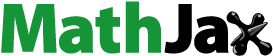
ABSTRACT
Flavonoids in ginkgo biloba L. leaf (abbreviated as GBL) extracts exhibited superior biological activity, but their light color limited their development in the field of textiles. Therefore, this work innovatively used GBL extracts as textile functional finishing agent, through exhaustion dyeing process endowed protein fabrics the multifunction. Flavonoids of GBL in protein fabrics showed outstanding rubbing and washing fastness, becuase of their formed strong affinity by electrostatic attraction, hydrogen bond and van der Waals force. Moreover, the flavonoid content on protein fibers was enhanced by the GBL extract concentration increase, treating time stretch, temperature improve. Fourier-transform infrared spectroscopy and X-ray photoelectron spectroscopy result that flavonoids in GBL extracts adsorbent the fibers. In addition, the treated protein fabrics possessed good antioxidant, antibacterial and heavy metal protective performance. Finally,GBL dyed silk fabrics exhibited stronger functionalities than GBL dyed wool fabrics. This work provides the methods that reuse GBL waste and functionalization of protein fabric.
摘要
银杏叶(GBL)提取物中黄酮类化合物表现出优异的生物活性,但其浅色限制了其在纺织领域的发展. 因此,本工作创新性地使用GBL提取物作为织物功能整理剂,通过穷举染色工艺赋予蛋白质织物多功能性. GBL黄酮类化合物在蛋白质织物中表现出优异的摩擦牢度和洗涤牢度,因为它们通过静电吸引、氢键和范德华力形成强大的亲和力. 此外,随着GBL提取物浓度的增加、处理时间的延长、温度的提高,蛋白质纤维中黄酮类化合物的含量也有所增加. 傅立叶变换红外光谱和X射线光电子能谱结果表明,GBL提取物中的黄酮类化合物对纤维具有吸附性. 此外,经处理的蛋白质织物具有良好的抗氧化、抗菌和重金属防护性能. 最后,GBL染色丝绸织物表现出比GBL染色羊毛织物更强的功能性. 这项工作提供了GBL废物的再利用和蛋白质织物的功能化的方法.
Introduction
Ginkgo biloba L. has been around for 250 million years which is called “living fossil” in Chinese. It is one of the most ancient herb medicinal tree species for treating many diseases (Fang et al. Citation2020; Li et al. Citation2021). In especially ginkgo biloba L. leaves (GBL) are confirmed safe and non-toxic for humans and used for diseases such as Alzheimer’s disease, cardiovascular disease, dementia, memory loss, and cerebral ischemia (Heinonen and Gaus Citation2015). More than 70 kinds of flavonoids have been found in GBL (Zhao et al. Citation2018); they are responsible for many beneficial bioactivities, such as antioxidant (Ražná et al. Citation2020), antibacterial (Zhang et al. Citation2018), detoxification of heavy metal (Mehrandish, Rahimian, and Shahriary Citation2019), antidepressant (Chen et al. Citation2019). The major flavonoids in GBL are the derivatives of quercetin, kaempferol and isorhamnetin, linked with glucose and rhamnose in different linkage forms and quantity (Liu et al. Citation2015). Their chemical structures are given in . It is noted that about 50 million GBL trees have been planted to demand for GBL products each year (Zhang et al. Citation2017), resulting in a huge number of leaves treated through landfills or incineration process as waste resources (Lee, Park, and Chung Citation2017; Zhou et al. Citation2018). However, this treatment method has led to serious environmental pollution, which cannot meet the criteria of green and sustainable development. During the past few decades, GBL wastes have been used in adsorbent of heavy metal (Ma et al. Citation2022), synthesis of carbon quantum dots (Wang et al. Citation2020), production of biohydrogen (Li et al. Citation2020) and so on. But these applications have low utilization rate, accordingly, a reasonable conversion of GBL waste to the valuable products has become an urgent problem.
Inspired by some functionalization textile treated with plant flavonoids (Dong et al. Citation2019; Gu, Dong, and Zhang Citation2022), the flavonoids of GBL waste can be suggested as a promising source of valuable functional finishing agent. Therefore, in this work, GBL waste extracts were treated for protein fabrics as a natural functional agent, because of the high affinity between flavonoids and protein fibers. Protein fabrics (mainly included the wool fabrics and silk fabrics) were one of the most important natural fabrics in the modern textile industry, especially silk fabrics had been regarded as a common fabric for undergarmenst because of its several outstanding properties such as smooth, soft, hygroscopicity and so on (Zhou and Tang Citation2017). Protein fabrics treated by GBL waste extract were endowed with anti-bacterial (Zhang et al. Citation2018), anti-oxidative acting (Ražná et al. Citation2020), and heavy metal protective performance (Cai, Song, and Chang Citation2022). It could protect people from getting infected by harmful microorganisms, prevent the oxidative stress caused by harmful species such as active oxygen radicals, avoid the toxicity of heavy metal ions which might lead to significant mutagenic and carcinogenic effects in the human body (Alonso et al. Citation2013; Jing et al. Citation2017; Zhou et al. Citation2015).
In order to achieve multifunctional of textiles using plant flavonoid compounds.GBL waste extracts containing flavonoid compounds were prepared by ethanol/water extraction method.The chemical structures and flavonoid content were characterized, then GBL waste extracts treated two protein fabrics (wool and silk fabrics). The effects of several main treating factors on the content of flavonoid compounds on protein fabrics were studied. In addition, antimicrobial activities, antioxidant activities and heavy metal protective performances of both protein fabrics were investigated and compared.
Experimental
Materials and chemicals
The fallen leaves of L. ginkgo biloba were collected in mid-November under a female about 15-year-old GBL tree on the campus of Tiangong University. They were firstly treated with warm deionized water repeatedly, and then dried at 30°C, finally grounded into powders before used. Two protein fabrics (wool and silk fabrics) were obtained by Tianjin Renli Woolen Company and Nanning Customs Technical Center respectively. Prior to testing, these fabric samples were washed with sodium dodecyl benzene sulfonate at room temperature for 2 h to remove impurities and air dried. Beef extract, peptone and agar were purchased from Beijing Aoboxing Bio-tech Co., Ltd. (China) and used for the antibacterial tests. H2O2 (30%w⁄w), HCl (36.5%wt), NaOH, Na3PO4, NaNO2, K2Cr2O7, AlCl3, ethanol, pyrogallic acid and potassium ferricyanide were of analytical grade and employed without any treatment. Deionized water was used throughout the study.
Extraction of flavonoid compounds from GBL
The trials of flavonoids extraction from GBL powders were conducted by refluxed boiling methods which were described by Maqbool et al. with slight modification (Maqbool et al. Citation2021). 10.0 g GBL powders were added into aqueous ethanol (80%, V/V) with designed extraction time, ratio of liquid to raw material, pH and extraction temperature. Afterward, the extraction solution filtered through filter and then separated the supernatant by centrifugation (4000 rpm for 20 min). Finally, it was kept as the final extracts (denoted as GBL-e) in a refrigerator at 4°C-5°C for subsequent tests within 3 days.
Determination of the total flavonoids concentrations (TFC)
An aliquot of 0.5 ml GBL-e was added to a 25 ml flask containing 1.0 mL of NaNO2 (5% w/v) and 10 mL of NaOH solution (1.0 M). And then, 1 mL of AlCl3 solution (10%w/v) was added. After mixing for 6 min, 70% (v/v) ethanol aqueous solution was added to the flask and made to the volume. The solution was allowed to keep for 25 min, and the absorbance at 510 nm of the solution sample was detected using a UV-2401 Shimadzu spectrophotometer with 70% (v/v) ethanol aqueous solution as a blank control. Finally, TFC was estimated using rutin as the reference, the calibration equation was expressed as y = 8.752×-0.0023 (R2 = 0.9853), where y was absorbance and x was TFC (Gu, Dong, and Zhang Citation2022).
Dyeing method of both protein fabrics with GBL-e
The overall preparation process of the dyed protein fabrics is illustrated in . Dye bath was prepared by diluting GBL-e in distilled water, flavonoid concentration was adjusted by controlling the ratio of GBL-e and distilled water. Adjustment of pH was made with diluted HCl or NaOH aqueous solution. Two protein fabrics were separately immersed in dye bath at a liquor ratio of 1:30. Dyeing of protein fabrics was carried out a regular exhaustion dyeing process using an Ahiba Nuance ECO infrared laboratory dyeing machine at the requisite temperature for a given time. After dyeing, the resulting protein fabrics were rinsed some times using warm water, followed by dried at 60°C for 15 min. Two protein fabrics (wool and silk fabrics) dyed with GBL-e were correspondingly denoted as GBL-e-W and GBL-e-S. Finally, the flavonoid content on protein fabrics were calculated by an equation: Qfla = (TFC0 - TFC1)V/m, where TFC0 and TFC1 were the TFC values of dye bath before and after dyeing (mgml−1), respectively. V was the volume of the dyed bath (ml). m was the mass of dyed protein fabric (g).
Measurements of protein fabrics dyed with GBL-e
Protein fabrics were respectively analyzed using color strength (K/S), Fourier-transform infrared (FTIR) and X-ray photoelectron spectroscopy (XPS) before and after dyeing procedure. The K/S of the dyed protein fabrics were obtained from a Datacolor 800 spectrophotometer (Datacolor International, USA) between 360 nm to 700 nm, with an illuminant D65 and a 10° standard observer. Higher K/S value demonstrated deeper color of fabrics sample appearance. For FTIR analysis, a Nicolet iS50 FT-IR spectrometer was used for testifying their surface chemical constitution. A K-alpha X-ray photoelectron spectrometer (Thermo Fisher Scientific Inc.,USA) was employed to record the core level spectra of several elements on fibrous samples under 300 W Al K conditions.
Functional properties of dyed protein fabric
The achieved functionalities of dyed protein fabrics were evaluated in terms of antibacterial, antioxidant and heavy metal protective performance.
Antibacterial activity evaluation. Escherichia coli ATCC 8739 (E.coli) and Staphylococcus aureus ATCC 6538 (S.aureus) were applied as model bacteria using a modified ASTM E2149–2013 method (Standard Test Method for Determining the Antimicrobial Activity of Antimicrobial Agents under Dynamic Contact Conditions) (Xu et al. Citation2020). More details regarding the antibacterial activity assessment were shown in our previous work (Dong, Sun, and Gu Citation2023).
Antioxidant activity determination. (i) The hydroxyl radical (OH•) scavenging activity of the GBL dyed protein fabric was determined using a modified test method as reported by Smirnoff et al (Smirnoff and Cumbes Citation1989). In a typical procedure, 50 mg of the dyed protein fabric was immersed in mixed solution containing 2.0 mL of FeSO4 aqueous solution (6.0 mmolL−1) and 2.0 mL of salicylic acid solution (6.0 mmolL−1), followed by the addition of 2.0 mL of H2O2 (6.0 mmolL−1). The resulting mixture was incubated at 37°C for 60 min. The absorbance of the mixture at 510 nm was determined by a UV-2401 spectrophotometer (Shimadzu Co. Japan). The inhibition rate of hydroxyl radicals was calculated as Inhibition% = (1-As/Ac)×100%. Where Ac and As refer to the absorbance of the hydroxyl radical at 510 nm in the absence and presence of the dyed protein fabric, respectively. (ii) The superoxide radical (•O2−) scavenging activity was measured in accordance with a method developed by (Li et al. (Citation2012). In brief, the dyed protein fabric were added into mixture solution containing Tris-HCl buffer (2 mL, 50 mmolL−1, pH 8.2) and pyrogallol (0.5 mL, 6.0 mmolL−1 in 10.0 mmolL−1 HCl), and then the resulting mixture was vigorously shaken at 37°C before being analyzed at 325 nm every 30 sec for 5 min. The slope of the correlation of absorbance with time was calculated. The mixture solution with the untreated protein fabric was used as the control. The superoxide radical inhibition ability is expressed as EquationEquation (1)(1)
(1) :
Where ΔA325 nm,control is the increase in the A325 nm value of the mixture with the untreated protein fabric. ΔA325 nm,sample is the increase in the A325 nm value of the mixture with the dyed protein fabric. t is the time required for the determination (5 min in this case).
Heavy metal protective performance. Hexavalent chromium ion (Cr(VI)) was selected as the representative heavy metal ion which was highly toxic pollutants, the Cr(VI) resistant power of dyed protein fabrics were examined using a regular method described in our previous article (Gu, Dong, and Zhang Citation2022).
Color fastness evaluation
According to Chinese Textiles Test Specification for Color Fastness (GB/T 3921–2008, GB/T 3920–2008 and GB/T 8426–2008), color fastnesses to washing, rubbing and light of GBL-e dyed protein fabrics were correspondingly tested and evaluated.
Result and discussion
Characterization of GBL-e
GBL-e with different extraction times were characterized by UV-Vis and the solid powder of dried GBL-e was analyzed by FTIR. The results are presented in .
UV-Vis spectroscopy is able to determine the chromophore groups and aromatic rings of plant extracts due to electronic transitions in the molecular structure of organic compounds. As shown in , GBL-e displayed two UV-Vis absorption bands at about 260–270 nm and 330–350 nm, respectively. Both absorption bands mainly due to the structural characteristic of flavonoids in GBL which could be corresponded to B-C ring conjugation (cinnamoyl system) and A-C ring conjugation (benzoyl system) (Santos et al. Citation2017), the structure framework of flavonoid is given in illustration of .
The FTIR spectroscopy was once an important analytical technique for the structure of organic compounds in plant extracts. The FTIR spectrum of GBL-e extracts between 500–4000 cm−1 was shown in . The prominent band centered at 3264 cm−1 mainly ascribed to the stretching vibration mode of -OH. The apparent band at 2931 cm−1 could be attributed to the asymmetric stretching vibrations of the -CH group. The combined absorption observed at 1780 and 1604 cm−1 was attributed to C=O and C=C stretching vibrations. The combined band of C-H in-plane bending and skeletal stretching of the aromatic ring occurs around 1393 cm−1. An absorption band in the range of 500–900 cm−1 may be assigned to the C-C out-of-plane bending of the chromone ring. Following the discussion above, the main components of GBL-e extract were flavonoids which contain these typical peaks (Gu, Dong, and Zhang Citation2022; Xu et al. Citation2020).
Dyeing process of GBL-e
To obtain the highest content of the flavonoid of GBL-e on the protein fabrics, the content of flavonoid compounds (Qfla) of the both dyed protein fabrics (GBL-e-W and GBL-e-W) were optimized using a regular exhaustion method, and the results were then measured and presented in .
shows that Qfla value of both GBL-e dyed protein fabrics gradually increased with the extension of dyeing time, and the Qfla values under the GBL-e dosage of 100 ml for GBL-e-S and GBL-e-W at 180 min were 6.38 mgg−1 and 4.53 mgg−1, respectively. The result proposed that the flavonoids in GBL-e constantly adsorbed onto protein fabrics in dyeing process. Generally, flavonoids contained numerous hydroxyl groups and some carboxyl groups in the molecular structure which exhibited a bit acidic character. The protein fiber behaved as an ampholyte because it was composed chiefly of amino acids which bore carboxyl and amino groups, they separately belonged to acidic group and basic group. The carboxylic acid groups conferred a net negative charge to protein fiber above the isoelectric point. Therefore, the protein fiber easily formed strong affinity with flavonoids by electrostatic attraction (Xu et al. Citation2020; Zhou et al. Citation2015). Other multiple interactions also existed between flavonoids and protein fibers. The hydrogen bonding between the oxygen and nitrogen atoms of the peptide backbone in protein fiber and the hydroxyl groups in flavonoid compounds. According to previous study (Santos et al. Citation2017), most of flavonoids were linked with glycoside in different linkage forms and number with high molecular weight. Thus, it also depends on van der Waals forces to bond with protein fibers (). Comparing the adsorption of flavonoids in GBL-e on both protein fibers, it can be noted that the Qfla value of GBL-e-S were larger than GBL-e-W under the same dyeing conditions, which indicated that silk fiber had higher affinity for flavonoids than wool fiber. There were obvious differences in surface morphology, supramolecular structure, crystallinity, orientation and chemical composition. Although wool fiber bore more active groups with strong chemical reactivity than silk fiber, the affinity ability of wool fiber to flavonoids weaker than silk fiber due to small specific surface area and distinct scale layer (Hasni et al. Citation2011; Wang et al. Citation2016; Xu and Chance Citation2005). also presented the enhanced effect of GBL-e dosage on Qfla values. The results indicated that the content of flavonoid compounds on protein fabrics increased with continuously enhanced the GBL-e concentration in dye bath. This was because increasing dye concentration in the bath could improve the driving force of dye molecules to adsorb toward wool surface. Under the optimum GBL-e dosage of 100 ml and the given dyeing time of 120 min, the most suitable dyeing temperature was analyzed.
shows the influence of dyeing temperature on Qfla values of both dyed protein fabrics. Dyed protein fabrics presented rising color strength and flavonoid adsorption with the increasement of dyeing temperature. As the temperature rose, the concentration of GBL-e on the fabric surface increased accordingly and the fiber macromolecular chain moved more drastically, which resulted in higher flavonoid uptake.
Color characteristics and fastness
represents that K/S curves of GBL-e dyed two protein fabrics gradually increased with the extension of dyeing time, proposing that the surface color strength of protein fabrics significantly increased with prolongation of dyeing time. This was because a large proportion of flavonoids in GBL-e were pale yellow in color and their treatment had a certain influence on the appearance of dyed protein fabrics. Color fastness such as rubbing, washing and light fastness of both dyed protein fabrics were listed in . Rubbing and washing fastness of both dyed fabrics exhibited considerably good. However, they had poor light fastness because the flavonoids on protein fabrics were oxidized into quinones, which generated conjugate quinone chromophore under strong light (Ren et al. Citation2016).
Table 1. Color fastnesses of GBL-e dyed two protein fabrics.
Characterization of the GBL-e dyed protein fabrics
In order to gain insight into the adsorption process of flavonoids onto protein fabrics, GBL-e-S and GBL-e-W were characterized using FTIR and XPS, respectively, and compared with the original sample, the results of which are shown in
The FTIR spectra of GBL-e-S and original silk fabric were shown in . Several characteristic absorption peaks of both original protein fabrics located at 1610–1640 cm−1, 1500–1520 cm−1 and 1210–1240 cm−1 were attributed to amide structures of protein fabric (mainly -CONH- group). The band of their disulfide bonds were found to be located between 1000 cm−1 and 1200 cm−1, especially wool fabrics. After dyeing, FTIR spectras of GBL-e-S and GBL-e-W showed absorption peaks at 1700–1750 cm−1, 1600–1650 cm−1 and 1020–1050 cm−1, which were assigned to stretching vibration of OH, CH and C=O in flavonoids. In addition, the hydrogen bonds between the flavonoids and protein fabrics led to an increased width of the absorption peak at 3000–3500 cm−1. There was a characteristic adsorption band between 500 cm−1 and 950 cm−1, which was attributed to the vibration modes of benzene rings of flavonoids. The results demonstrated that the flavonoids in GBL-e had been successfully incorporated with surface of both protein fabrics.
exhibits the wide-range XPS spectra of both original protein fabrics, GBL-e-S and GBL-e-W. In both original silk fabrics, the peaks at 155 eV, 285 eV, 400 eV and 532 eV appeared from S 2p, C 1s, N 1s and O1s signals, respectively (Yu et al. Citation2018). Whereas in GBL-e-S, the C content continuously increased from 67.62% to 82.18% and the O content slightly increased from 16.60% to 18.60%, meanwhile the C element of GBL-e-W increased from 69.83% to 75.37%, and the O element GBL-e-W increased from 18.64% to 24.54%. Protein fabric mainly composed of C, O, N, S and H elements, while flavonoids were mainly composed of C, O and H elements. Therefore, this result indicates that flavonoid molecules in GBL-e are deposited on the both protein fiber fabrics, which is consistent with FTIR analysis.
Functionalities
Antioxidant activity
Human skin is chronically exposed to stress from atmospheric irradiation and other environmental oxidants which can induce various skin pathological conditions (Packer and Valacchi Citation2002). To protect the skin against oxidative stress, the development of antioxidant clothing was a crucial measure. Previous studies had reported that flavonoids from GBL are reported as a kind of effective and highly promising source of antioxidants (Rimkiene et al. Citation2017). It may help in improved cellular tolerance against oxidative stress thereby protecting cell against oxidative damage commonly associated to diseases (Singh et al. Citation2019). showed the antioxidant performance of both GBL-e dyed protein fabrics with different Qfla values by measuring hydroxyl radicals and superoxide radicals inhibition in vitro.
Figure 7. Relationship between Qfla of GBL dyed wool fabrics and Inhibition% for both free radicals.

As seen in , the original protein fabrics (Qfla = 0 mgg−1) had a weak antioxidant ability (both radicals scavenging abilities were less than 15%), possibly due to the existence of carboxyl and amino groups in the protein fibers, which could scavenge a small amount of free radicals. It was obvious that both radicals scavenging abilities of GBL-e dyed protein fabrics gradually increased with an increase in its Qfla value, indicating that the dyed protein fabrics possesses good antioxidant performance. This phenomenon could be attributed to that the flavonoids in GBL-e on dyed protein fabrics acted as strong antioxidant with lower redox potentials (between 0.23 V to 0.75 V), flavonoids were able to contribute hydrogen atoms to highly reactive free radicals and inhibited the free radical chain reaction (Galleano et al. Citation2010; Hidalgo, Sánchez-Moreno, and de Pascual-Teresa Citation2010; Pietta Citation2000), and the aroxyl radical may react with a second radical, acquiring a stable quinone structure ().
Antibacterial activity
Protein fabrics are warm and comfortable material, preferred for manufacture of some garments, however, they always provide a favorable environment for the microorganisms which can generate foul odors, allergic reactions and fiber degradation (Muresan et al. Citation2020). Previous pharmacological studies reported that flavonoids of GBL-e had remarkable antibacterial activity (Sati et al. Citation2019), it could treat some diseases caused by skin pathogens (Chassagne et al. Citation2019). Therefore, protein fabrics subsequently dyed with GBL-e in order to obtain textile materials with antibacterial properties, the results are presented in and .
Figure 9. Photographs of survival E. coli (1) and S. aureus (2) colonies on the agar Petri dish after contacting with undyed silk fabrics (a), GBL-e-S (b), undyed wool fabrics (c) or GBL-e-W (d).

Table 2. Antibacterial properties of both GBL dyed protein fiber fabrics.
It was clearly shown in that the number of bacterial colonies from GBL-e dyed protein fabric samples were much less than those from the original protein fabrics. The percentage of bacterial reduction (W%) of dyed protein fabrics against E. coll and S. aureus is listed in . It shows that W% values of GBL-e-S were of 95.95% and 93.44% for E. coll and S. aureus, respectively, and W% values of GBL-e-W were observed toward E. coli and S. aureus with 85.96% and 82.48%. The results further concluded that both protein fabrics dyed with the GBL-e possessed antibacterial properties against E. coli and S. aureus which are two kinds of common bacteria in human life. There were two reasons for the results (Farhadi et al. Citation2019; Jucá et al. Citation2020): (i) Changing of the membrane permeability. Flavonoids could cause cell wall weakening, consequently membrane damage had occurred and intracellular constituents leaked from the cell. (ii) Nucleic acid synthesis inhibition. Flavonoids were able to change the morphology of bacterial cells and significantly inhibit the global synthesis of DNA and RNA after acting on bacteria. (iii) Inhibition of biological enzymes. The hydroxyl phenolic groups of flavonoid had affinity for bacterial enzymes and thus interfered with enzyme synthesis pathways. In addition, it was also found that the bacterial reduction against E. coli was slightly higher than that against S. aureus. Such a phenomenon could be attributed that the cell wall of the gram-positive (S. aureus) was composed of a thick layer of peptidoglycan, consisting of linear polysaccharide chains cross-linked by short peptides, which led to difficult penetration of flavonoids compared to the gram-negative bacteria (E. coli).
Heavy metal protection
Airborne dust inclusive of heavy metal-containing particulate matter are strongly linked with epidemics of respiratory diseases. It has been confirmed that GBL could protect human organs against heavy metal induced damage. Hence, developing heavy metal protective fabrics by GBL is an effective measure to reduce or prevent respiratory problem risks. In this work, Cr(VI) was selected as the representative heavy metal ion which were highly toxic pollutants. Both GBL-e dyed protein fabrics with similar Qfla values (about 7.20 mgg−1) were applied to remove Cr(VI) species in vitro, and Cr(VI) reduction percentage (R%) and total Cr species removal rate (ɳ%) were measured during the reaction. The original wool fabric was also used in a control experiment for comparison, and the results were shown in .
It was clear in that R% and η% value were less than 20% within 60 min in the presence of both original protein fabric respectively, which might be attributed to the slow adsorption of Cr(VI) species onto protein fiber during the reaction. When GBL-e dyed protein fabrics were added, their R% and η% value gradually increased with the reaction time. At the end of reaction, R% values of both GBL-e dyed protein fabrics were close to 100%, and η% values reached 94.78% for GBL-e-S and 84.26% for GBL-e-W. This demonstrated that both GBL-e dyed protein fabrics could significantly remove Cr(VI) species from water system, which might be closely related to adsorption-coupled reduction mechanism of Cr(VI) by natural flavonoids (Park et al. Citation2007). According to this mechanism, anionic Cr(VI) species might firstly adsorb onto GBL-e dyed protein fabrics, and then be reduced to Cr(III) by adjacent electron-donor groups of flavonoid molecules, this possible mechanism for Cr(VI) removal process by flavonoids on GBL-e dyed protein fiber was described as (Nasrollahzadeh and Sajadi Citation2015). Afterward, the Cr(III) were then removed from the solution through the reaction of Cr(III) ions with abundant functional groups such as OH, C=O and -C-O- in flavonoid molecular structure (Fathy, El-Wakeel, and Abd El-Latif Citation2015; Fedenko, Shemet, and Landi Citation2017), because these groups could be regarded as the oxygen containing ligand sites to react with Cr(III) for producing the stable complexes through intramolecular or/and models. In general, Cr(III) showed much less toxic than Cr(VI). More importantly, there might be a simultaneous coordination of Cr(III) ions with adjacent OH groups in flavonoid molecules and with carboxyl and amino groups in wool structure, which significantly favored the adsorption of Cr(III) ions on the dyed wool fiber to prevent it from entering the human respiratory tract. Moreover, R% and η% value of GBL-e-S were higher than that of GBL-e-W under the same reaction conditions, which indicated that GBL-e-S could remove chromium ions more than GBL-e-W due to the large specific surface area, smooth fiber surface and the orderly ease of chromium ion contact.
Conclusions
In the present work, GBL leaves could extracted with aqueous ethanol (80%, V/V) to obtain GBL-e containing flavonoid compounds, which were applied to prepare multifunctional protein fabrics by an exhaustion dyeing technique. The adsorption of flavonoids and color strength of both GBL-e dyed protein fabrics gradually increased in dyeing process, furthermore high temperature and GBL-e dosage enhanced the adsorption of flavonoids onto protein fabrics. The adsorption path of the protein fabrics with the GBL-e was preliminarily considered to contribute to the formation of stable complexes mainly through electrostatic attraction, van der Waals interactions and hydrogen bonding forces. Silk fabrics exhibited a higher adsorption capability toward flavonoid than wool fabrics, and conferred better antibacterial and antioxidant performance than wool fabrics with similar Qfla value. Furthermore, the GBL dyed protein fabrics might be reduced or prevent the harm of heavy metals to the human body such as respiratory problems and poisoning risk. In conclusion, the extracts from GBL waste could act as functional finishing agent for the simultaneous coloration and functional modification of protein fabrics.
Highlights
Flavonoids were extracted from Binkgo Biloba L. leaf
Waste wool fibers were functionalized with Binkgo Biloba L. leaf extract
Increasing deposition of flavonoids on wool fibres improved health functionality
Acknowledgments
This research was supported in part by the Jiangsu Province Industry-University-Research Cooperation Project (FZ20220571 and FZ20220724), in part by the Jiangsu Advanced Textile Engineering Technology Center Funds (XJFZ/2022/1 and XJFZ/2022/3), in part by the Nantong Basic Science Research Program (MSZ2022162, JC22022049 and JCZ2022109), in part by the Research Project of Jiangsu College of Engineering and Technology (GYKY/2023/6, GYKY/2022/4, GYJY202303 and GYJY202307), in part by Natural Science Foundation of the Jiangsu Higher Education Institutions of China (23KJB540002) and in part by Science and Technology Project of China National Textile And Apparel Council (2022030).
Disclosure statement
No potential conflict of interest was reported by the author(s).
Additional information
Funding
References
- Alonso, C., M. Martí, V. Martínez, L. Rubio, J. L. Parra, and L. Coderch. 2013. “Antioxidant Cosmeto-Textiles: Skin Assessment.” European Journal of Pharmaceutics and Biopharmaceutics 84 (1): 192–16. https://doi.org/10.1016/j.ejpb.2012.12.004.
- Cai, Y. R., Y. Song, and C. Chang. 2022. “Adsorption Properties and Mechanism of Ginkgo Biloba Leaf-Based Materials for Cd (II) in Aqueous Solution.” Environmental Science and Pollution Research 29 (52): 78499–78508. https://doi.org/10.1007/s11356-022-21310-x.
- Chassagne, F., X. Huang, J. T. Lyles, and C. L. Quave. 2019. “Validation of a 16th Century Traditional Chinese Medicine Use of Ginkgo Biloba as a Topical Antimicrobial.” Frontiers in Microbiology 10:775–805. https://doi.org/10.3389/fmicb.2019.00775.
- Chen, P., M. Hei, L. Kong, Y. Liu, Y. Yang, H. Mu, and J. Duan. 2019. “One Water-Soluble Polysaccharide from Ginkgo Biloba Leaves with Antidepressant Activities via Modulation of the Gut Microbiome.” Food & Function 10 (12): 8161–8171. https://doi.org/10.1039/C9FO01178A.
- Dong, Y., J. Gu, P. Wang, and H. Wen. 2019. “Developed Functionalization of Wool Fabric with Extracts of Lycium Ruthenicum Murray and Potential Application in Healthy Care Textiles.” Dyes and Pigments 163:308–317. https://doi.org/10.1016/j.dyepig.2018.12.011.
- Dong, Y., X. Sun, and J. Gu. 2023. “Surface Carboxylation of Hydrophobic Synthetic Fibers for Enhancing Deposition of Reduced Graphene Oxide to Create Highly Conductive and Bactericidal Textiles.” The Journal of the Textile Institute 114 (8): 1135–1145. https://doi.org/10.1080/00405000.2022.2109111.
- Fang, J., Z. Wang, P. Wang, and M. Wang. 2020. “Extraction, Structure and Bioactivities of the Polysaccharides from Ginkgo Biloba: A Review.” International Journal of Biological Macromolecules 162:1897–1905. https://doi.org/10.1016/j.ijbiomac.2020.08.141.
- Farhadi, F., B. Khameneh, M. Iranshahi, and M. Iranshahy. 2019. “Antibacterial Activity of Flavonoids and Their Structure-Activity Relationship: An Update Review.” Phytotherapy Research 33 (1): 13–40. https://doi.org/10.1002/ptr.6208.
- Fathy, N. A., S. T. El-Wakeel, and R. R. Abd El-Latif. 2015. “Biosorption and Desorption Studies on Chromium (VI) by Novel Biosorbents of Raw Rutin and Rutin Resin.” Journal of Environmental Chemical Engineering 3 (2): 1137–1145. https://doi.org/10.1016/j.jece.2015.04.011.
- Fedenko, V. S., S. A. Shemet, and M. Landi. 2017. “UV-Vis Spectroscopy and Colorimetric Models for Detecting Anthocyanin-Metal Complexes in Plants: An Overview of in vitro and in vivo Techniques.” Journal of Plant Physiology 212:13–28. https://doi.org/10.1016/j.jplph.2017.02.001.
- Galleano, M., S. V. Verstraeten, P. I. Oteiza, and C. G. Fraga. 2010. “Antioxidant Actions of Flavonoids: Thermodynamic and Kinetic Analysis.” Archives of Biochemistry and Biophysics 501 (1): 23–30. https://doi.org/10.1016/j.abb.2010.04.005.
- Gu, J., Y. Dong, and J. Zhang. 2022. “Comparative Study of Four Different Flavonoid Compounds‐Containing Plant Extracts Functionalised Waste Wool for Accelerating Aqueous Chromium (VI) Reductive Removal.” Coloration Technology 138 (1): 97–113. https://doi.org/10.1111/cote.12575.
- Hasni, I., P. Bourassa, S. Hamdani, G. Samson, R. Carpentier, and H. A. Tajmir-Riahi. 2011. “Interaction of Milk α-And β-Caseins with Tea Polyphenols.” Food Chemistry 126 (2): 630–639. https://doi.org/10.1016/j.foodchem.2010.11.087.
- Heinonen, T., and W. Gaus. 2015. “Cross Matching Observations on Toxicological and Clinical Data for the Assessment of Tolerability and Safety of Ginkgo Biloba Leaf Extract.” Toxicology 327:95–115. https://doi.org/10.1016/j.tox.2014.10.013.
- Hidalgo, M., C. Sánchez-Moreno, and S. de Pascual-Teresa. 2010. “Flavonoid-Flavonoid Interaction and Its Effect on Their Antioxidant Activity.” Food Chemistry 121 (3): 691–696. https://doi.org/10.1016/j.foodchem.2009.12.097.
- Jing, F., R. Liang, J. Xiong, R. Chen, S. Zhang, Y. Li, and L. Wu. 2017. “MIL-68 (Fe) as an Efficient Visible-Light-Driven Photocatalyst for the Treatment of a Simulated Waste-Water Contain Cr (VI) and Malachite Green.” Applied Catalysis B: Environmental 206:9–15. https://doi.org/10.1016/j.apcatb.2016.12.070.
- Jucá, M. M., F. M. S. Cysne Filho, J. C. de Almeida, D. D. S. Mesquita, J. R. D. M. Barriga, K. C. F. Dias, and S. M. M. Vasconcelos. 2020. “Flavonoids: Biological Activities and Therapeutic Potential.” Natural Product Research 34 (5): 692–705. https://doi.org/10.1080/14786419.2018.1493588.
- Lee, M. E., J. H. Park, and J. W. Chung. 2017. “Adsorption of Pb (II) and Cu (II) by Ginkgo-Leaf-Derived Biochar Produced Under Various Carbonization Temperatures and Times.” International Journal of Environmental Research and Public Health 14 (12): 1528. https://doi.org/10.3390/ijerph14121528.
- Li, X. 2012. “Improved Pyrogallol Autoxidation Method: A Reliable and Cheap Superoxide- Scavenging Assay Suitable for All Antioxidants.” Journal of Agricultural and Food Chemistry 60 (25): 6418–6424. https://doi.org/10.1021/jf204970r.
- Li, W., C. Cheng, G. Cao, and N. Ren. 2020. “Enhanced Biohydrogen Production from Sugarcane Molasses by Adding Ginkgo Biloba Leaves.” Bioresource Technology 298:122523. https://doi.org/10.1016/j.biortech.2019.122523.
- Liu, X. G., S. Q. Wu, P. Li, and H. Yang. 2015. “Advancement in the Chemical Analysis and Quality Control of Flavonoid in Ginkgo Biloba.” Journal of Pharmaceutical and Biomedical Analysis 113:212–225. https://doi.org/10.1016/j.jpba.2015.03.006.
- Li, R., Z. Xia, B. Li, Y. Tian, G. Zhang, M. Li, and J. Dong. 2021. “Advances in supercritical carbon dioxide extraction of bioactive substances from different parts of Ginkgo biloba L.” Molecules 26 (13): 4011. https://doi.org/10.3390/molecules26134011.
- Ma, J., N. L. Ma, D. Zhang, N. Wu, X. Liu, L. Meng, and M. Li. 2022. “Zero Waste Multistage Utilization of Ginkgo Biloba Branches.” Chemosphere 292:133345. https://doi.org/10.1016/j.chemosphere.2021.133345.
- Maqbool, M., S. Ali, M. T. Hussain, A. Khan, and S. Majeed. 2021. “Comparison of Dyeing and Functionalization Potential of Some Selected Plant Extracts Applied on Cotton Fabric.” Journal of Natural Fibers 18 (1): 42–50. https://doi.org/10.1080/15440478.2019.1612304.
- Mehrandish, R., A. Rahimian, and A. Shahriary. 2019. “Heavy Metals Detoxification: A Review of Herbal Compounds for Chelation Therapy in Heavy Metals Toxicity.” Journal of Herbmed Pharmacology 8 (2): 69–77. https://doi.org/10.15171/jhp.2019.12.
- Muresan, E. I., M. Diaconu, C. Zaharia, G. Rosu, A. Danila, and A. Pui. 2020. “Bioactive Textiles Obtained by Using Aqueous Extracts of Vine Leaves.” Fibers and Polymers 21 (11): 2505–2512. https://doi.org/10.1007/s12221-020-1153-5.
- Nasrollahzadeh, M., and S. M. Sajadi. 2015. “Green Synthesis of Copper Nanoparticles Using Ginkgo Biloba L. Leaf Extract and Their Catalytic Activity for the Huisgen [3+2] Cycloaddition of Azides and Alkynes at Room Temperature.” Journal of Colloid and Interface Science 457:141–147. https://doi.org/10.1016/j.jcis.2015.07.004.
- Packer, L., and G. Valacchi. 2002. “Antioxidants and the Response of Skin to Oxidative Stress: Vitamin E as a Key Indicator.” Skin Pharmacology and Applied Skin Physiology 15 (5): 282–290. https://doi.org/10.1159/000064531.
- Park, D., S. R. Lim, Y. S. Yun, and J. M. Park. 2007. “Reliable Evidences That the Removal Mechanism of Hexavalent Chromium by Natural Biomaterials is Adsorption-Coupled Reduction.” Chemosphere 70 (2): 298–305. https://doi.org/10.1016/j.chemosphere.2007.06.007.
- Pietta, P. G. 2000. “Flavonoids as Antioxidants.” Journal of Natural Products 63 (7): 1035–1042. https://doi.org/10.1021/np9904509.
- Ražná, K., Z. Sawinska, E. Ivanišová, N. Vukovic, M. Terentjeva, M. Stričík, and M. Kačániová. 2020. “Properties of Ginkgo Biloba L.: Antioxidant Characterization, Antimicrobial Activities, and Genomic microRna Based Marker Fingerprints.” International Journal of Molecular Sciences 21 (9): 3087. https://doi.org/10.3390/ijms21093087.
- Ren, Y., J. Gong, F. Wang, Z. Li, J. Zhang, R. Fu, and J. Lou. 2016. “Effect of Dye Bath pH on Dyeing and Functional Properties of Wool Fabric Dyed with Tea Extract.” Dyes and Pigments 134:334–341. https://doi.org/10.1016/j.dyepig.2016.07.032.
- Rimkiene, L., A. Kubiliene, A. Zevzikovas, D. Kazlauskiene, and V. Jakstas. 2017. “Variation in Flavonoid Composition and Radical-Scavenging Activity in Ginkgo Biloba L. Due to the Growth Location and Time of Harvest.” Journal of Food Quality 2017:1–8. https://doi.org/10.1155/2017/6840397.
- Santos, E. L., B. H. L. N. S. Maia, A. P. Ferriani, and S. D. Teixeira. 2017. “Flavonoids: Classification, biosynthesis and chemical ecology.” Flavonoids-From Biosynthesis to Human Health 13:78–94. https://doi.org/10.5772/67861.
- Sati, P., P. Dhyani, I. D. Bhatt, and A. Pandey. 2019. “Ginkgo Biloba Flavonoid Glycosides in Antimicrobial Perspective with Reference to Extraction Method.” Journal of Traditional and Complementary Medicine 9 (1): 15–23. https://doi.org/10.1016/j.jtcme.2017.10.003.
- Singh, S. K., S. Srivastav, R. J. Castellani, G. Plascencia-Villa, and G. Perry. 2019. “Neuroprotective and Antioxidant Effect of Ginkgo Biloba Extract Against AD and Other Neurological Disorders.” Neurotherapeutics 16 (3): 666–674. https://doi.org/10.1007/s13311-019-00767-8.
- Smirnoff, N., and Q. J. Cumbes. 1989. “Hydroxyl Radical Scavenging Activity of Compatible Solutes.” Phytochemistry 28 (4): 1057–1060. https://doi.org/10.1016/0031-9422(89)80182-7.
- Wang, C., H. Shi, M. Yang, Y. Yan, E. Liu, Z. Ji, and J. Fan. 2020. “Facile Synthesis of Novel Carbon Quantum Dots from Biomass Waste for Highly Sensitive Detection of Iron Ions.” Materials Research Bulletin 124:110730. https://doi.org/10.1016/j.materresbull.2019.110730.
- Wang, B., W. Yang, J. McKittrick, and M. A. Meyers. 2016. “Keratin: Structure, Mechanical Properties, Occurrence in Biological Organisms, and Efforts at Bioinspiration.” Progress in Materials Science 76:229–318. https://doi.org/10.1016/j.pmatsci.2015.06.001.
- Xu, G., and M. R. Chance. 2005. “Radiolytic Modification and Reactivity of Amino Acid Residues Serving as Structural Probes for Protein Footprinting.” Analytical Chemistry 77 (14): 4549–4555.
- Xu, X., J. Gong, Z. Li, Q. Li, J. Zhang, L. Wang, and J. Huang. 2020. “Mordant Free Dyeing and Functionalization of Wool Fabrics with Biocolorants Derived from Apocynum Venetum L. Bast.” ACS Sustainable Chemistry & Engineering 8 (33): 12686–12695. https://doi.org/10.1021/acssuschemeng.0c04757.
- Yu, J., Z. Pang, J. Zhang, H. Zhou, and Q. Wei. 2018. “Conductivity and Antibacterial Properties of Wool Fabrics Finished by Polyaniline/Chitosan.” Colloids and Surfaces A: Physicochemical and Engineering Aspects 548:117–124. https://doi.org/10.1016/j.colsurfa.2018.03.065.
- Zhang, N., W. Lan, Q. Wang, X. Sun, and J. Xie. 2018. “Antibacterial Mechanism of Ginkgo Biloba Leaf Extract When Applied to Shewanella Putrefaciens and Saprophytic Staphylococcus.” Aquaculture and Fisheries 3 (4): 163–169. https://doi.org/10.1016/j.aaf.2018.05.005.
- Zhang, Y., P. Y. Liu, Q. Kong, S. Xing, X. Liu, and L. Sun. 2017. “The Contents of Terpene Trilactone and Flavonoid in Leaves of Seedlings from Ancient Female Ginkgo Trees in China.” Horticultural Plant Journal 3 (4): 165–171. https://doi.org/10.1016/j.hpj.2017.06.002.
- Zhao, L. J., W. Liu, S. H. Xiong, J. Tang, Z. H. Lou, M. X. Xie, and D. F. Liao. 2018. “Determination of Total Flavonoids Contents and Antioxidant Activity of Ginkgo Biloba Leaf by Near-Infrared Reflectance Method.” International Journal of Analytical Chemistry 2018:1–7. https://doi.org/10.1155/2018/8195784.
- Zhou, G., J. Ma, Y. Tang, X. Wang, J. Zhang, and J. A. Duan. 2018. “Multi-Response Optimization of Ultrasonic Assisted Enzymatic Extraction Followed by Macroporous Resin Purification for Maximal Recovery of Flavonoids and Ginkgolides from Waste Ginkgo Biloba Fallen Leaves.” Molecules 23 (5): 1029–1048. https://doi.org/10.3390/molecules23051029.
- Zhou, Y., and R. C. Tang. 2017. “Natural Flavonoid-Functionalized Silk Fiber Presenting Antibacterial, Antioxidant, and UV Protection Performance.” ACS Sustainable Chemistry & Engineering 5 (11): 10518–10526. https://doi.org/10.1021/acssuschemeng.7b02513.
- Zhou, Y., J. Zhang, R. C. Tang, and J. Zhang. 2015. “Simultaneous Dyeing and Functionalization of Silk with Three Natural Yellow Dyes.” Industrial Crops and Products 64:224–232. https://doi.org/10.1016/j.indcrop.2014.09.041.