以使用接触或非接触方法来测量. 在所介绍的工作中,使用MicroSpy®轮廓轮廓仪的非接触方法用于评估平纹和3/1S斜纹棉布织物的表面质量. 根据DIN EN ISO 4287标准,使用Mark III软件进行表面几何参数和功能的测定. 结果表明,与平纹织物相比,斜纹织物具有较大的粗糙度. 沿着经线和纬线形成的轮廓具有不同的形状,这取决于织物的组织. 自相关函数反映了机织物的编织图案. 所进行的研究证实,通过轮廓仪测量织物表面几何形状的非接触方法提供了关于织物表面质量的非常详细和复杂的数据.
Introduction
Surface quality is one of the basic characteristics of all objects. The surface is a kind of coating “covering” the interior of the object, separating the object from the environment. The surface of an object determines its appearance as well as its interaction with other objects and media surrounding the object, such as liquids and gases. There are many features that characterize the surface quality of objects. It should be mentioned here, among others, hardness, electrostatic properties, wettability, color, friction and roughness (Hasan et al. Citation2008; Liao et al. Citation2011; Sahoo Citation2011). Among the listed properties, roughness is the feature that is the most often identified with the quality of the surface. Surface roughness is commonly understood as the occurrence of unevenness on the surface, which is caused by differences in the height of individual points on the surface. Surface irregularities can be of various nature – shape errors, waviness and roughness, which together form the surface topography. It is defined as the configuration of the surface taking into account its shape as well as the presence and relative position of its characteristic points. When characterizing the surfaces of objects, the terms: “geometric structure” of surface or “surface geometry” are used. As mentioned earlier, the geometric structure of surface consists of three components (Cohen Citation2019; Wieczorowski Citation2013):
shape,
waviness,
roughness.
Measurement of geometric structure of material surface can be done using contact or contactless methods. In contact methods, the sensor in the form of stylus moves across the surface of the material and instrument measures the vertical movement of the sensor caused by the unevenness of the surface. This method provides the value of one parameter: Ra – arithmetic mean of the absolute of the ordinate values within a defined area. It expresses, as an absolute value, the difference in height of each point compared to the arithmetical mean of height of surface (Gadelmawla et al. Citation2002; Matusiak Citation2022). However, the scientists dealing with issues of the geometric structure of surfaces believe that the Ra parameter does not fully characterize the surface topography. With the same value of Ra, one can deal with a very diverse surface geometry (Kacalak et al. Citation2011; Sahoo Citation2011). It can be illustrated by the results of the roughness measurement by means of the KES-FB4. show the shape of the profiles along the warp of woven fabrics of plain and hopsack 2/2 (020) weaves. The value of the SMD (Surface Mean Deviation) parameter determined by the KES-FB4, equivalent to Ra is at the same level for both fabric variants. Whereas, the shape of the profiles is quite different ().
Figure 1. The shape of the profile created along the warp of the plain woven fabric; SMDwarp =18.428 mm.

Figure 2. The shape of the profile created along the warp of the hopsack woven fabric; SMDwarp =18.481 mm.

In non-contact methods, usually the optical instruments are applied. They can measure the surface in three dimensions, providing data on the entire area. Non-contact methods are much more accurate than contact methods. This is due to the fact that in contact methods the size of the measuring tip determines which irregularities are registered by the instrument. Indentations smaller than the size of the sensor are not penetrated by it. This means that the shape of the profile recorded by the contact profilometer may significantly differ from the actual shape of the profile of the measured surface. Moreover, the non-contact methods provide a big number of data characterizing the surface topography. They are values of the parameters of geometric structure of surface, histograms of height distribution, material ratio curve, autocorrelation function, fractal dimension and other graphs and functions. Due to this fact, the non-contact methods of measurement of surface roughness are becoming more and more popular. They are used in measurements more and more often all over the world for an assessment of surface of metal elements, machine parts, dental material, car bodies, etc. (Cohen Citation2019; Grzesik, Rech, and Żak Citation2015; Kłonica Citation2016; Zhu Citation2015).
In measurements of surface roughness of textile materials usually the contact method is applied using the KES (Kawabata Evaluation System) module FB4 (Krucińska, Konecki, and Michalak Citation2006; Maâtoug, Sahnoun, and Sakli Citation2010; Sülara, Öner, and Okur Citation2013). The instruments measure only one roughness parameter – the SMD characterizing the geometrical roughness of textile surface. In the KES-FB4, the measuring sensor is made of the U-shaped steel wire of a diameter of 0.5 mm and width −6 mm ().
The dimensions of the sensor influence a precision of measurement. They eliminate the roughness components of dimensions smaller than the dimensions of the sensor.
The KES system was developed by Kawabata to enable objective – instrumental assessment of the hand of textile materials. The parameters provided by the KES-FB are the base for calculation of the Total Hand Value. The size and sensitivity of the KES-FB4 sensor corresponds to the size and sensitivity of human fingertips. Therefore, the KES-FB4 device allows you to instrumentalize an assessment of feelings when assessing the smoothness of the surface of textile materials, taken into account when assessing the fabric hand. From the scientific and technological point of view the information from the KES-FB4 is absolutely insufficient to fully characterize the surface. KES-FB4 provides information on only one parameter characterizing the topography of fabric surfaces – SMD – geometrical roughness. It corresponds to the Ra which is the arithmetic mean of the absolute ordinate Z(x) (points’ height) within the sampling length/area. This parameter does not say anything about the distribution of the heights of individual points over the entire measured area.
Due to this fact, in the case of textile materials the non-contact methods are more and more often applied in measurement their surface geometry (Calvimontes et al. Citation2009; Calvimontes, Hasan, and Dutschk Citation2010; Hasan et al. Citation2008, Matusiak Citation2022; Ramgulam, Amirbayat, and Porat Citation1993; Vik, Viková, and Hrubosova Citation2014; Matusiak and Frącczak Citation2018). The first non-contact method was presented in 1993 by Ramgulam, Amirbayat and Porat (Citation1993). The device developed by them is able to measure the surface roughness of fabrics using the laser technique. Calvimontes et al. (Citation2010) used the study of the geometric structure of fabric surfaces using the optical method to assess the effect of fabric surface topography on their wettability. Calvimontes et al. (Citation2009) also conducted research on the development of systematics and topographic procedure for the characterization of composite materials in order to study them and identify the impact of production conditions on the quality of the composite surface. Vik et al. (Citation2014) applied the Talysurf profilometer in the study of clothing labels with printed Braille symbols. Matusiak and Frącczak (Citation2018) studied seersucker woven fabrics in order to develop a method of quantifying the seersucker effect of the seersucker woven fabrics.
The non-contact methods are still not widespread in textile engineering. Various instruments are used, but there are not developed and recognized any procedures for preparing samples for testing and testing the geometric structure of the surface of textile materials using the non-contact methods. Whereas, the correct and precise assessment of surface geometry of textile materials is very important both form the scientific and practical points of view. The surface geometry of textile materials influences the functionality of the materials in different aspects (). Especially, the surface geometry of the fabrics influences their comfort-related properties.
Figure 4. Relationship between the geometric structure of surface and functional properties of fabrics.

The aim of the work is to expand the current state of knowledge and acquire new knowledge in the field of the geometric structure of fabric surfaces, research on this structure and show the influence of fabric weave on the geometric structure of its surface. Moreover, the aim of the research was to identify the possibility of using the contactless-optical method for comprehensive characterization of the geometric structure of fabric surface. Surface testing using non-contact methods is not yet widespread in the textile industry. Optical methods are much more precise than contact methods. In addition, optical methods provide information about a number of parameters and functions that comprehensively describe the geometric structure of the surface of textile materials. These parameters and functions are not available when measured with the KES-FB4 instrument, which is widely used in the textile industry.
Which of these parameters are important depends on the tested object and its purpose and desired properties. In the field of textile materials, knowledge on this subject is very limited. I would like to broaden the knowledge in this area.
In the frame of the presented work, two variants of cotton woven fabrics were the objects of the investigations: of the plain and 3/1 S twill weave. Measurement of the geometric structure of surface was done using the non-contact optical method by means of the MicroSpy® Profile profilometer co-operation with the Mark III software.
Materials and methods
In the frame of the presented work, two variants of cotton woven fabrics were measured and analyzed. These were the fabrics made of the same warp and yarn −60 tex OE (Open End) in two weaves: plain (P) and twill (T) 3/1 S. The basic parameters of the investigated fabrics are presented in .
Table 1. The basic structural parameters of the investigated fabrics.
The surface geometry of the fabrics was measured using the MicroSpy Profile profilometer () by FRT (FRT Citation2016; Matusiak Citation2022). It is the instrument utilizing the optical method for precise measurement of the surface characteristics. The profilometer uses the principle of chromatic distance measurement by means of the FRT CWL (chromatic white-light) sensor. It is based on the patented method which makes use of the chromatic aberration (in principle the wavelength-dependent refractive index) of the optical lenses (FRT Citation2016).
The measurement principle is the following. The measured sample is illuminated by focused white light. A passive lens with larger chromatic aberration fans out the white light vertically in different coloured focal points and consequently – in different height. While light beam meets a measured surface, the focused light – in contrast to the unfocused components of the beam – will be reflected optimally. It gets into a miniaturized spectrometer via the same lens and a cable made of glass fibre. The wavelength, corresponding to the colour of the reflected light, results in the distance from sensor to sample.
The data from the profilometer are processed using specialized Mark III software, also by FRT (FRT Citation2018). The scans of fabric surface were done for the area of dimensions of 4.9 cm x 4.9 cm. The lateral resolution of the chromatic aberration confocal head is ≤6 μm, the vertical resolution is 30 nm. For each fabric variant, five samples were measured and analyzed. On the basis of the pictures from the profilometer and the data processing using the Mark III software the parameters and functions characterizing the surface topography were determined according to the DIN EN ISO 4287 standard (DIN Citation2010). Exemplary picture from the profilometer is presented in .
First, the raw data from the profilometer were modified in order to eliminate the invalid data. It was done using the “Area interpolation” option available in the Mark III software. Generally, the interpolation refers to finding the value of given function that exists within a sequence of values – data points. It is a process of inserting an intermediate value between two other values. In the Mark III software it is possible to apply the “Row and column interpolation” or “Area interpolation.” In the “Row and column interpolation” the invalid data are replaced by the average of the linear interpolation in x direction and the linear interpolation in y direction. In the “Area interpolation” method the closed areas of invalid data are corrected by a 2-dimensional interpolation.
Next, the waviness was eliminated. The waviness is not a feature of the fabric surface. It is a result of uneven adhesion of the sample to the table of the profilometer and should be eliminated. In order to eliminate the waviness the cut-of filter λc = 1.4 mm was applied.
A roughness profile is created by removing the long-wavelength component from the primary profile corresponding to the waviness of the profile. In order to create the roughness profile it is necessary to eliminate the waviness using the so called cut-off filter. It refers to the predetermined wavelength to be removed from a primary (unfiltered) profile. There are some principles of establishing the cut-off filter for the stiff objects of solid surface. The textile materials are flexible and porous. Due to this fact, the principles valid for the solid objects are not valid for fabrics. Additionally, the geometry of surface of textile materials is created at three levels of roughness: roughness of fibres surface, roughness of yarns surface and finally – roughness originated from the interweaving of warp and weft. i.e. weave. All these factors should be taken into consideration while measuring the surface of textile materials using the optical methods.
In the presented work the value of the cat-of filter was established according to literature (Matusiak Citation2022), in which the selection of the cut-off filter value was preceded by preliminary research. The exemplary picture of the fabric surface after elimination of waviness is presented in .
Figure 7. Exemplary picture of data from the profilometer after waviness elimination for the twill fabric.

The data prepared in such a way were the objects of further analysis. Using appropriate tools available in the Mark III software, the basic parameters and functions were determined for both variants of woven fabrics. For each specimen, the values of parameters were calculated. Next, the average value from five individual results was calculated as a final result for given variant of fabric.
Results and discussion
By processing the data from the profilometer using the Mark III software, it is possible to determine the values of all parameters described in appropriate standard [20]. In the standard there are defined the formulas used for calculation of particular parameters. In , there are presented the mean values and standard deviation of the selected parameters characterizing the surface geometry of the tested woven fabrics.
Table 2. Results of profilometric measurement of surface geometry of the woven fabrics.
The Ra parameter is an arithmetic mean of the absolute of the ordinate values within a defined area. The Ra parameter is expressed by the following equation:
where:
lr – length of the elementary section,
z(x) – value of ordinates (height of a point on the surface).
The Ra parameter expresses, as an absolute value, the difference in height of each point compared to the arithmetical mean height of the surface ().
The Rq parameter is defined as the root mean square value of the ordinate values within a defined area (EquationEquation 2(2)
(2) ).
It is equivalent to the standard deviation of heights. There are opinions that the Rq parameter expresses the surface roughness better than the Ra parameter. Both, the Ra and Rq are called the height parameters.
The Rz parameter expresses the mean roughness depth. It is calculated as the mean value of 5 maximum peak-to-valley roughness depths in five successive sampling lengths. It is a sum of the Rp and Rv. They represent appropriately the maximum peak height and the maximum valley depth of a surface within the sampling area ().
The Rt parameter represents the sum of the maximum peak height and the maximum valley depth of a surface within the evaluation area ().
The Rsk is a skewness. The Rsk parameter represents the degree of bias of the roughness shape (asperity) and is used to evaluate deviations in the height distribution. It is calculated from the following formula:
An interpretation of the Rsk value is the following:
Rsk = 0: The height distribution symmetric against the mean line,
Rsk > 0: Deviation beneath the mean line,
Rsk < 0: Deviation above the mean line.
The Rku parameter is called kurtosis. Its value is a measure of the sharpness of the roughness profile.
An interpretation of the Rku value is the following:
Rku < 3 : Height distribution is skewed above the mean plane,
Rku = 3: Height distribution is normal (sharp portions and indented portions co-exist),
Rku > 3: Height distribution is spiked.
The Rz, Rp, Rv and Rt parameters belong to the group of the amplitude parameters, whereas the Ra, Rq, Rsk and Rku are in the group of average amplitude parameters.
In the case of both fabric variants, the values of the Rq parameter are higher than the value of the Ra parameter (). Additionally, the values of the height parameters: Ra and Rq for the plain weave fabric are lower than that for the twill fabric. It means that generally, the surface roughness of the twill fabric is greater than the surface roughness of the plain fabric.
The values of the rest of parameters for both variants of the analyzed woven fabric are compared in . In the case of the Rz, Rv and Rt parameters, their values for the twill fabric are higher than that for the plain fabric. Whereas, in the case of the Rp parameter the relation is opposite ().
It means that the roughness depth and depth of valleys on the twill fabric surface is greater than the depth of valleys on the plain fabric surface. Probably, it results from a kind of weave and a way of interlacing of the warp and weft yarns. The differences between the pick height and depth of valley of both fabrics being compared are also clearly visible on the profiles created along the warp and weft of the fabrics ().
Statistical analysis using the one-way ANOVA confirmed that in majority of cases the differences between the values of parameters characterizing the geometry of fabrics’ surface for the plain and twill weave fabrics are statistically significant at the significance level 0.05 (). In , the statistically significant differences are marked by red color. Only in the case of the skewness (Rsk) and kurtosis (Rku) the differences are statistically insignificant.
Table 3. The results of the one-way Anova for the parameters characterizing the surface geometry of the investigated cotton woven fabrics.
Using the Mark III software it is possible to create the profiles of fabric surface in any direction and any place of measured sample. The picture below shows exemplary profile of the fabric and the placement of the profile ().
Figure 12. Exemplary profile of the investigated fabric sample: a) placement of the profile, b) shape of the profile.

Using this option for each fabric sample, profiles have been created both: in the warp and weft directions. As it was mentioned earlier, for each fabric variant five repetitions of measurement have been performed. It is impossible to create the average profiles based on five individual profiles. Due to this fact, the exemplary profiles are shown for both variants of woven fabrics: plain and twill ().
Figure 13. Exemplary profiles of the plain woven fabric: a – along the warp (blue line), b – weft (red line).

Figure 14. Exemplary profiles of the twill woven fabric: a – along the warp (blue line), b – weft (red line).

The shapes of the profiles are in agreement with the values of the amplitude parameters. For the plain woven fabric, the distance between the picks and neighboring valleys is regular along the profiles. The distance between the peaks and valleys in the profile along the warp is greater than the distance in the profile created along the weft (). It is due to the fact that the density of the weft yarn is significantly lower (ca. three times) than the density of the warp yarn (). It is reflected by the shape of the profiles.
In the case of the profiles created for the twill woven fabric their shape is different. The distance between neighboring valleys is much greater than that for the plain woven fabric. It is especially seen for the profile created in the warp direction (). The valley represents the place of the repeat where the thread goes from one side of the fabric to the other. In the 3/1 twill weave there is the following sentence: three warp yarns go over the weft thread and one warp yarn goes under the weft thread. In the weft direction () of the twill fabric the distance between the neighboring valleys is smaller because the density of warp is greater than the density of weft. It means that the number of valleys in the length unit in the profile along the weft is smaller than that in the warp direction.
Due to different weaves of the analyzed fabric variants, the distribution of height on the fabric surface in the frame of investigated sample area is different. It is illustrated by the histograms.
Similarly to the profiles of surface, it is impossible to create the average histogram based on individual histograms for particular specimens. show the exemplary histograms for fabrics of the plain and twill weave.
The Mark III software provides also the information about the most frequent height and histogram maximum. The results read from histograms are presented in . The average of the histogram maximum is at the same level for both variants of analyzed woven fabrics. Significant difference is observed in the aspect of the most frequent height. The most frequent height for the twill weave fabric is three times greater than that for the plain weave fabric.
Table 4. The data from the histograms illustrated the height distribution on the fabric surface.
Using the profilometric measurement and analysis of the results it is also possible to detect the directionality of the surface (Matuszewski Citation2013). For this purpose, the autocorrelation function can be applied. The autocorrelation is the cross-correlation of a signal with itself and thus gives information about the self-similarity. The autocorrelation is a mathematical tool for finding repeating patterns, such as the presence of periodic structures, which has been buried under noise (roughness).
present the exemplary graphs of autocorrelation function for the investigated fabric variants.
It is clearly seen that the autocorrelation function reflects the pattern of weave. In the case of the autocorrelation function for the twill fabric () we can see the directionality of weave. Using the tools available in the Mark III software it is also possible to measure an angle of inclination of the striations on the fabric surface.
In the case of the woven fabric of twill weave, the inclined striations are intentional because they create a design effect. They are visible to the naked eye. However, in many cases, the directionality of the surface is not intentional. It is an error caused by various reasons connected with yarn quality or technological process. In such situation, the detection of directionality is useful in assessment of quality of fabrics and preventing such errors. The directionality of the surface can influence the liquid spreading on the fabric surface, color reflectance and thermal radiation of the fabric (cao et al. Citation2019; Mcdonald Citation2023).
Summing up
Performed investigations confirmed that the non-contact method of surface measurement using the MicrosSpy® Profile profilometer provides various and detailed information about the geometric structure of surface of the woven fabrics. There are a lot of parameters and functions which can be applied to describe the surface geometry of the fabrics. In the case of the cotton woven fabrics being investigated, the obtained results showed a significant differentiation of the surface topography depending on the fabric weave. The purpose and function of the fabric determines which of the parameters and functions characterizing the surface geometry are the most useful. It is a main task of the researchers investigating the surface topography of the fabrics to select tools and parameters which describe the surface geometry in the best way from the point of view of the properties and functions of fabrics and goods made of them.
Highlights
Application of innovative non-contact optical method of measurement of geometric structure of textile materials by means of the profilometer
First such complex and systematic characterization of surface geometry of textile materials
Showing the influence of weave on the surface geometry of cotton woven fabrics
Confirming the usefulness of the profilometric measurements in an assessment a quality of surface of textile materials
Disclosure statement
No potential conflict of interest was reported by the author.
References
- Calvimontes, A., K. Grundke, A. Müller, and M. Stamm. 2009. “Advances for the Topographic Characterisation of SMC Materials.” Materials 2 (3): 1084–16. https://doi.org/10.3390/ma2031084.
- Calvimontes, A., M. M. B. Hasan, and V. Dutschk. 2010. “Effects of Topographic Structure on Wettability of Woven Fabrics.” In Woven Fabric Engineering, edited by P. Dobnik-Dubrovski, 71–92. Rijeka, Croatia: Intech Open.
- Cao, B., Q. Liu, Y. Du, J. L. Roujean, J. P. Gastellu-Etchegorry, I. F. Trigo, W. Zhan, et al. 2019. “A Review of Earth Surface Thermal Radiation Directionality Observing and Modeling: Historical Development, Current Status and Perspectives.” Remote Sensing of Environment 232:111304. https://doi.org/10.1016/j.rse.2019.111304.
- Cohen, D. K. Surface Texture Measurement - Did You Set the Cutoff Lengths Properly? 2019. Michigan Metrology Ltd. Blog. https://michmet.com/?s=Did+You+Set+The+Cutoff+Lengths+Properly%3F.
- DIN (Deutsches Institut fur Normung). 2010. DIN EN ISO 4287, Geometrical Product Specifications (GPS) - Surface Texture: Profile Method - Terms, Definitions and Surface Texture Parameters, German Version
- FRT the art of metrologyTM. 2016. Operating Manual FRT MicroSpy® Profile, Version 2.104, Bergisch Gladbach, Germany
- FRT the art of metrologyTM. 2018. Manual Mark III. Version 3.11 R2T1. Germany: Bergisch Gladbach.
- Gadelmawla, E. S., M. M. Koura, T. M. A. Maksoud, I. M. Elewa, and H. Soliman. 2002. “Roughness Parameters.” Journal of Materials Processing Technology 123 (1): 133–145. https://doi.org/10.1016/S0924-0136(02)00060-2.
- Grzesik, W., J. Rech, and K. Żak. 2015. “Characterization of Surface Textures Generated on Hardened Steel Parts in High-Precision Machining Operations.” International Journal of Advanced Manufacturing Technologies 78 (9–12): 2049–2056. https://doi.org/10.1007/s00170-015-6800-4.
- Hasan, M. M. B., A. Calvimontes, A. Synytska, and V. Dutschk. 2008. “Effects of Topographic Structure on Wettability of Differently Woven Fabrics.” Textile Research Journal 78 (11): 996–1003. https://doi.org/10.1177/0040517507087851.
- Kacalak, W., F. Szafraniec, R. Tomkowski, D. Lipiński, and C. Łukianowicz. 2011. “Metodyka oceny zdolności klasyfikacyjnej parametrów charakteryzujących cechy stereometryczne nierówności powierzchni. (Methodology for evaluating the classification ability of parameters characterizing the stereometric features of surface irregularities).” Pomiary Automaryka Kontrola 57 (5/2011): 542–546.
- Kłonica, M. 2016. “Analysis of Geometric Features of the Surface 316l Steel After Different Machining Tools.” Journal of Technology and Exploitation, Mechanical Engineering 2 (1): 73–79. https://doi.org/10.35784/jteme.512.
- Krucińska, I., W. Konecki, and M. Michalak. 2006. Systemy pomiarowe we włókiennictwie (Measuring Systems in Textile Industry). Lodz, Poland: University of Technology.
- Liao, X., J. Hu, Y. Li, Q. Li, and X. Wu. 2011. “A Review on Fabric Smoothness-Roughness Sensation Studies.” Journal of Fiber Bioengineering & Informatics 4 (2): 105–114. https://doi.org/10.3993/jfbi06201101.
- Maâtoug, N., M. Sahnoun, and F. Sakli. 2010. Comparative Study on the Surface Roughness Evaluation of Weft Knitted Fabrics by TST©, KES FB-4 and the Vibrating Blade Test Benches, Paper presented at International Conference of Applied Research in Textile © CIRAT-4, Gent, Belgium
- Matusiak, M. 2022. “Investigation of Surface Geometry of Seersucker Woven Fabrics. 2022.” AUTEX Research Journal. https://doi.org/10.2478/aut-2022-0023.
- Matusiak, M., and Ł. Frącczak. 2018. “Investigation of 3D Woven Fabric Topography Using Laser-Scanning.” Fibres & Textiles in Eastern Europe 26 (127): 81–88. https://doi.org/10.5604/01.3001.0010.7801.
- Matuszewski, M. 2013. Kierunkowość struktury geometrycznej powierzchni w transformacji warstwy wierzchniej (Directionality of the geometrical structure of the surface in the transformation of the surface layer) Rozprawy Nr 170, Bydgoszcz, Poland: Uniwersytet Technologiczno-Przyrodniczy im. Jana i Jędrzeja Śniadeckich w Bydgoszczy
- Mcdonald, L. 2023. Colour and Directionality in Surface Reflectance, Proceedings of the NANOP 2023 Conference (Nanophotonics and Micro/Nano Optics International Conference), Barcelona, Spain. Accessed Jully 26, 2023 https://www.doc.gold.ac.uk/aisb50/AISB50-S20/aisb50-S20-macdonald-paper.pdf.
- Ramgulam, R. B., J. Amirbayat, and I. Porat. 1993. “Measurement of Fabric Roughness by a Non-Contact Method.” The Journal of the Textile Institute 84 (1): 99–106. https://doi.org/10.1080/00405009308631250.
- Sahoo, P. 2011. “Surface Topography.” In Tribology for Engineers. A Practical Guide, edited by J. P. Davim, 1–39. Cambridge England: Wodhead Publishing.
- Sülara, V., E. Öner, and A. Okur. 2013. “Roughness and Frictional Properties of Cotton and Polyester Woven Fabrics.” Indian Journal of Fibre & Textile Research 30:349–356.
- Vik, M., M. Viková, and Z. Hrubosova. 2014. Surface Topography of Textile Braille Labelling, Paper presented at 7th International Textile, Clothing & Design Conference – Magic World of Textiles, Dubrovnik, Croatia
- Wieczorowski, M. 2013. “Teoretycz ne podstawy przestrzennej analizy nierówności powierzchni (Theoretical foundations of spatial analysis of surface irregularities).” Inżynieria Maszyn 18:3. https://yadda.icm.edu.pl/baztech/element/bwmeta1.element.baztech-c75be068-ca3a-423e-95fd-fa2e231fe39a.
- Zhu, S. 2015. Understanding Engineered Surfaces in Automotive, Quality Magazine. Accessed 3 16 2023. https://www.qualitymag.com/articles/92990-understanding-engineered-surfaces-in-automotive.