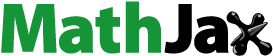
ABSTRACT
A commonly wasted agricultural waste, pomegranate peel has attracted attention as a useful biomaterial with a wide range of potential uses. Fruit and vegetable wastes create environmental difficulties, as well as a loss of valuable biomass and a financial cost to enterprises, due to its high biodegradability. This study focuses on the extraction and characterization of cellulose from both the outer and inner layers of pomegranate peel. The cellulose extraction process involves a multi-step approach, encompassing delignification and mercerization. Fourier transform infrared spectroscopy (FTIR) revealed characteristic peaks associated with cellulose, confirming its presence in the extracted material. Field emission scanning electron microscopy (FESEM) depicted the morphological transformation of cellulose as non-cellulosic components were removed during the extraction process. Particle size analysis demonstrated that alkaline treatment reduced the cellulose particle size. X-ray diffraction (XRD) analysis indicated a significant increase in cellulose crystallinity due to the elimination of hemicellulose and lignin following the extraction process. The chemical composition analysis revealed that treated pomegranate peels contained higher cellulose content compared to their untreated counterparts. This study offers insightful information about the possible uses of pomegranate peel cellulose and its adaptability to a different application.
摘要
石榴皮是一种常见的农业废弃物,作为一种有用的生物材料,具有广泛的潜在用途,引起了人们的关注. 水果和蔬菜废弃物由于其高生物降解性,造成了环境困难,也造成了宝贵生物量的损失和企业的财务成本. 本研究的重点是从石榴皮的外层和内层提取纤维素并对其进行表征. 纤维素提取过程涉及多步骤方法,包括脱木素和丝光. 傅立叶变换红外光谱(FTIR)揭示了与纤维素相关的特征峰,证实了纤维素在提取材料中的存在. 场发射扫描电子显微镜(FESEM)描述了在提取过程中去除非纤维素成分时纤维素的形态转变. 粒度分析表明,碱处理降低了纤维素的粒度. X射线衍射(XRD)分析表明,由于提取过程后半纤维素和木质素的消除,纤维素结晶度显著增加. 化学成分分析显示,与未处理的石榴皮相比,处理过的石榴皮含有更高的纤维素含量. 这项研究提供了关于石榴皮纤维素的可能用途及其对不同应用的适应性的深入信息.
Introduction
Pomegranates are an excellent source of nutrients and antioxidants, and they are readily available after the pomegranate juice and pomegranate arils have been produced (Venkitasamy et al. Citation2019). The purported wound-healing qualities of pomegranate draw attention to it (Bahadoram et al. Citation2022; Kim et al. Citation2021). Pomegranate is an important source of bioactive chemicals and has been utilized in folk medicine for many generations in Greece and Russia (Fakudze et al. Citation2022; Faria and Conceição Citation2011). Pomegranate juice has been shown to have a high level of antioxidant activity and to be useful in preventing atherosclerosis (Balakrishnan et al. Citation2017; Charles et al. Citation2016). According to the ferric reducing antioxidant power (FRAP) assay, pomegranate peel had the highest antioxidant activity of any of the peel, pulp, or seed fractions of any of the 28 commonly eaten fruits in China (Charles, Huang, and Chang Citation2008). The edible portion of the pomegranate fruit is comprised of 40% arils and 10% seeds (Shabtay et al. Citation2008). Bioactive substances such as phenolics and flavonoids, in particular anthocyanins, make up the majority of the arid berries’ rinds, which are primarily composed of water and a small amount of total sugar (Sihag et al. Citation2022). It is estimated that the pomegranate peel accounts for about half of the fruit’s total weight and is a significant source of potassium, calcium, phosphorus, magnesium, and sodium; complex polysaccharides; and a wide range of bioactive compounds, including phenolics, flavonoids, proanthocyanidin compounds, and ellagic acid glycosides (Kumar et al. Citation2022; Siddiqui et al. Citation2019). The peel of a fruit typically constitutes a significant proportion, ranging from 15% to 50% of the overall fruit structure. Once the edible flesh of the fruit has been consumed, the peel is commonly discarded (Görgüç, Gençdağ, and Yılmaz Citation2022). In some circumstances, the amount of waste generated is greater than the amount of product that was produced. In addition, more than a billion tons of fruit and vegetable wastes (FVWs) are produced in India, China, the United States, and the Philippines each year, with the majority and of this trash being disposed of in landfills and rivers, causing environmental pollution (Pathak, Mandavgane, and Kulkarni Citation2015). As an alternative to dumping, it in a landfill, this material might be used to create new products with added value such as reinforcer in polymer composites. Rather than using FPW for a single application, an integrated method that ensures economic feasibility for several applications is needed (Afolalu et al. Citation2021, Citation2022; Paul et al. Citation2022). In the context of global pomegranate production, Cano-Lamadrid et al. (Cano-Lamadrid et al. Citation2022) have noted that the total production of pomegranate worldwide is approximately three million tons. Given that the peel and seeds of the pomegranate represent about 54% of the fruit, this equates to approximately 1.62 million tons of waste. Pomegranate peel waste that is disposed in landfills breaks down anaerobically, releasing methane, a powerful greenhouse gas that drives climate change. Furthermore, poor disposal techniques can pollute water and soil, which affects agricultural lands and ecosystems (Sharma, Singh, and Sharma Citation2023). With the rise of biorefinery concepts, research has shifted to converting biomass into new product as a new approach (Ilyas et al. Citation2019; Ilyas, Sapuan, and Ishak Citation2018, Citation2018; Joglekar et al. Citation2019).
Pomegranate peel (Punica granatum) is gaining attention as an attractive biomaterial derived from a natural renewable resource (Giannelli et al. Citation2021; Xiang et al. Citation2022). With the growing risks associated with global warming, extreme emissions, and resource scarcity, finding alternative materials that reduce the depletion of Earth’s resources and minimize environmental impact is crucial. Pomegranate peel offers several advantages, including its biodegradable nature, abundant availability, low cost, non-toxicity, low density, eco-friendliness, recyclability, and high strength-to-weight ratio (El Barnossi, Moussaid, and Iraqi Housseini Citation2021; Giannelli et al. Citation2021; Xiang et al. Citation2022). Chemically, pomegranate peel is composed of various constituents, including cellulose, hemicellulose, lignin, wax, ash, and other soluble substances (Akhtar et al. Citation2015; Al-Rawahi et al. Citation2013; Zhai et al. Citation2018). The cellulose content in the peel contributes to its strength and stability, making it a suitable candidate for various applications. The crystallinity index is often used to determine the crystalline or amorphous nature of a fiber (Alwash Citation2020; Zeng et al. Citation2022). The physical, chemical, and mechanical characteristics of pomegranate peel fibers can vary based on factors such as plant maturity, growth conditions, and location.
Several recent studies have focused on investigating natural fibers, examining their chemical composition, mechanical, thermal, and morphological characteristics (Ishak et al. Citation2012; Yusriah et al. Citation2014). In a study by Yusriah et al. (Yusriah et al. Citation2014), the impact of betel nut husk (BNH) maturation stages (raw, ripe, matured) on its physical, mechanical, thermal, and morphological properties was discussed. The researchers found that the ripe fiber demonstrated the highest tensile strength among the different maturation stages. Another study examined the chemical composition and tensile properties of sugar palm fibers (Ishak et al. Citation2012). The researchers discovered that the green fibers exhibited the greatest tensile strength, attributed to their optimal chemical composition, including a high cellulose content, as well as hemicellulose and lignin (Kamaruddin et al. Citation2021, Citation2022). However, there is a scarcity of research on the application of Punica granatum fibers and their composites. Extraction and characterization of cellulose from peel waste serve several purposes. First, it addresses the serious environmental threat caused by agricultural waste, particularly fruit peels. It can reduce the environmental impact of this waste by separating valuable components like cellulose from it. Second, in agricultural waste, cellulose is a common component of plant biomass. Its extraction and use can assist in reducing the use of synthetic, non-biodegradable materials. Third, high-quality cellulose produced from waste goods has potential applications in a wide range of industries, including food packaging, textile manufacture, and wastewater treatment. Finally, the cellulose extraction process gives economic value to what would otherwise be deemed waste. This method encourages not only sustainability but also economic development. Thus, this current study aims to investigate the properties of cellulose from the outer and inner layer of pomegranate peel (Punica granatum) from Malaysia contributing to the body of knowledge on waste valorization and providing a basis for potential applications of the extracted cellulose. The cellulose derived from Punica granatum underwent a comprehensive characterization process, encompassing chemical composition, morphology, particle size analysis, crystallinity, and functional group analysis. The findings were presented and compared with those of other natural fibers.
Experimental details
Materials
The pomegranate peel used in this study was collected from the market in Cheras, Taman Connaught, Malaysia. The chemicals used in the cellulose extraction process, including sodium hydroxide (NaOH), sodium chlorite (NaClO2) and acetic acid (CH3COOH) were supplied by Evergreen Engineering & Resources (Malaysia).
Methods
The extraction of cellulose from pomegranate peel is a multi-step process. Cellulose fibers were extracted from pomegranate peel (PP) through two main processes, namely delignification and mercerization. The initial process was performed in accordance with ASTM D1104–56 (1978) (Abraham et al. Citation2013) to prepare holocellulose using the process delignification, designed primarily to remove lignin from pomegranate peel. For the experiment, 200 g of outer (PP1) and inner layer (PP2) of pomegranate peels were first pretreated with water by rinsing with tap water to remove foreign particles and dust. The pomegranate peel is then treated with a delignifying agent, such as sodium hydroxide, to remove the lignin. The clean peels were soaked in 500 mL of a 5% w/v NaOH solution for 2 h at 23 ± 2°C. The cellulose produced was filtered and immersed in 500 mL of distilled water containing approximately 7 mL of acetic acid to neutralize the cellulose. The mixture was stirred for approximately 30 s before it was allowed to settle for 5 min, then rinsed it with water until a pH meter indicated that the cellulose residue was free from acid. The extracted cellulose, referred to as holocellulose, was treated further to produce α-cellulose according to ASTM D1103–60 (1977) (Afolalu et al. Citation2021). The alkaline treatment is the most important step in the extraction of cellulose from pomegranate peel. It is followed by the bleaching step, which can improve the whiteness and clarity of the cellulose product. The bleaching process typically involves the use of a bleaching agent such as sodium chlorite (NaClO2). The holocellulose was soaked in a 1000 mL beaker containing 650 mL of hot distilled water at 95°C. The beaker was then transferred to a water bath set at 70°C. 4 mL of acetic acid and 8 g of sodium chlorite were added to the beaker every hour for seven consecutive hours. The change of color of the pomegranate peels from light brown to white indicated the degree of delignification. The final step involves isolating the holocellulose, which consists of an acid treatment and filtration. A weak acid, such as acetic acid (4 mL) in 500 mL mixture of cellulose, was added to break down the cellulose fibers and make them easier to separate. Finally, it was filtered, washed, and rinsed with distilled water. The final product, denoted as pomegranate peel cellulose 1 (PPC1) and pomegranate peel cellulose 2 (PPC2), was dried in an oven at 50°C overnight. Fourier transform infrared spectroscopy (FTIR) and X-ray diffraction (XRD) analyses were utilized to confirm the purity of the extracted cellulose. Functional groups present in the cellulose were identified by the FTIR, while the crystallinity index of the cellulose was determined by the XRD analysis.
Fourier transform infrared spectroscopy (FTIR)
FTIR spectra (Perkin Elmer, Model: Spectrum 100) were used to identify the functional groups of the various cellulose materials in ATR-FTIR mode. The FTIR spectra were studied by measuring the transmittance percentage relative to the wavenumber, typically carried out at room temperature. With a spectral resolution of 4 cm−1, each specimen was scanned 16 times between 4000 and 525 cm−1. Measurements of the sample’s response to the radiative source yield spectra, which can then be analyzed. In FTIR spectroscopy, the IR radiation that is absorbed by the sample causes the molecules to vibrate due to the energy they absorb. The difference in energy between the resting and excited vibrational states determines which specific wavelengths of light are absorbed by the molecules. The molecular structure dictates which wavelengths are absorbed by a material under investigation.
Field emission scanning electron microscopy (FESEM)
FESEM (FEI Nova NanoSEM 230 FE-SEM, Czech Republic) was utilized to inspect the surface morphology of materials and microstructure at an acceleration voltage of 3 kilovolts (kV). The samples were dried in oven set to 50°C and then affixed to a copper stub with carbon tapes. This process took a total of 20 min. The samples were coated with a thin layer of gold using sputtering equipment to prevent electrostatic charge and poor analytical resolution. These preparations helped to observe the sample’s morphology and have them photographed.
Particle size analysis
Crystalline cellulose’s particle size was measured using a laser diffraction particle size analyzer (Nano-sizer; Model: Nano-S, Brand: Malvern Instruments, Worcestershire, UK). Cellulose’s refractive index was determined using a refractometer. The particle size analysis was conducted under specific analysis conditions as follows: The sample concentration used was 0.1% (w/v), with distilled water serving as the dispersion medium. A scattering angle of 90° was employed at the refractive index of 1.50. Cellulose particles were dispersed in distilled water during the study. It was then necessary to immerse the particle size analyzer’s probe in the suspension to perform the analysis.
X-ray diffraction (XRD)
The crystallinity of pomegranate peels and cellulose isolated from pomegranate peels was determined through X-ray diffraction (XRD) analysis. The diffraction profile was acquired using a Shimadzu XRD 6000 X-ray diffractometer operated at 40 kV and 30 mA and heated at a rate of 2°C per minute. The samples were scanned using a monochromatic Cu-Kα radiation source with a wavelength of 1.5406 Å in step-scan mode. The scan varied the 2θ angle from 5 to 70 degrees. Segal technique was used to calculate the crystallinity index (CI) (Mohammad Rahmani et al. Citation2022; Segal et al. Citation1959; Syafri et al. Citation2022). The Segal CI (%) was calculated by the following equation:
where I002 is the highest 2θ peak intensity of the (002) for cellulose Iβ at 22.7° and of the (0 2 0) peak for cellulose II at 2θ = 21.7°, and Iam is the amorphous intensity at 2θ = 18° for cellulose Iβ and at 2θ = 15° for cellulose II. The highest peak intensity value for crystalline cellulose (I002) was observed at around 2 theta = 21–22 degrees, and the maximum peak intensity value for the amorphous portion of cellulose type I (Iam) was observed at around 2 theta = 15 degrees (Szymańska-Chargot et al. Citation2017).
Chemical composition
Standard procedures were used to determine the chemical composition of the samples based on the TAPPI T222 (2006) (acid insoluble lignin in wood and pulp) and TAPPI T203 (1999) (Afolalu et al. Citation2022) with slight modification by the Institute of Tropical Forestry and Forest Products, Universiti Putra Malaysia (INTROP-UPM) (Ilyas, Sapuan, and Ishak Citation2018). Briefly, the TAPPI T222 procedure was as follows: 40 mL sulfuric acid (72%, H2SO4) was added gradually to the test specimen beakers at 10–15°C while swirling and macerating with a glass rod. Maintain the beaker in a 20 ± 1°C bath for material dispersion. After the dispersion, continue bathing at 20 ± 1°C for 2 h keeping cover the beaker with a watch glass and mixed the material often to ensure thorough solution. 300–400 mL of water was added into a flask and transfer the substance from the beaker. Washed and diluted with water to 3% sulfuric acid concentration. Keep maintain consistent volume by boiling the solution for 4 h using a reflux condenser by letting the insoluble lignin settle in an inverted flask overnight. Then filter the supernatant solution through a crucible without agitating the precipitate and transfer the lignin quantitatively to the filter using hot water. Finally, weighed the lignin after washed in hot water to remove acid and dried in an oven at 105 ± 3°C. The TAPPI 203 procedure was as follows: the sample was placed in a beaker and added 75 mL of 17.5% NaOH reagent at 25 ± 0.2°C maintaining the stir to ensure complete dispersion. After dispersion, 17.5% NaOH reagent was added keeping the suspension at 25 ± 0.2°C for 60 ± 5 min. Finally, the suspension was filtered and collected the filtrate for Alpha-Cellulose determination using titration with 0.5N potassium dichromate solution. For Beta- and Gamma-cellulose determination, the filtrate was heated to coagulate beta-cellulose, then settle and obtain a clear solution.
Results and discussion
Extraction and isolation of cellulose
Outer and inner layer of pomegranate peels (PP1 and PP2) (a), dewaxed and rinsed peels (b), alkaline treated PP1 and PP2 (c), bleached PP1 and PP2 suspension (d and e), extracted cellulose of pomegranate peels (PPC1 and PPC2) (f) and stepwise process are depicted in . The extraction and isolation of cellulose from pomegranate peels (PP1 and PP2) is a multi-step process (Vallejo et al. Citation2021; Zeng et al. Citation2022). The first step is to remove the dust and suspended particles from the pomegranate peels by rinsing them with tap water. The next step is to soak the pomegranate peels in hot distilled water. This process, called mercerization, causes the pomegranate peels to swell and their color to change from brown to pale yellow. This process causes cellulose fibers to swell and become more hydrophilic. This is due to the fact that the alkaline solution disrupts the hydrogen bonds between the cellulose fibers, allowing them to absorb more water. The swelling of the cellulose fibers also makes them more transparent, which is why the pomegranate peels turn pale yellow during this process. The mercerization process also removes pigments and other hydrophilic compounds from the pomegranate peels. The third step is to treat the pomegranate peels with an alkaline solution, such as sodium hydroxide (NaOH). This process, called alkali treatment, causes the pomegranate peels to turn from yellow to brown to pale orange. The alkaline treatment removes hemicellulose and lignin from the pomegranate peels, which makes the cellulose fibers more visible. Hemicellulose is a polysaccharide that is found in the cell walls of plants. It is made up of glucose, xylose, and arabinose. Lignin is a complex polymer that is also found in the cell walls of plants. It is made up of phenylpropanoid units. Both hemicellulose and lignin can interfere with the bonding of cellulose fibers, so their removal makes the cellulose fibers more visible. The fourth step is to bleach the pomegranate peels with sodium chlorite. This process removes any remaining lignin and hemicellulose from the pomegranate peels, making the cellulose fibers even more visible. This is done by using a chemical called sodium chlorite. It oxidizes lignin and hemicellulose, breaking them down into smaller molecules that are soluble in water. The removal of lignin and hemicellulose further increases the visibility of the cellulose fibers. The final step is to isolate the cellulose fibers (PPC1 and PPC2) from the pomegranate peels. This can be done by filtering the solution or by centrifugation (Szymańska-Chargot et al. Citation2017). The final yield of PPCs was about 30−35% after hydrolysis treatment (of initial weight).
Fourier transform infrared spectroscopy (FTIR)
Functional group classification in pomegranate peel cellulose and untreated pomegranate peel is achieved through a targeted FTIR spectrum analysis. Peaks at (1441 cm−1), (1324 cm−1), (1177 cm−1), (1027 cm−1), and (877 cm−1) are corresponding to characteristic peaks of cellulose, as shown in . The cellulose in both pomegranate peels (PP1 and PP2) and pomegranate peel cellulose (PPC1 and PPC2) exhibits a C-H-vibration indication at 877 cm−1 and 892 cm−1, respectively, due to β-glycosidic linkage of cellulose and hemicellulose (Szymańska-Chargot et al. Citation2017). The high intensity of these peaks in PPC1 than PPC2 caused by C-H and C – O-C stretching vibrations in cellulose affirms that the relative content of cellulose grows during the alkali treatment (Shulga et al. Citation2023). The occurrence of peaks at 1033 cm−1 and 1032 cm−1 with significant intensity is attributed to the C-O stretching vibration of the cellulose. A small peak appears in both (PP and PPC) FTIR graphs at 1242 cm−1 attributed to C-O stretching of acetyl group of lignin. Additionally, the peaks in the FTIR spectra of the two samples are located at around 1324 cm−1 for pomegranate peels and for the cellulose of pomegranate peels. Nonetheless, many shoulder peak appears in cellulose (PPC1 and PPC2) from pomegranate peels is greater than that of peels (PP1 and PP2) (Thai et al. Citation2020). At 1441 cm−1, a band showing a peak was observed in both the samples and is the most prominent peak from cellulose and hemicellulose representing -CH2 bending vibration. The high intensity of this peak refers to degree of cellulose crystallinity as shown in in pomegranate peel cellulose FTIR graph (Vallejo et al. Citation2021). Peak at 1612 cm−1 in pomegranate peel diminished in cellulose graph refer to the removal of lignin attribute by C=C, O-H bending from adsorbed water, and C=O stretching from hemicellulose (Ilyas, Sapuan, and Ishak Citation2018). The hydroxyl bond stretch of the O-H group can be attributed to the peak at (3342, 2896) cm−1, while the peak at (3333, 2916) cm−1 is part of the pomegranate peel cellulose spectrum. The increased level of organic extractives such as phenolic acid, methyl ester, and fatty acid methyl ester, which has an intramolecular interaction between the -COOH group, is responsible for the peak at 2843 cm−1 in the spectra of pomegranate peel cellulose (Vârban et al. Citation2021). Peaks appeared at 3285 cm−1 attributed to -O-H stretching vibration in both the peels and cellulose due to the presence of hydroxyl group of cellulose, hemicellulose, waxes, phenolic, and aliphatic hydroxyl group of lignin (Syafri et al. Citation2022).
Field emission scanning electron microscopy (FESEM)
Surface morphologies of cellulose isolated from that peels are shown in . Non-cellulosic components, including sticky polysaccharides of hemicellulose, lignin, pectin, and waxes, cover the surface of pomegranate peel cellulose (PP2), giving it a non-uniform appearance and an irregular shape (Oh et al. Citation2005). shows cellulose as long, thin, fibrous structures in both images. These structures are typical of cellulose nanocrystals, which are often referred to as rod- or needle-like. The surface of these structures seems rough, indicating a high surface area, which is advantageous in many cellulose nanocrystal applications, such as the fabrication of reinforced composites or as drug delivery carriers.
Figure 3. FESEM image of cellulose from (a) outer layer of pomegranate peel (PPC1) and (b) inner layer of pomegranate peel (PPC2).

Delignification of pomegranate peels using acidified sodium chlorite results in a cleaner surface on the cellulose, demonstrating that the lignin and other extractives (waxes, oil, and pectin) have been eliminated (Abraham et al. Citation2013). , shows that after being treated with alkali, the cellulose in pomegranate peel became uniformly microfibrillated, proving that the hemicellulose that was present in the peel had been removed. Morphology of cellulose fiber from different sources is shown in .
Table 1. Morphology and crystallinity index of cellulose fiber from different sources.
Particle size analysis
Cellulose’s particle size distribution was evaluated using data from a particle size analyzer as shown in . Particle size distribution analysis began with measuring the refractive index of cellulose extracted from pomegranate peels (PPC1 and PPC2) with a refractometer. Cellulose extracted from pomegranate peels had the same refractive index of 1.53. These results suggest that the particles in the PPC2 sample are smaller than those in the PPC1 sample. This could be due to differences in the outer and inner layer of peel chemical compositions and an intensive treatment might result in smaller particles. It is also worth noting that the width of the peaks provides information about the size distribution of the particles. A narrower peak suggests a more uniform particle size, while a wider peak suggests a broader size distribution (Harini, Ramya, and Sukumar Citation2018). Alkaline treatment reduced the particle size and distribution of cellulose extracted from pomegranate peels. shows the two peaks that refer to the bimodal distribution that indicate the two different sizes present in the solutions. The presence of two sizes is likely due to aggregation or clumping of particles in water suspension to form larger particles. The average size distribution in PPC1 was 0.053–15.10 nm in size. In contrast, the majority of cellulose particles in PPC2 were 0.53–27.71 nm in size. The particle size distribution narrowed in accordance with this decrease in particle size. The polydispersity index (PDI) of PPC1 was 0.55, while the PDI of PPC2 was 0.75. This indicates that the alkaline treatment produced a more homogeneous distribution of cellulose particles. It follows that the alkaline treatment of cellulose breaks down the intermolecular hydrogen bonds that bind the chains of cellulose together, reducing particle size (Sharma, Sharma, and Srivastava Citation2022). As a result, the cellulose chains are able to unwind and become susceptible to breaking up. Based on these results, alkaline treatment appears to be a viable technique for reducing cellulose that has been isolated from pomegranate peels.
X-ray diffraction (XRD)
XRD analysis was used to assess the crystallinity index as well as changes in crystallinity and amorphous region of pomegranate peels (PP1 and PP2), and pomegranate peel cellulose (PPC1 and PPC2) as shown in .
Figure 5. X-ray diffraction curve for pomegranate peels (PP1 and PP2) and pomegranate peel cellulose (PPC1 and PPC2).

The strong and sharp peak intensity of cellulose generated from PPC was confirmed by a 2θ value of 22° which is a common value of cellulose type I. The amorphous phase of cellulose type I, on the other hand, was characterized by a low diffracted intensity of roughly 18° at a 2θ value. The amorphous region in the XRD spectrum of PP is identified by the presence of a broad peak around 21° at 2θ value. The XRD graph shows that pomegranate peels have a low crystallinity index (28.80% for PP1 and 28.59% for PP2) due to the presence of a large amount of amorphous hemicellulose and lignin (Harini and Mohan Citation2020; Syafri et al. Citation2022). The alkaline and bleaching processes remove the hemicellulose and lignin, leading to an increase in the crystallinity index of the cellulose to 78.16% for PPC1 and 67.96% for PPC2. These results are comparable with earlier results as shown in . The crystalline region in the XRD spectrum of PPC is identified by the presence of several sharp peaks. The increase in the crystallinity of the cellulose may be attributed to an increase in the intra and intermolecular hydrogen bonding after the alkaline and bleaching processes (Tang et al. Citation2013). It could have several beneficial effects on its properties, such as increased strength, stiffness, and durability for better reinforcement agent in preparation of composite materials.
Chemical composition
From , it was seen that PPC1 had the highest cellulose contents, and it was 71.35% followed by PPC2 with 67.52%. The cellulose content for PP1 and PP2 was 58.75%. In addition, more cellulose was found in the pomegranate peels that had been treated (PPC) than in the raw pomegranate peels (PP). Some extractive components of raw pomegranate peels were likely removed during the delignification and mercerization processes, which then separated a greater proportion of the insoluble chemicals in the treated peels, explaining the observed result (Mazlita, Lee, and Hamid Citation2016; Ouajai and Shanks Citation2005).
Table 2. Chemical composition analysis of pomegranate peel components.
Conclusions
The present study focused on the extraction and characterization of cellulose derived from the outer and inner layer of pomegranate peel. The primary objective was to highlight the importance of waste valorization in this context. The utilization of FTIR analysis allowed for the identification of distinctive cellulose peaks in the cellulose and pomegranate peel. Furthermore, the FESEM study provided visual evidence indicating that the rod-like shape depicts the successful elimination of non-cellulosic constituents during the extraction procedure. The results of the particle size analysis indicate the possibility of manipulating the size of the particles (PPC1), while the XRD analysis revealed an observed increase in the crystallinity of cellulose (PPC1>PPC2). The chemical composition analysis conducted on the treated pomegranate peels revealed a notable increase (71%) in the cellulose content compared to the untreated samples (60%). The results of this study demonstrate the considerable potential exhibited by pomegranate peel cellulose in a wide range of applications that include but are not limited to composite materials, food packaging, textiles, and wastewater treatment. These applications hold promise in terms of waste reduction and economic advancement. The research highlights the significance of implementing sustainable practices and harnessing the potential of easily accessible agricultural waste for the development of value-added products.
Highlights
Pomegranate peels turned into microsized cellulose with high crystallinity through alkaline and bleaching treatments.
Fruit and vegetable waste creates environmental problems and financial costs but can be used as valuable biomass.
FTIR, FESEM, PSA, and XRD techniques used to characterize pomegranate peel cellulose obtained from a waste product.
Author’s contribution
Vasi Uddin Siddiqui: Conceptualization, Methodology, Software, Formal analysis, Validation, Writing – Reviewing and Editing. Tareq Mohammed Almehjani: Writing – Original draft preparation, Investigation. S.M. Sapuan: Supervision, Conceptualization, Project administration, Funding acquisition. Tarique Jamal: Formal analysis, Writing – Reviewing and Editing. R.A. Ilyas: Funding acquisition, Writing – Reviewing and Editing. Sayed M Eldin: Funding acquisition, Writing – Reviewing and Editing. Abir Khan: Writing – Reviewing and Editing. Yusuf Jameel: Writing – Reviewing and Editing.
Acknowledgments
Authors would like to acknowledge Universiti Putra Malaysia (UPM) for providing research facilities. This work was financially supported by Universiti Putra Malaysia through Geran Putra Inisiatif (No. GPI/2022/9720100).
Disclosure statement
No potential conflict of interest was reported by the author(s).
Data availability statement
Data sharing is not applicable to this article as no new data were created or analyzed in this study.
Additional information
Funding
References
- Abraham, E., B. Deepa, L. A. Pothen, J. Cintil, S. Thomas, M. J. John, R. Anandjiwala, and S. S. Narine. 2013. “Environmental Friendly Method for the Extraction of Coir Fibre and Isolation of Nanofibre.” Carbohydrate Polymers 92 (2): 1477–14. https://doi.org/10.1016/j.carbpol.2012.10.056.
- Afolalu, S. A., O. Okwilagwe, O. O. Yusuf, O. R. Oloyede, S. O. Banjo, and F. Ademuyiwa. 2022. “Overview of Nano-Agro-Composite Additives for Wastewater and Effluent Treatment.” Green Energy and Technology 223–236. https://doi.org/10.1007/978-3-030-95820-6_19.
- Afolalu, S. A., E. Y. Salawu, T. S. Ogedengbe, O. O. Joseph, O. Okwilagwe, M. E. Emetere, O. O. Yusuf, A. Noiki, and S. Akinlabi. 2021. “Bio-Agro Waste Valorization and Its Sustainability in the Industry: A Review.” IOP Conference Series: Materials Science & Engineering 1107 (1): 012140. https://doi.org/10.1088/1757-899X/1107/1/012140.
- Akhtar, S., T. Ismail, D. Fraternale, and P. Sestili. 2015. “Pomegranate Peel and Peel Extracts: Chemistry and Food Features.” Food Chemistry 174:417–425. https://doi.org/10.1016/j.foodchem.2014.11.035.
- Al-Rawahi, A. S., M. S. Rahman, N. Guizani, and M. M. Essa. 2013. “Chemical Composition, Water Sorption Isotherm, and Phenolic Contents in Fresh and Dried Pomegranate Peels.” Drying Technology 31 (3): 257–263. https://doi.org/10.1080/07373937.2012.710695.
- Alwash, A. 2020. “The Green Synthesize of Zinc Oxide Catalyst Using Pomegranate Peels Extract for the Photocatalytic Degradation of Methylene Blue Dye.” Baghdad Science Journal 17 (3): 0787. https://doi.org/10.21123/bsj.2020.17.3.0787.
- Bahadoram, M., S. Hassanzadeh, S. Bahadoram, and K. Mowla. 2022. “Effects of Pomegranate on Wound Repair and Regeneration.” World Journal of Plastic Surgery 11 (1): 157–159. https://doi.org/10.52547/wjps.11.1.157.
- Balakrishnan, P., M. S. Sreekala, M. Kunaver, M. Huskić, and S. Thomas. 2017. “Morphology, Transport Characteristics and Viscoelastic Polymer Chain Confinement in Nanocomposites Based on Thermoplastic Potato Starch and Cellulose Nanofibers from Pineapple Leaf.” Carbohydrate Polymers 169:176–188. https://doi.org/10.1016/j.carbpol.2017.04.017.
- Cano-Lamadrid, M., L. Martínez-Zamora, N. Castillejo, and F. Artés-Hernández. 2022. “From Pomegranate Byproducts Waste to Worth: A Review of Extraction Techniques and Potential Applications for Their Revalorization.” Foods 11 (17): 2596. https://doi.org/10.3390/foods11172596.
- Charles, A. L., K. Cato, T.-C. Huang, Y.-H. Chang, J.-Y. Ciou, J.-S. Chang, and H.-H. Lin. 2016. “Functional Properties of Arrowroot Starch in Cassava and Sweet Potato Composite Starches.” Food Hydrocolloids 53:187–191. https://doi.org/10.1016/j.foodhyd.2015.01.024.
- Charles, A. L., T. C. Huang, and Y. H. Chang. 2008. “Structural Analysis and Characterization of a Mucopolysaccharide Isolated from Roots of Cassava (Manihot Esculenta Crantz L.).” Food Hydrocolloids 22 (1): 184–191. https://doi.org/10.1016/j.foodhyd.2006.10.012.
- de Oliveira JP, G. P. Bruni, K. O. Lima, S. L. El Halal, da Rosa GS, A. R. Dias, and E. da Rosa Zavareze. 2017. “Cellulose Fibers Extracted from Rice and Oat Husks and Their Application in Hydrogel.” Food Chemistry 221:153–160. https://doi.org/10.1016/j.foodchem.2016.10.048.
- Djafari Petroudy, S. R., S. Arjmand Kahagh, and E. Vatankhah. 2021. “Environmentally Friendly Superabsorbent Fibers Based on Electrospun Cellulose Nanofibers Extracted from Wheat Straw.” Carbohydrate Polymers 251: 117087. https://doi.org/10.1016/j.carbpol.2020.117087.
- Ejaz, U., S. Muhammad, F. I. Ali, I. A. Hashmi, and M. Sohail. 2020. “Cellulose Extraction from Methyltrioctylammonium Chloride Pretreated Sugarcane Bagasse and Its Application.” International Journal of Biological Macromolecules 165: 11–17. https://doi.org/10.1016/j.ijbiomac.2020.09.151.
- El Barnossi, A., F. Moussaid, and A. I. Housseini. 2021. “Tangerine, Banana and Pomegranate Peels Valorisation for Sustainable Environment: A Review.” Biotechnology Reports 29:e00574. https://doi.org/10.1016/j.btre.2020.e00574.
- Fakudze, N. T., E. C. Aniogo, B. P. George, and H. Abrahamse. 2022. “The Therapeutic Efficacy of Punica Granatum and Its Bioactive Constituents with Special Reference to Photodynamic Therapy.” Plants 11 (21): 2820. https://doi.org/10.3390/plants11212820.
- Faria, A., and C. Conceição. 2011. “The Bioactivity of Pomegranate: Impact on Health and Disease.” Critical Reviews in Food Science and Nutrition 51 (7): 626–634. https://doi.org/10.1080/10408391003748100.
- Giannelli, M., V. Lacivita, T. Posati, A. Aluigi, A. Conte, R. Zamboni, and M. A. Del Nobile. 2021. “Silk Fibroin and Pomegranate By-Products to Develop Sustainable Active Pad for Food Packaging Applications.” Foods 10 (12): 2921. https://doi.org/10.3390/foods10122921.
- Görgüç, A., E. Gençdağ, and F. M. Yılmaz. 2022. “Industrial Pomegranate Wastes and Their Functional Benefits in Novel Food Formulations.” In Mediterranean Fruits Bio-Wastes, 721–738. Springer International Publishing. https://doi.org/10.1007/978-3-030-84436-3_31.
- Harini, K., and C. C. Mohan. 2020. “Isolation and Characterization of Micro and Nanocrystalline Cellulose Fibers from the Walnut Shell, Corncob and Sugarcane Bagasse.” International Journal of Biological Macromolecules 163:1375–1383. https://doi.org/10.1016/j.ijbiomac.2020.07.239.
- Harini, K., K. Ramya, and M. Sukumar. 2018. “Extraction of Nano Cellulose Fibers from the Banana Peel and Bract for Production of Acetyl and Lauroyl Cellulose.” Carbohydrate Polymers 201 (June): 329–339. https://doi.org/10.1016/j.carbpol.2018.08.081.
- Ilyas, R. A., S. M. Sapuan, and M. R. Ishak. 2018. “Isolation and Characterization of Nanocrystalline Cellulose from Sugar Palm Fibres (Arenga Pinnata).” Carbohydrate Polymers 181 (October 2017): 1038–1051. https://doi.org/10.1016/j.carbpol.2017.11.045.
- Ilyas, R. A., S. M. Sapuan, M. R. Ishak, and E. S. Zainudin. 2018. “Development and Characterization of Sugar Palm Nanocrystalline Cellulose Reinforced Sugar Palm Starch Bionanocomposites.” Carbohydrate Polymers 202 (September): 186–202. https://doi.org/10.1016/j.carbpol.2018.09.002.
- Ilyas, R. A., S. M. Sapuan, M. R. Ishak, and E. S. Zainudin. 2019. “Sugar Palm Nanofibrillated Cellulose (Arenga Pinnata (Wurmb.) Merr): Effect of Cycles on Their Yield, Physic-Chemical, Morphological and Thermal Behavior.” International Journal of Biological Macromolecules 123:379–388. https://doi.org/10.1016/j.ijbiomac.2018.11.124.
- Ishak, M. R., S. M. Sapuan, Z. Leman, M. Z. A. Rahman, and U. M. K. Anwar. 2012. “Characterization of Sugar Palm (Arenga Pinnata) Fibres Tensile and Thermal Properties.” Journal of Thermal Analysis and Calorimetry 109 (2): 981–989. https://doi.org/10.1007/s10973-011-1785-1.
- Joglekar, S. N., P. D. Pathak, S. A. Mandavgane, and B. D. Kulkarni. 2019. “Process of Fruit Peel Waste Biorefinery: A Case Study of Citrus Waste Biorefinery, Its Environmental Impacts and Recommendations.” Environmental Science and Pollution Research 26 (34): 34713–34722. https://doi.org/10.1007/s11356-019-04196-0.
- Kamaruddin, Z. H., R. Jumaidin, A. I. Rushdan, M. Z. Selamat, and R. H. Alamjuri. 2021. “Characterization of Natural Cellulosic Fiber Isolated from Malaysian Cymbopogan Citratus Leaves.” BioResources 16 (4): 7729–7750. https://doi.org/10.15376/biores.16.4.7729-7750.
- Kamaruddin, Z. H., R. Jumaidin, M. Z. Selamat, and R. A. Ilyas. 2022. “Characteristics and Properties of Lemongrass (Cymbopogan Citratus): A Comprehensive Review.” Journal of Natural Fibers 19 (14): 8101–8118. https://doi.org/10.1080/15440478.2021.1958439.
- Kim, H. J., J. H. Jeong, Y. H. Choi, and Y. Eom. 2021. “Review on Cellulose Nanocrystal-Reinforced Polymer Nanocomposites: Processing, Properties, and Rheology.” Korea-Australia Rheology Journal 33 (3): 165–185. https://doi.org/10.1007/s13367-021-0015-z.
- Kumar, C. T. M., S. Mondal, W. G. Prasad, G. S. Rathod, H. V. Raghu, and A. Kokkiligadda. 2022. “Evaluation of Physicochemical and Functional Attributes of Whey Powder Incorporated with Pomegranate Peel Extract.” Food Chemistry Advances 1 (August): 100088. https://doi.org/10.1016/j.focha.2022.100088.
- Mazlita, Y., H. V. Lee, and S. B. A. Hamid. 2016. “Preparation of Cellulose Nanocrystals Bio-Polymer from Agro-Industrial Wastes: Separation and Characterization.” Polymers and Polymer Composites 24 (9): 719–728. https://doi.org/10.1177/096739111602400907.
- Oh, S. Y., D. I. Yoo, Y. Shin, and G. Seo. 2005. “FTIR analysis of cellulose treated with sodium hydroxide and carbon dioxide.” Carbohydrate Research 340 (3): 417–428. https://doi.org/10.1016/j.carres.2004.11.027.
- Ouajai, S., and R. A. Shanks. 2005. “Composition, Structure and Thermal Degradation of Hemp Cellulose After Chemical Treatments.” Polymer Degradation and Stability 89 (2): 327–335. https://doi.org/10.1016/j.polymdegradstab.2005.01.016.
- Pathak, P. D., S. A. Mandavgane, and B. D. Kulkarni. 2015. “Fruit peel waste as a novel low-cost bio adsorbent.” Reviews in Chemical Engineering 31 (4): 361–381. https://doi.org/10.1515/revce-2014-0041.
- Paul, V., A. D. Tripathi, S. Yamini, V. K. Paswan, T. Patil, T. Verma, and K. Darani. 2022. “Biocomposites from Fruit and Vegetable Wastes and Their Applications.” In Fruits and Vegetable Wastes, 385–406. Springer Nature Singapore. https://doi.org/10.1007/978-981-16-9527-8_16.
- Rahmani, A. M., P. Gahlot, K. Moustakas, A. A. Kazmi, C. S. P. Ojha, and V. K. Tyagi. 2022. “Pretreatment Methods to Enhance Solubilization and Anaerobic Biodegradability of Lignocellulosic Biomass (Wheat Straw): Progress and Challenges.” Fuel 319 (November 2021): 123726. https://doi.org/10.1016/j.fuel.2022.123726.
- Segal, L., J. J. Creely, A. E. Martin, and C. M. Conrad. 1959. “An Empirical Method for Estimating the Degree of Crystallinity of Native Cellulose Using the X-Ray Diffractometer.” Textile Research Journal 29 (10): 786–794. https://doi.org/10.1177/004051755902901003.
- Shabtay, A., H. Eitam, Y. Tadmor, A. Orlov, A. Meir, P. Weinberg, Z. G. Weinberg, et al. 2008. “Nutritive and Antioxidative Potential of Fresh and Stored Pomegranate Industrial Byproduct as a Novel Beef Cattle Feed.” Journal of Agricultural and Food Chemistry 56 (21): 10063–10070. https://doi.org/10.1021/jf8016095.
- Sharma, C., S. N. Sharma, and R. Srivastava. 2022. “Analysis of Cellulose Extracted from Waste Products.” Colloid and Polymer Science 300 (9): 1027–1036. https://doi.org/10.1007/s00396-022-05005-w.
- Sharma, M., M. Singh, and R. Sharma. 2023. “Transforming Pomegranate Waste into Value-Added Products: An Innovative Approach to Sustainability.” Journal of Survey in Fisheries Sciences 10 (3): 120–127. https://doi.org/10.53555//sfs.v10i1.1715.
- Shulga, G., J. Rizhikovs, B. Neiberte, A. Verovkins, S. Vitolina, T. Betkers, and R. Makars. 2023. “Processing and Properties of Wood-plastic Composite Containing Alkali-Treated Birch Wood Shavings and Bioadditive Obtained by Biorefinery of Birch Bark.” Forests 14 (9): 1906. https://doi.org/10.3390/f14091906.
- Siddiqui, V. U., A. Ansari, R. Chauhan, and W. A. Siddiqi. 2019. “Green Synthesis of Copper Oxide (CuO) Nanoparticles by Punica Granatum Peel Extract.” Materials Today: Proceedings 36:751–755. https://doi.org/10.1016/j.matpr.2020.05.504.
- Sihag, S., A. Pal, Ravikant, and V. Saharan. 2022. “Antioxidant Properties and Free Radicals Scavenging Activities of Pomegranate (Punica Granatum L.) Peels: An in-Vitro Study.” Biocatalysis and Agricultural Biotechnology 42 (September 2021): 102368. https://doi.org/10.1016/j.bcab.2022.102368.
- Syafri, E., Jamaluddin, N. H. Sari, M. Mahardika, P. Amanda, and R. A. Ilyas. 2022. “Isolation and Characterization of Cellulose Nanofibers from Agave Gigantea by Chemical-Mechanical Treatment.” International Journal of Biological Macromolecules 200 (September 2021): 25–33. https://doi.org/10.1016/j.ijbiomac.2021.12.111.
- Szymańska-Chargot, M., M. Chylińska, K. Gdula, A. Kozioł, and A. Zdunek. 2017. “Isolation and Characterization of Cellulose from Different Fruit and Vegetable Pomaces.” Polymers 9 (12): 495. https://doi.org/10.3390/polym9100495.
- Tang, L., B. Huang, N. Yang, T. Li, Q. Lu, W. Lin, and X. Chen. 2013. “Organic Solvent-Free and Efficient Manufacture of Functionalized Cellulose Nanocrystals via One-Pot Tandem Reactions.” Green Chemistry 15 (9): 2369. https://doi.org/10.1039/c3gc40965a.
- Thai, Q. B., S. T. Nguyen, D. K. Ho, T. Du Tran, D. M. Huynh, N. H. N. Do, T. P. Luu, et al. 2020. “Cellulose-Based Aerogels from Sugarcane Bagasse for Oil Spill-Cleaning and Heat Insulation Applications.” Carbohydrate Polymers 228 (September 2019): 115365. https://doi.org/10.1016/j.carbpol.2019.115365.
- Vallejo, M., R. Cordeiro, P. A. N. Dias, C. Moura, M. Henriques, I. J. Seabra, C. M. Malça, and P. Morouço. 2021. “Recovery and Evaluation of Cellulose from Agroindustrial Residues of Corn, Grape, Pomegranate, Strawberry-Tree Fruit and Fava.” Bioresources and Bioprocessing 8 (1). https://doi.org/10.1186/s40643-021-00377-3.
- Vârban, R., I. Crișan, D. Vârban, A. Ona, L. Olar, A. Stoie, and R. Ștefan. 2021. “Comparative FT-IR Prospecting for Cellulose in Stems of Some Fiber Plants: Flax, Velvet Leaf, Hemp and Jute.” Applied Sciences 11 (18): 8570. https://doi.org/10.3390/app11188570.
- Venkitasamy, C., L. Zhao, R. Zhang, and Z. Pan. 2019. “Pomegranate.” In Integrated Processing Technologies for Food and Agricultural By-Products, 181–216. Elsevier. https://doi.org/10.1016/B978-0-12-814138-0.00008-3.
- Xiang, Q., M. Li, J. Wen, F. Ren, Z. Yang, X. Jiang, and Y. Chen. 2022. “The Bioactivity and Applications of Pomegranate Peel Extract: A Review.” Journal of Food Biochemistry 46 (7). https://doi.org/10.1111/jfbc.14105.
- Yang, X., F. Han, C. Xu, S. Jiang, L. Huang, L. Liu, and Z. Xia. 2017. “Effects of Preparation Methods on the Morphology and Properties of Nanocellulose (NC) Extracted from Corn Husk.” Industrial Crops and Products 109:241–247. https://doi.org/10.1016/j.indcrop.2017.08.032.
- Yusriah, L., S. M. Sapuan, E. S. Zainudin, and M. Mariatti. 2014. “Characterization of Physical, Mechanical, Thermal and Morphological Properties of Agro-Waste Betel Nut (Areca Catechu) Husk Fibre.” Journal of Cleaner Production 72:174–180. https://doi.org/10.1016/j.jclepro.2014.02.025.
- Zeng, J., X. Ren, S. Zhu, and Y. Gao. 2022. “Cellulose Nanocrystals from Pomegranate Peel: Isolation, Characterization, and Its Reinforcement for Chitosan Film.” Journal of Materials Science 57 (24): 11062–11076. https://doi.org/10.1007/s10853-022-07255-z.
- Zhai, X., C. Zhu, Y. Li, Y. Zhang, Z. Duan, and X. Yang. 2018. “Optimization for Pectinase-Assisted Extraction of Polysaccharides from Pomegranate Peel with Chemical Composition and Antioxidant Activity.” International Journal of Biological Macromolecules 109:244–253. https://doi.org/10.1016/j.ijbiomac.2017.12.064.
- Zianor Azrina, Z., M. D. Beg, M. Y. Rosli, R. Ramli, N. Junadi, and A. M. Alam. 2017. “Spherical Nanocrystalline Cellulose (NCC) from Oil Palm Empty Fruit Bunch Pulp via Ultrasound Assisted Hydrolysis.” Carbohydrate Polymers 162:115–120. https://doi.org/10.1016/j.carbpol.2017.01.035.