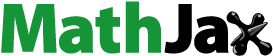
ABSTRACT
The aim of the research was to identify differences in the content of phenolic acids in flax fibers and antioxidant activity of the fibers depending on the soil moisture level during plant growth and flax variety. The experiment involved cultivating flax under controlled conditions with constant levels of irrigation: 25%, 35%, and 45% of field water capacity (FWC). The experiment conducted in the vegetative hall of the Institute of Natural Fibers and Medicinal Plants, National Research Institute in Poland was repeated three times: in 2019, 2020, and 2021. The fibers were extracted from the straw with use of dew retting method. Flax fibers exhibit biological activity. In current study, the impact of drought during flax growth on the antioxidant activity of fibers was determined, as bioactive fibers of linen clothing touching the skin can contribute to neutralizing free radicals formed next to human body. The antioxidant activity of tested fibers was examined using DPPH and FRAP methods. The quantity of phenolic acids may affect the antioxidant capacity of the fibers. The research results showed that the cultivation of flax plants at a soil moisture level of 45% FWC favors the development of antioxidant activity in the fibers.
摘要
本研究的目的是确定亚麻纤维中酚酸含量和纤维抗氧化活性的差异,这取决于植物生长过程中的土壤湿度水平和亚麻品种. 试验包括在控制条件下种植亚麻,灌溉水平不变: 分别为田间持水量的25%、35%和45%. 该实验在波兰国家研究所天然纤维和药用植物研究所的植物大厅进行了三次重复: 2019年、2020年和2021年. 采用露水脱胶法从稻草中提取纤维. 亚麻纤维具有生物活性. 在目前的研究中,确定了亚麻生长过程中干旱对纤维抗氧化活性的影响,因为亚麻服装中接触皮肤的生物活性纤维有助于中和人体附近形成的自由基. 使用DPPH和FRAP方法检测测试纤维的抗氧化活性. 酚酸的含量可能会影响纤维的抗氧化能力. 研究结果表明,在45%FWC的土壤水分水平下培养亚麻植物有利于纤维抗氧化活性的发展.
Introduction
The increasing average air temperature worldwide in recent decades is a consequence of climate change, the effects of which impact various areas of human life and the natural environment. Droughts and the growing popularity of products made from flax fibers have inspired an examination of the influence of weather conditions during flax (Linum usitatissimum L.) cultivation on the quality of fibers that can be used in the textile industry. Flax is the third largest natural source of fibers and one of the five major oilseed crops in the world (Mishra and Awasthi Citation2021). Due to their positive environmental characteristics and properties suitable for textile purpose, flax fibers can be an alternative to synthetic fibers that generate non-biodegradable waste with limited recycling capabilities. The increasing societal awareness of the need to care for the environment, as well as the desire for comfort provided by clothing, have led to the resurgence of popularity of linen garments. Given its numerous advantages, flax deserves attention as a textile material. The health-promoting properties of flax fibers manifest in their antibacterial, antioxidant and phenolic acid content.
Phenolic acids, depending on their structure and chemical properties, can be absorbed to varying degrees by the skin. Their hydrophilicity and lipophilic properties make that acids penetration through the lipid layer of the skin is enable. The quantity and dynamics of substance absorption through the skin depend on biological factors (age, skin condition, cellular metabolism) and physicochemical factors (partition coefficient between the stratum corneum and the carrier, size, spatial structure, and polarity of molecules). Phenolic compounds, upon penetrating the epidermis, can undergo enzymatic or non-enzymatic reactions. The penetration of active molecules can occur through the epidermis, cells, intercellular spaces, vesicles, or through sweat glands and hair follicles (Dzialo et al. Citation2016). Zimniewska demonstrated a strong correlation between the content of phenolic acids in flax fibers and their antioxidant activity, suggesting their positive impact on human skin during use (Zimniewska Citation2015).
Antioxidant activity is the ability to neutralize the harmful effects of free radicals, which are formed in the body as a result of the reduction of oxygen molecules to water molecules. These molecules have the ability to exist independently and their high reactivity and chemical reactions with cells are metabolically unfavorable (Czajka Citation2006). Bast fibers, depending on the plant variety, fibers extraction method and subsequent stages of initial raw material processing, exhibit varying levels of antioxidant activity. It has been proven that bast fibers obtained through decortication show higher antioxidant activity compared to retted fibers (Zimniewska et al. Citation2018). The natural defense mechanism of the human body regularly combats free radicals, but external factors such as UV radiation, environmental pollution, smoking cigarettes and consumption of unhealthy food or alcohol can exacerbate their accumulation. An excessive amount of free radicals leads to oxidative stress, causing adverse changes in the structure of nucleic acids, proteins, and lipids, resulting in cell damage and accelerated aging. Furthermore, oxidative stress contributes to the development of civilization-related diseases, including cardiovascular diseases, cancers, diabetes, osteoporosis, neurodegenerative disorders, and others (Muzykiewicz et al. Citation2019).
In the studies, it was demonstrated that controlled drought stress at 25% field water capacity (FWC) reduces flax yields by 39.7–49.3% depending on the variety, compared to plants grown under optimal conditions (Heller and Byczynska Citation2015). There is also available literature on how drought affects the quality of flax fibers, including length, linear mass, tensile strength, longitudinal and cross-sectional appearances (Kwiatkowska et al. Citation2023). However, there is a lack of sources regarding the impact of water deficit during flax vegetative growth on the content of phenolic acids in fibers and their antioxidant activity.
Materials and methods
The schedule of the experiments
The research focused on three varieties of flax (Linum usitatissimum L.): Artemida, Modran, and Sara. Theplants of these varieties were cultivated under controlled conditions providing soil moisture levels at 25%, 35%, and 45% of the field water capacity of the soil. The climatic conditions and soil composition were the same for all plants in a given year. The experiment was conducted over three consecutive years, namely 2019, 2020, and 2021.
The experiment took place at the vegetative hall of the Institute of Natural Fibers and Medicinal Plants National Research Institute in Petkowo (52°12′40″N, 17°15′31″E). Metal pots with a diameter of 30 cm were used, with 12 replicates for each flax variety tested. The pots were weighed and filled with soil taken from the field, ensuring the appropriate crop rotation for flax cultivation. The growth and development of flax were assessed using the BBCH scale (Biologische Bundesanstalt, Bundessortenamt und Chemische Industrie), which is a standardized system for evaluating the state and stage of growth of crop plants. It allows farmers to apply treatments at optimal agricultural periods. For each pot, 50 flax seeds were sown at a depth of 1 cm. After sowing, a layer of quartz sand (300 g with a thickness of 0.5 cm) was applied to the soil surface of each pot to protect against excessive drying and soil crusting. From the time of sowing until the beginning of the rapid growth phase, when the flax plants reached a height of 18–20 cm (according to BBCH 32), the soil moisture in the pots was maintained at the optimal level of 45% field water capacity. Field water capacity refers to the maximum amount of water that the soil can hold after saturation and excess water drainage.
Once the flax plants had reached a uniform and balanced level of growth, particularly during the germination and early growth stage when they reached a height of 5–6 cm (BBCH 11), a thinning process was conducted in the pots. Approximately 30–32 healthy and evenly grown plants were left in each pot. When the plants reached a height of 18–20 cm (BBCH 32), which marked the initial stage of rapid growth, controlled soil water deficits were introduced at two levels: 25% FWC in four pots and 35% FWC in other four pots with flax for every variety. The remaining four pots were maintained at the control level of 45% FWC for every variety. The symbols of the tested flax varieties grown under specific soil moisture conditions can be found in . Water supplementation was carried out using a weighing method to determine soil moisture and based on field water capacity with use of the Wanschaty method. The experiment was conducted without use of pesticides during flax growing period. The plants were harvested at the green-yellow stage of flax maturity (BBCH 83). The natural dew retting process of the flax straw in the field took 27 days in 2019, 21 days in 2020 and 26 days in 2021, following the necessary steps performed in this type of retting, as advised by an experienced expert. The retting process was completed when the bark in the stem was easily broken and chipped, and the fiber separated from it after breaking. The length of the retting period varied due to different weather conditions during the years of the experiment. The retting method was aimed at ensuring high bioactivity of the fibers. Well retted straw was processed with use of lab breaking machine and hand scutching process. Obtained flax fibers were objective of the further study covering determination of lignin and phenolic acids content in the fibers as well as evaluation of their antioxidant activity depending on soil moisture content to reach this study goal.
Table 1. List of symbols of tested flax varieties grown under specific soil moisture conditions.
Determination of phenolic acids
The method of separating bioactive compounds in flax fibers was conducted based on the studies (Gryszczynska et al. Citation2015; Liu et al. Citation2006). Sample preparation involved placing approximately 0.6 g of flax fiber in a conical flask, followed by adding 15 ml of 70% ethanol. The sample was extracted in an ultrasonic bath for 30 minutes, cooled and filtered. The fibers were processed twice using the same procedure. The supernatants were combined and evaporated to dryness using a rotary evaporator under vacuum. The residue was dissolved in 2 ml of 70% ethanol and the solution was transferred to a 2 ml volumetric flask. Analytical standards such as p-coumaric acid, ferulic acid were purchased from ChromaDex (Irvine, CA, USA) and syringic acid and sinapic acid from Sigma Aldrich.
High-performance liquid chromatography (HPLC) with a diode array detector (DAD) was used for the determination of phenolic acids (1100 system, Agilent). The separation and identification of phenolic acids in the samples were performed using chromatographic analysis with the Zorbax Poroshell 120 SB-C18 column (2.7 mm × 3.0 mm × 100 mm, Agilent). The mobile phase consisted of a mixture of two solutions: solution A containing 0.1% phosphoric acid, and solution B consisting of acetonitrile. Gradient elution was employed for separation. The flow rate was set at 0.8 ml/min, starting with 90% solution A (13 min − 78% solution A, 14 min − 60% solution A, 30 min − 60% solution A). The column temperature was maintained at 40°C, and the injection volume was 100 µl. Peaks were identified by comparing retention times and UV-VIS spectra with those of standard solutions. Quantitative determination of these compounds was achieved using calibration curves prepared with pure standards. Detection of substances was carried out at 205 nm, 303 nm, and 330 nm.
Determination of antioxidant activity
The FRAP (Ferric Reducing Antioxidant Power) and DPPH (2,2-Diphenyl-1-picrylhydrazyl) methods allow for the measurement of different antioxidant properties of substances. Moreover, they have different ranges of sensitivity, meaning that one method may detect antioxidant activity that is not detected by the another method.
The sample preparation involved placing approximately 1 g of flax fibers in a round-bottom flask along with 25 ml of 40% ethanol. The mixture was then heated under a reflux condenser for one hour. After cooling, the solution was filtered into a 25 ml volumetric flask. The sample was then supplemented with 40% ethanol to a volume of 25 ml.
TPTZ (2,4,6-tris(2-pirydylo)-1,3,5-triazyna) and DPPH (2,2-difenylo-1-pikrylohydrazyl) were provided by Sigma Aldrich (Darmstadt, Germany). Sodium acetate, acetic acid, chlorous acid, ferric chloride, and phosphoric acid were purchased from Avantor Performance Materials Poland S.A. (Gliwice, Poland). Acetonitrile was supplied by J.T. Baker (Phillipsburg, NJ, USA).
Determination of the ferric reducing antioxidant power (FRAP)
The method for determining the Ferric Reducing Antioxidant Power (FRAP) based on the reduction of the TPTZ compound in the presence of an antioxidant was developed according to the research (Benzie and Strain Citation1996). The activity of the fibers extract toward iron ions was measured at different extract concentrations, which were prepared by diluting the sample solution with 40% ethanol. Then, 0.5–2.5 ml of the sample solution was supplemented with 40% ethanol to a final volume of 2.5 ml. The test solution was prepared in a test tube containing 3.0 ml of the TPTZ solution, 0.1 ml of the sample solution at five different concentrations, and 0.3 ml of water. The tubes were incubated for 4 minutes in a water bath at 37°C. After cooling, the absorbance was measured at λ = 593 nm by comparing it with a blank solution, using 3 replicates. The blank solution was prepared with 3.0 ml of the TPTZ solution, 0.1 ml of 40% ethanol, and 0.3 ml of water. This procedure was repeated for the samples with the extract. A fresh solution was prepared by mixing 30 mM TPTZ with 40 mM chlorous acid, 300 mM acetate buffer (pH = 3.6), and a 20 mM solution of ferric chloride (III) in a volume ratio of 1:10:1.
The determined absorbance value of the sample is directly proportional to the concentration of the antioxidant, which was then converted to FRAP units using a comparison with a standard solution, according to the equation (Wilczyńska Citation2009):
Determination of the 2, 2-diphenyl-1-picrylhydrazyl (DPPH) radical scavenging activity
The method used for determining antioxidant activity using the DPPH (2,2-diphenyl-1-picrylhydrazyl) solution was adapted for the purposes of this study based on research (Huang, Ou, and Prior Citation2005; Katalinic et al. Citation2006; Qian and Nihorimbere Citation2004). The activity of the fibers extract against free radicals was measured at five different extract concentrations, prepared by diluting the sample solution with 40% ethanol. The 0.5–2.5 ml of the sample solution was added to the test tubes and then the volume was brought up to 2.5 ml with 40% ethanol. The test solution was prepared in a test tube containing 3.9 ml of DPPH solution in ethanol (6 × 10^-5 M) and 0.1 ml of the sample solution at various concentrations. The test solution was mixed and stored in darkness. After 30 minutes, the absorbance of the test solution was measured at λ = 515 nm by comparing it with 40% ethanol with three replicates. The differences in absorbance between the samples and the control were calculated and expressed as the percentage DPPH radical scavenging capacity using the following formula (Pekal Citation2014):
I% – inhibition of DPPH radical [%],
A0 – initial absorbance value of DPPH radical,
At – absorbance of DPPH radical after a specified reaction time (t).
Furthermore, using the calibration curve and linear equation, the parameter IC50 was determined, which indicates the required concentration of the antioxidant-containing substance to scavenge 50% of the initial DPPH radicals.
Chemical analyses
Lignin content
The determination of lignin content in flax fibers was carried out according to the Industry Standard BN-86/7501–11 - Textiles – Test methods for textile raw materials – Flax and hemp fibers – Determination of lignin content. The lignin content was determined by treating the grinded fibers (a cutting mill SM 100; RETSCH with a 1 mm sieve) with a mixture of concentrated sulfuric acid and orthophosphoric acid to dissolve cellulose, hemicellulose and pectin. The insoluble lignin was then filtered on a G4 filter crucible and dried to a constant weight. The lignin content was calculated using the following formula:
– lignin content [%],
– mass of lignin after drying [g],
– initial mass of the sample [g],
– sample moisture [%].
FTIR-ATR analysis
The spectroscopic analysis using the Fourier Transform Infrared Attenuated Total Reflectance (FTIR-ATR) method was conducted to identify and characterize the organic and inorganic substances present in the fibers. The FTIR-ATR analysis measures the intensity of infrared radiation after passing through the sample and utilizes the data to determine the chemical composition of the sample. The analysis was performed using the iS10 model from TA Instruments, with the Smart iTX_ZnSe accessory. Spectra for each sample consisted of 32 scans with a resolution of 4 cm−1 in the range of 600 to 4000 cm−1.
Results and discussion
In agriculture, the selection of plant varieties plays a key role, which involves selecting and breeding plants in order to obtain new varieties that combine the desired characteristics. The intention of this process is also to obtain varieties that are more adapted to specific cultivation parameters, taking into account climatic, soil and topographic conditions. The types of flax selected in the experiment are varieties commonly grown in Poland for fibers production. The Artemida variety is characterized by high resistance to fusarium wilt and lodging. The vegetation period is 104 days. The height of the plants is described as medium-high. Artemida is distinguished by the high quality of its long fiber. The length of the growing season of the Modran variety is 100 days. It is also characterized by high resistance to fusarium blight and lodging and very good quality of long fiber. The height of the plants is considered average. The Sara fiber flax variety is characterized by a high yield of ginned straw (86.7 dt/ha), seed yield (9.4 dt/ha), and fiber efficiency of approximately 22%. The length of the growing season is 106 days. The plants, in favorable conditions, reach a height of approx. 75 cm. The variety is resistant to fusarium blight and lodging and is characterized by good quality of long fiber (Heller Citation2012).
Phenolic acids content
The antioxidant properties of substances result from content of antioxidants, e.g. phenolic acids conditioned by their chemical structure. The number of hydroxyl groups and their position in the aromatic ring affect the activity of these compounds. Acids with substituents located in the ortho- or para-position exhibit the strongest antioxidant activity (Staszek Citation2012). Two types of phenolic acids are distinguished: hydroxybenzoic acid and hydroxycinnamic acid. The most popular derivatives of hydroxybenzoic acid include gallic acid, p-hydroxybenzoic acid, protocatechuic acid, vanillic acid and syringic acid, while derivatives of hydroxycinnamic acid include caffeic acid, ferulic acid, p-coumaric acid, and sinapic acid (Shamanin et al. Citation2022). Results of published research indicate that derivatives of cinnamic acid are more effective antioxidants than derivatives of benzoic acid (Mitek and Gasik Citation2007). present the content of syringic acid, sinapic acid, p-coumaric acid, and ferulic acid in flax fibers derived from different varieties subjected to drought stress during plants growth.
Table 2. The content of selected phenolic acids in flax fibers derived from different varieties subjected to drought stress during growth in 2019.
Table 3. The content of selected phenolic acids in flax fibers derived from different varieties subjected to drought stress during growth in 2020.
Table 4. The content of selected phenolic acids in flax fibers derived from different varieties subjected to drought stress during growth in 2021.
The statistical analysis was conducted for flax varieties cultivated for all three years in condition of different moisture content in soil. The analysis of variance of factors for the content of p-coumaric acid in flax fibers in the mixed scheme showed that the fibers of the Modran and Sara varieties had a statistically significant lower content of a given phenolic acid compared to the fibers of the Artemida variety. The average content of p-coumaric acid was statistically significantly the highest in the fibers in the case of soil moisture at the level of 25% FWC, while its lowest content was recorded in the condition of 35% FWC of the soil. Fibers of the Sara variety were characterized by the significantly highest content of syringic acid compared to fibers of the Modran and Artemida varieties. At the level of 25% FWC of the soil, the fibers had significantly the lowest content of syringic acid compared to other moisture conditions. Cultivation of flax in soil moisture at the level of 35% FWC resulted in a higher content of syringic acid in the fibers than 45% FWC soil. Artemida fibers had the highest ferulic acid content. In the case of soil moisture of 45% FWC, the content of ferulic acid in the fibers was significantly higher compared to 25% and 35% FWC soil.
Antioxidant activity
Frap
The FRAP method, which allows the determination of the ability to reduce iron ions, enables the direct assessment of the reducing capacity of a given compound. The antioxidant properties of the samples were determined by comparing the changes in absorbance of the solution with the absorbance value of the standard solution. The absorbance, for each case, changes linearly over a wide range. It is assumed that one FRAP unit corresponds to the ability to reduce 1 mole of Fe3+ to Fe2+ (Wilczyńska Citation2009). The reduction potential of flax fibers extracts ranged from 12.72 to 300.83 µg/l FRAP, depending on the applied sample concentration. By using the results of the sample concentration’s impact on the level of antioxidant activity, a calibration curve along with its equation was determined for each case. The assessment of the iron ion reduction ability was based on one selected solution concentration = 4 mg/ml ().
Figure 1. The reduction abilities of iron ions by fiber extracts at a concentration of 4 mg/ml for samples from 2019, 2020, and 2021.

The analysis of the results of tests on the ability of flax fibers to reduce metal ions confirms that out of 9 analyzed cases:
in five cases, the highest FRAP index was found for flax fibers grown under the highest soil moisture, with FRAP increasing with the increase of the FWC
in two cases, the highest FRAP index was found in flax fibers grown in conditions at the level of 35% FWC
in five cases, the lowest FRAP index was observed in flax fibers grown under drought stress conditions 25% FWC
in no case was the highest FRAP value recorded for the fibers supplied by plants growing under the drought stress of 25% FWC
Dpph
The antioxidant activity of flax fibers extracts was expressed as percentage inhibition (I%) of DPPH radical, ranging from 0.24% to 15.99% at the given concentrations. The IC50 parameter, which represents the concentration of antioxidant substance required to scavenge 50% of the initial DPPH radicals, was calculated using a linear regression equation (). A higher IC50 value indicates lower efficiency of the antioxidant. The antioxidant activity tested by the DPPH method corresponds to the results of flax fibers using the FRAP method in the case of the Modran variety in 2020 and 2021, where the highest level of antioxidant activity of fibers from plants grown under control conditions of 45% FWC was recorded, and the lowest for 25% FWC.
Figure 2. The required concentration of the antioxidant-containing substance to scavenge 50% of the initial DPPH radicals for the samples from 2019, 2020, and 2021.

No fibers obtained from each tested variety of flax grown under drought stress 25% FWC reached the highest level of antioxidant activity, with the exception of Sara grown under drought stress in 2020.
Lignin content
The chemical analysis of flax fibers involved determining the lignin content after the removal of waxy and fatty substances. A comprehensive analysis including the determination of cellulose, hemicellulose, and pectin was not possible due to insufficient material obtained from the conducted pot experiment. Zimniewska demonstrated strong correlations between the lignin content in the fibers and the content of ferulic acid and p-coumaric acid. Fibers with the highest content of these components exhibited the highest antioxidant activity (Zimniewska Citation2015).
The lignin content in the fibers in 2019 () ranges from 4.79% to 6.16%. The highest value is observed in fibers from the Artemida variety grown under the highest drought stress (A25). The content slightly decreases with increasing soil moisture. Among the Modran variety, fibers from the intermediate soil moisture level (35% FWC) exhibit the highest lignin content. For the Sara variety, the parameter increases with increasing moisture and reaches the highest value in the control group (S45). The lignin content in the fibers in 2020 ranges from 4.85% to 7.06%. For the Artemida variety, the highest value is observed in fibers from the highest drought stress (A25), followed closely by the control group (A45). The Modran variety shows the highest lignin content in fibers from plants grown under intermediate soil moisture level 35% FWC (M35). The lignin content in the control (M45) and drought stress (M25) groups is similar. For the Sara variety, soil moisture during growth does not significantly affect the lignin content in the fibers. The lignin content in the fibers in 2021 ranges from 4.53% to 7.76%. For the Artemida variety, the highest lignin content is observed in fibers from the highest drought stress (A25), where the amount of lignin decreases with increasing moisture. The Modran and Sara varieties show similar patterns, with no noticeable differences between drought stress and the intermediate soil moisture level at 35% FWC. However, the lignin content decreases with increasing moisture (M45, S45).
Post hoc tests showed that in 2019 the lignin content in the fibers was significantly the lowest compared to 2020 and 2021. The main effect of the flax variety also turned out to be statistically significant – the fibers of the Sara variety were characterized by a significantly higher lignin content than the other varieties. There was also a statistically significant main effect of the level of soil moisture during plant cultivation. In the case of 45% FWC, the lignin content in the fibers was significantly lower compared to 25% FWC and 35% FWC soil. Statistically significant interaction of the flax cultivar with the level of soil moisture during cultivation consisted in the fact that in the case of the Artemida variety, soil moisture at the level of 25% FWC resulted in a lower content of lignin compared to 35% FWC and 45% FWC. On the other hand, in the case of Modran and Sara cultivars, soil moisture at the level of 35% FWC influenced a higher lignin content in the fibers than 25% and 45% PPW. In the case of 25% and 45% of the soil PPW, the fibers of the Modran variety were characterized by the lowest level of lignin content compared to the other two varieties. On the other hand, within 35% of the PPW, the fibers of the Artemida variety showed a significantly lower average content of lignin than Sara and Modran.
FTIR-ATR analysis
The FTIR-ATR spectrophotometric analysis was performed in order to obtain information on the molecular structure of the top layer of flax fibers, e.g. in order to identify compounds and to confirm the presence of phenolic acids. The infrared spectra of the tested fibers are used to identify the absorbance ranges representing the vibrations of such functional groups as: O-H, C=O, C=C, COO, C-H, CH2, CH3, COC, which come from chemical components in the fiber (Konczewicz, Zimniewska, and Valera Citation2017). The analysis of the impact of drought level and the flax variety from a given year did not result in significant differences between the occurrence of peaks in a given wave number range (). There are only slight shifts in the given peaks, which may be responsible for the vibrations of the same type of functional groups.
Figure 4. The FTIR – ATR spectra of flax fibers: artemida, Modran and Sara varieties from control and drought stress in 2019.

Figure 5. The FTIR – ATR spectra of flax fibers: artemida, Modran and Sara varieties from control and drought stress in 2020.

Figure 6. The FTIR – ATR spectra of flax fibers: artemida, Modran and Sara varieties from control and drought stress in 2021.

Based on the infrared tests, it was shown that the fibers of the following varieties: Artemida, Modran and Sara, from the control and drought stress in 2019. 2020 and 2021 show absorption in the range of:
3300 – 3600 cm− 1 : a wide band of OH stretching vibrations of the hydroxyl group, which occur in cellulose, the main component of flax fibers, as well as in hemicellulose, pectin, waxes and fats
2800 – 3000 cm− 1 : several bands of CH and CH2 vibrations of aliphatic groups can be observed
1700 – 1750 cm− 1 : C=O vibration bands of the carbonyl group are visible, which are characteristic of organic acids, ketones and aldehydes, and in linen fibers they are present in lignin, pectin, waxes and fats
1500 – 1600 cm− 1 : C=C stretching vibrations occurring in the aromatic ring, which are characteristic of lignin
1627 – 1637 cm− 1 : OH vibration bands are visible, originating from water adsorbed by the fiber
1050 – 1150 cm− 1 : stretching bands of COC vibrations of the ether group are visible, which occur in cellulose, hemicellulose and pectin
The analysis of vibration bands for phenolic acids can be identified based on the occurrence of stretching vibrations of the Ar-OH phenolic group present in lignin. This is due to the fact that phenolic acids are extracted from lignin. Spectrophotometric separation of individual chemical compounds of the fiber is not possible due to the presence of identical functional groups in different components of the fiber. The analysis of spectra of fibers from all years of experiment, varieties and the level of FWC used allowed to determine the occurrence of common bands in the wavelength range with only small shifts ().
Table 5. Analysis of the type of vibrations and functional groups for range of wavenumbers of FTIR – ATR fiber spectra.
Conclusions
Nature is extremely complex and difficult to predict. Uncontrolled changes in external factors can lead to unpredictable reactions that occur in the plant during its growth. The antioxidant activity of flax fibers is a parameter conditioned by many factors, including depends on the variety and the method of retting the flax straw. The dew retting of flax straws on the field causes activation of microorganisms, mainly fungi and bacteria that decompose pectic substances, partially also lignin and hemicellulose. Aggressive microorganisms and the resulting enzymes can affect the content of phenolic acids and thus the ability of the fiber to fight free radicals. The dew retting is a method that is highly weather dependent and cannot be controlled. It was impossible to ensure the same air temperature and humidity and the same amount of precipitation in 2019, 2020, 2021 of the experiment, which could cause differences in the FRAP and DPPH results of the research.
In conclusion, it is very likely that the conditions of cultivation of flax plants in the range of soil moisture at the level of 45% FWC are conducive to the formation of antioxidant activity in the fiber. At the same time, it should be noted that the drought stress at the level of 25% of the FWC limits the formation of fibers with high antioxidant activity in all the tested varieties of flax. This should be explained by the fact that a plant growing in difficult conditions of drought stress uses the antioxidant reserve fighting for survival, thus the fiber from these plants will be characterized by lower antioxidant activity.
Highlights
Three different varieties of flax were grown in the vegetation hall with constant levels of irrigation: 25%, 35%, and 45% of field water capacity of the soil. The experiment was conducted three times in 2019, 2020, 2021.
The content of phenolic acids in flax fibers was determined using high-performance liquid chromatography (HPLC) with a diode array detector (DAD) and the antioxidant activity was determined using the FRAP and DPPH methods.
Chemical analysis of fibers involved determining the lignin content in the fiber and analyzing the results of FTIR-ATR tests.
Drought during the growth of flax plants has a negative impact on the development of antioxidant activity in flax fibers.
Drought during plant growth had a positive effect on the lignin content in flax fibers.
Author’s contribution
Conceptualization: Małgorzata Zimniewska, Edyta Kwiatkowska
methodology: Edyta Kwiatkowska, Małgorzata Zimniewska,
software: Edyta Kwiatkowska
validation: Edyta Kwiatkowska
formal analysis: Edyta Kwiatkowska
investigation: Edyta Kwiatkowska
resources: Edyta Kwiatkowska, Patrycja Przybylska, Agnieszka Gryszczyńska, Wanda Różańska
data curation: Edyta Kwiatkowska
writing – original draft preparation: Edyta Kwiatkowska, Małgorzata Zimniewska
writing – review and editing: Małgorzata Zimniewska, Edyta Kwiatkowska
visualization: Edyta Kwiatkowska, Patrycja Przybylska, Agnieszka Gryszczyńska, Wanda Różańskaa
supervision, Małgorzata Zimniewska
project administration: Edyta Kwiatkowska
All authors have read and agreed to the published version of the manuscript.
Ethical approval
We confirm that all the research meets ethical guidelines and adheres to the legal requirements of the study country. The research does not involve any human or animal-welfare-related issues.
Disclosure statement
No potential conflict of interest was reported by the author(s).
Additional information
Funding
References
- Benzie, I. F. F., and J. J. Strain. 1996. “The Ferric Reducing Ability of Plasma (FRAP) as a Measure of Antioxidant Power: The FRAP Assay.” Analytical Biochemistry 239 (1): 70–14. https://doi.org/10.1006/abio.1996.0292.
- Czajka, A. 2006. “Wolne rodniki tlenowe a mechanizmy obronne organizmu.” Nowiny Lekarskie 75 (6): 582–586.
- Dzialo, M., J. Mierziak, U. Korzun, M. Preisner, J. Szopa, and A. Kulma. 2016. “The Potential of Plant Phenolics in Prevention and Therapy of Skin Disorders.” International Journal of Molecular Sciences 17 (2): 1–41. 160. https://doi.org/10.3390/ijms17020160.
- Gryszczynska, A., B. Opała, Z. Łowicki, M. Dreger, M. Górska-Paukszta, M. Szulc, E. Kamińska, E. Litwin, P. Struzik, and W. Dyr. 2015. “Bioactive Compounds Determination in the Callus and Hydroalcoholic Extracts from Salvia Miltiorrhiza and Salvia Przewalskii-Preliminary Study on Their Anti-Alcoholic Activity Effects.” Phytochemistry Letters 11:399–403. https://doi.org/10.1016/j.phytol.2014.11.009.
- Heller, K. 2012. Metodyka integrowanej ochrony roślin dla uprawy lnu włóknistego, Instytut Włókien Naturalnych i Roślin Zielarskich w Poznaniu; Poznań. Accessed March 3, 2023. https://www.agrofagi.com.pl/260,pliki-ukryte-w-menu?nobreakup#plik_577.
- Heller, K., and M. Byczynska. 2015. “The Impact of Environmental Factors and Applied Agronomy on Quantitative and Qualitative Traits of Flax Fiber.” Journal of Natural Fibers 12 (1): 26–38. https://doi.org/10.1080/15440478.2013.879088.
- Huang, D., B. Ou, and R. L. Prior. 2005. “Prior the Chemistry Behind Antioxidant Capacity Assays.” Journal of Agricultural and Food Chemistry 53 (6): 1841–1856. https://doi.org/10.1021/jf030723c.
- Katalinic, V., M. Milos, T. Kulisic, and M. Jukic. 2006. “Screening of 70 Medicinal Plant Extracts for Antioxidant Capacity and Total Phenols.” Food Chemistry 94 (4): 550–557. https://doi.org/10.1016/j.foodchem.2004.12.004.
- Konczewicz, W., M. Zimniewska, and M. A. Valera. 2017. “The Selection of a Retting Method for Extraction of Bast Fibers as Response to Challenges in Composite Reinforcement.” Textile Research Journal 88 (18): 2104–2119. https://doi.org/10.1177/0040517517716902.
- Kwiatkowska, E., M. Zimniewska, P. Przybylska, and B. Romanowska. 2023. “Effect of Drought Stress on Quality of Flax Fibres.” Materials 16 (3752): 1–14. https://doi.org/10.3390/ma16103752.
- Liu, A. H., L. Li, M. Xu, Y. H. Lin, H. Z. Guo, and D. A. Guo. 2006. “Simultaneous Quantifi-Cation of Six Major Phenolic Acids in the Roots of Salvia Miltiorrhiza and Four Related Traditional Chinese Medicinal Preparations by HPLC–DAD Method.” Journal of Pharmaceutical & Biomedical Analysis 41 (1): 48–56. 11. https://doi.org/10.1016/j.jpba.2005.10.021.
- Mishra, D. K., and H. Awasthi. 2021. “Quality Evaluation of Flaxseed Obtained from Different Locations.” Biology and Life Sciences Forum 4 (1): 1–6. 70. https://doi.org/10.3390/IECPS2020-08754.
- Mitek, M., and A. Gasik. 2007. “Polifenole w żywności. Właściwości przeciwutleniające.” Przemysł Spożywczy 61 (9): 36–39.
- Muzykiewicz, A., J. Zielonka-Brzezicka, A. Nowak, and A. Klimowicz. 2019. “Aktywność antyoksydacyjna ekstraktów sporządzonych ze świeżych i suszonych surowców Ficus carica L.” Hygeia Public Health 54 (3): 201–209.
- Pekal, A. J. 2014. “Wpływ doboru procedury analitycznej na wyznaczanie właściwości antyutleniających próbek żywności.” PhD Thesis, University of Warsaw.
- Qian, H., and V. Nihorimbere. 2004. “Antioxidant Power of Phytochemicals from Psidium Guajava Leaf.” Journal of Zhejiang University Science 5 (6): 676–683. https://doi.org/10.1631/jzus.2004.0676.
- Shamanin, V. P., Z. H. Tekin-Cakmak, E. I. Gordeeva, S. Karasu, I. Pototskaya, A. S. Chursin, V. E. Pozherukova, et al. 2022. “Antioxidant Capacity and Profiles of Phenolic Acids in Various Genotypes of Purple Wheat.” Foods 11 (16): 1–14. 2515. https://doi.org/10.3390/foods11162515.
- Staszek, D. 2012. “Badania związków fenolowych w wybranych gatunkach szałwii (Salvia sp.) metodami chromatograficznymi HPLC i TLC.” PhD Thesis, University of Silesia, Katowice.
- Wilczyńska, A. 2009. “Metody oznaczania aktywności antyoksydacyjnej miodów pszczelich.” Bromatologia i Chemia Toksykologiczna 42 (3): 870–874.
- Zimniewska, M. 2015. “Antioxidant Activity of Fibres Originating from Traditional Varieties of Polish Flax Plants.” Fibres & Textiles in Eastern Europe 23 (6(114)): 41–47. 23. https://doi.org/10.5604/12303666.1167418.
- Zimniewska, M., W. Różańska, A. Gryszczyńska, B. Romanowska, and A. Kicińska-Jakubowska. 2018. “Antioxidant Potential of Hemp and Flax Fibers Depending on Their Chemical Composition.” Molecules 23 (8): 1–17. 1993. https://doi.org/10.3390/molecules23081993.