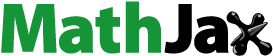
ABSTRACT
This study focuses on developing a fiber stacking of polymer laminate for high-impact load-absorbing applications, such as aircraft, automotive, and soundproofing structures. Therefore, the effect of high-strength fiber position effect on the tensile and dynamic behaviors of woven glass intertwined kenaf fiber thermoset composites was investigated in this research work. The tensile strength and dynamic mechanical properties of a hybrid stacking composite designed with maximum glass fiber, especially glass as an outer layer, are relatively higher. The outer layer of glass fiber is particularly effective in containing stress due to its high-strength characteristics, reducing stress transmission to the other core layers. The maximum storage of outer glass and inner kenaf woven stacking composite S1 were 7692.4 MPa, respectively. Besides, the most significant improvement in damping value was observed for the outer glass stacking kenaf (S1) composite compared to the all-other woven glass stacked composite. Furthermore, the composite with the outer glass layers and inner kenaf layers shows a more significant damping value due to the porous structure of the kenaf fibers, allowing for more excellent vibration energy dissipation. Overall, the stacking composites with a glass and kenaf layering arrangement exhibit good mechanical properties for high-impact load-absorbing applications.
摘要
这项研究的重点是开发一种用于高冲击载荷吸收应用的聚合物层压板纤维堆叠,如飞机、汽车和隔音结构. 因此,本文研究了高强度纤维位置效应对玻璃纤维交织红麻纤维热固性复合材料拉伸和动态性能的影响. 以最大玻璃纤维,特别是玻璃作为外层设计的混合堆叠复合材料的拉伸强度和动态力学性能相对较高. 玻璃纤维外层由于其高强度特性,在控制应力方面特别有效,减少了应力传递到其他芯层. 外层玻璃和内层红麻编织堆叠复合材料S1的最大储存压力分别为7692.4MPa. 此外,与所有其他编织玻璃堆叠复合材料相比,外层玻璃堆叠红麻(S1)复合材料的阻尼值提高最为显著. 此外,由于红麻纤维的多孔结构,具有外部玻璃层和内部红麻层的复合材料显示出更显著的阻尼值,从而允许更优异的振动能量耗散. 总体而言,具有玻璃和红麻分层排列的堆叠复合材料在高冲击载荷吸收应用中表现出良好的机械性能.
Introduction
Natural fibers are a popular choice for reinforcing Natural Fiber Reinforced Polymer (NFRP) composites in a variety of in structural and infrastructure applications, such as, civil structures, structural components, automotive industries, furniture, sports, aerospace, low-cost housing, packing marine structures and so forth (Jariwala and Jain Citation2019; Ramesh, Palanikumar, and Reddy Citation2017). Natural fibers are more environmentally friendly and economical than synthetic fiber. In addition, natural fibers have many benefits: biodegradability, high damping qualities, lower density, wide availability, and increased health safety (Chaudhary and Ahmad Citation2020; Kirubakaran, Rajkumar, and Rajesh Citation2022). Natural fibers are more available which are taken from plants, such as kenaf, jute, palm, phoenix fibers, banana, flax, hemp, coir, and ramie fiber, are fully bio-fibers (Aisyah et al. Citation2021; da Silva et al. Citation2022). Researchers that examined the kenaf fiber’s mechanical characteristics confirmed that it possesses several important mechanical characteristics. Furthermore, kenaf plant fibers’ strength and energy absorption are increased by their pectin, low wax, and water-reduced substance contents (Yosr et al. Citation2022). Additionally, numerous research studies revealed that using hybridization technique resolves the composite’s mechanical and dynamic properties, combining high-strength fibers like glass, carbon and kevlar synthetic fiber position with low-strength natural fiber (Calabrese et al. Citation2019; Genc and Ozdemir Citation2020; Jamshaid et al. Citation2016).
Numerous investigations on the thermomechanical properties (tan delta, storage, and loss modulus) of hybridization with various natural fiber reinforcements with high strength fiber utilized in the polymer matrix (Pothan et al. Citation2010; Selvaraj, Kaliyamoorthy, and Kirubakaran Citation2022). The mechanical and dynamic properties of hybrid fiber polymer (HFP) composites can be tailored to meet specific requirements by adjusting various factors, such as fiber content, fiber angle. fiber length, stacking layers, reinforcement, adhesion between fibers and matrix, fiber treatment, fabrication technique, and curing process. Many studies have shown that hybridizing and stacking multilayer woven polymer composites improve their mechanical and dynamic properties compared to randomly fiber-oriented composites (Sreenivasan et al. Citation2015; Amico, Angrizani; Prabhu et al. Citation2021). In addition, stacking of fiber in the hybrid polymer composite improves mechanical and dynamic properties based on inner and outer fiber position layers. The study examined how stacked oil palm fiber/glass fiber hybridization in the fiber sequence affected the mechanical characteristics of a polymeric laminate. Results showed that the position of the inner palm fiber and the outside synthetic glass fiber in the stacked composite increased the mechanical strength (Mohanavel et al. Citation2021).
Many studies have focused on FRP composites’ vibration properties (damping frequency and ratio). Specifically, natural fiber enhanced the vibrational properties due to its internal porous structure, which absorbed more energy (Kirubakaran et al. Citation2023; Palanikumar and Subbiah Citation2019). Phoenix natural fiber composites were created with varying fiber lengths, and their free vibration properties were investigated (Rajeshkumar and Hariharan Citation2014). The vibrational properties of the natural fiber composite were determined using a modal analysis technique. It was discovered that a composite that included Phoenix fibers with a length of 30 mm demonstrated the most considerable natural frequency. Moreover, the addition of bio natural fiber, natural filler, and stacking sequence enhanced the vibration characteristics (Bennet et al. Citation2015; Duc et al. Citation2014; Sreenivasan et al. Citation2015).
The literature analysis above, green materials are highly demanded for structural applications. By altering the fiber stacking and layer sequence, it is possible to improve polymer composites’ mechanical and dynamic properties. Mechanical and dynamic qualities are crucial to confirming hybrid composite construction for structural parts before they are used in real time. There is plenty of literature on the static and dynamic mechanical behavior of layered composites made of glass and natural fibers. The free vibration properties of stacked laminates with hybrid glass/kenaf fiber layers have not yet been reported. The effect of the position of the two fiber layers has not yet been documented. The current study examines woven glass intertwined kenaf-epoxy polymer laminates for their mechanical and dynamic properties. In addition, the impact of the fiber position on the interleaved laminates, outer and inner fiber layers was investigated. After the tensile test, the composite stacked fracture surface was evaluated using a scanning electron microscope (SEM).
Materials and methods
Thermoset resin, woven kenaf and glass
Sri Sai Sakthi Enterprises, Chennai, Tamil Nadu, provided the epoxy (EY 556) used as a thermoset polymer matrix. The ratio of resin to hardener (curing agent) for the composite preparation was 10:1. Araldite (HY951) is used as a curing agent. The kenaf and glass fabrics have a 0° weft orientation and a 90° warp orientation. Gogreen Products and Sri Sai Sakthi Enterprises of Tamil Nadu, India are the suppliers of these materials. Glass woven fabric (G) was employed as a high strength fiber reinforcing for developing kenaf fiber (K) stacked composite laminates.
Manufacture of layering sequence of glass stacking kenaf layers laminates
A hand lay-up process was employed to produce the kenaf/glass/epoxy layering sequence composite, followed by a compression load. Six fiber layers for each composite were used to maintain the 4 mm thickness of the composites. An alkaline chemical treatment was carried out on the kenaf fiber before it was used in the fabrication of the kenaf stacking laminate. The alkaline surface treatment of natural fiber increased the matrix-adhesion (Sreenivasan et al. Citation2015). After three hours of soaking in a 5% NaOH solution for the alkaline treatment, the plant fiber was neutralized with diluted hydrochloric acid. The chemically treated kenaf fibers were cleaned with tap, dried in the sunlight, and then cured in a hot oven box furnace for one hour at 60°C. depicts the various stacking laminate layering sequences arrangement and hand layup fabrication process. shows the description of stacked composites and their weight fractions of fiber & matrix.
Table 1. Description of stacked composites and their weight fractions of fiber & matrix.
Tensile test
A dog bone-shaped standard specimen was prepared for the tensile test utilizing a Universal Testing Machine (UTM) with two grippers under ASTM D638 requirements. Between these two grippers, the specimen was positioned, and the moveable end was stretched at a constant strain rate. The tensile standard sample size, both before and after the sample was fractured, is shown in .
Fractography analysis
Photographs of the composite’s tensile load fracture surface were taken using a scanning electron microscope (SEM) with the model number JOEL JSM 840A. Before that, the composite sample gold spattering was conducted for 15 min to improve the contact surface of the kenaf hybrid composite. SEM techniques to shed light on the fractured damage mechanism were used.
Dynamic mechanical analysis (DMA)
The ASTM D 4065–01 standards were followed, and DMA was used to assess the viscoelastic properties of the layering sequence stacking composites. A dual cantilever mode setup was used for the DMA testing, and samples of woven layering sequences were put to the test at a constant temperature rate of 2°C/min from ambient temperature to 180°C. At a frequency of 1 Hz, the dynamic properties, such as the storage, loss, and tan delta modules, were examined in this study.
Vibrational analysis
The shaker vibration test equipment, depicted in , was used to assess the vibration properties (damped frequencies and damping ratio) of kenaf natural fiber stacked composite samples in the type of cantilever beam set up. The frequency response of the clamped free beam after the impact hammer is used to trigger a vibration at the clamped end, and then the accelerometer collects the response frequency. ASTM E-756 was used to fabricate specimens of 150 × 40 * 4 mm3 for vibration tests. shows the 3 dB bandwidth method, commonly known as the cutoff frequency method. Equation 1 illustrates the relationship between the damping ratio (δ), damping factor (Qn), and loss factor (n), and displays the estimated damping ratio. The composites’ damping ratio was determined using equation 1 (Ramraji, Rajkumar, and Sabarinathan Citation2020).
where Δf3dB − 3 dB points for different between frequency, n- mode number.
Results and discussion
Tensile strength of stacking layered composites
shows the tensile strength results for the investigated glass/kenaf stacked composites. By observing the various placements of the glass layers, it can infer their tensile characteristics. However, its tensile value is lower than that of every other stacked composite, the pure kenaf composite (BS-base sample). Glass fiber layer position affects the tensile strength of stacked layered composites. The S1 stacked composite of an outer glass and an inner kenaf stacking layer showed the highest tensile strength. The surface stress on the surface high strength glass fiber positively increased the tensile strength. These results are in line with research on building composites made of basalt and flax fibers mixed with epoxy, which showed a greater tensile value than earlier stacking layered composites using high-strength basalt fiber as an outer skin layer (Ramraji, Rajkumar, and Sabarinathan Citation2020). In the S2 composite, the stacked layer of outer kenaf to the next glass layer composite tensile strength was 84 MPa, reduced by 18.4% compared to the stacked S1 composite. This fiber layer stacked design’s tensile strength is related to the layers of outer. Determined similar results of stacking fiber layer composites (Amico, Angrizani, and Drummond Citation2010). The outside glass layers of the outer hold the tensile load, transmitting less stress to the inner layers. On the other hand, the S3 stacked polymer composite showed a minimum tensile strength than the all-other stacked composite. This is because low strength outer fiber layers (Ramraji, Rajkumar, and Sabarinathan Citation2020; Shi et al. Citation2023). Two layers of glass fiber with four-layers of kenaf fiber composite showed higher tensile strength than S2 and S3 stacked polymer composites but less than the S1 stacked composite. The stacking order and skin layers have a greater impact on the layered composite’s tensile strength. When glass fiber is situated on the outside surface of an S4 stacked composite, the tensile strength increases. Overall, the mechanical tensile property test results showed that the position of glass layers and outer layer glass had a higher tensile strength value in stacking polymer composites. depicts the typical stress-strain curves of fiber sequence stacked composites. The BS composite had less stiffness than the other stacked composite, as seen in the figure. The increased stiffness of the material can be attributed to the superior strength of glass fibers and their improved interfacial adhesion with the matrix compared to kenaf fibers. As a result, the curves of stacked composites were the stiffness of all the glass and kenaf-stacked layer composites increased, as seen in .
Fractography analysis
The fractured tensile specimens of S1, S2, S3, S4 and BS stacked composites are depicted in . Fracture mechanisms such as fiber pullout, splitting, breakage, and debonding from the thermoset matrix were observed for kenaf glass fiber stacked polymer composites. Fiber splitting in an S1 composite is depicted in and both the fibers transverse and longitudinal directions are visible. These results are similar to what was found in references (Das et al. Citation2021; Ramraji, Rajkumar, and Sabarinathan Citation2020) which looked at the surface of composites with natural fiber hybrid glass layers of polymer composite fracture. Delamination of kenaf layers is also seen due to the poor bonding between the waxy layer fiber and the matrix. Kenaf fiber layers lead to delamination on load application due to poor interfacial bonding and mechanical properties (. The formation of voids in pure kenaf composites is caused by the weak adhesion between the fiber and the matrix resin (. The figure reveals just a few kenaf fiber pull-outs, which may be caused by ineffective kenaf-epoxy bonding and matrix and fiber cracking. Clusters of kenaf fiber-matrix were typical for S3 composites. SEM images of kenaf fiber hybrid S4 composites have identified fracture processes such as fiber cluster, void, and debonding from the epoxy matrix from . Similar findings were made when both basalt textiles were positioned on the tensile sides: the natural fibers break and explode into many strands, and interfaces are almost destroyed (Jamshaid et al. Citation2016).
Dynamic mechanical analysis
Storage modulus of kenaf stacked hybrid composites
In dynamic mechanical analysis, the storage modulus is an important property. At a particular temperature, it is the energy absorbed by oscillation time. displays the storage modulus difference between pure kenaf and glass/kenaf stacking layered composites at 1 Hz frequency. The base sample pure kenaf composite has the lowest storage modulus (3682.7 MPa) compared to the other stacking layered composites. This is due to the hybrid reinforcement with a small amount of glass fiber layer increasing the stiffness of the composite laminates (Pothan et al. Citation2010). The various positions of glass fiber in the layered stacking composite (S1, S2, S3, S4) decided the storage modulus properties. The storage modulus (7692.4 MPa) of the S1 composite is greater than that of the all stacking layered composite. This is owing to the immobility of the polymer chains due to the materials’ high stiffness in that state. The stress-strain curve in reveals that the outer layer glass fiber has higher stiffness than kenaf fiber (Das et al. Citation2021; Sreenivasan et al. Citation2015). Including a glass layer with high rigidity enhanced the storage modulus of DMA characteristics. In DMA, increasing the storage modulus of the composite by covering it with strong-stiffness glass. Polymer composites frequently exhibit two distinct zones, glassy and rubbery, as the testing temperature rises. Before they reach the glassy state, fiber-reinforced polymer composites often have a high storage modulus [33]. Due to the stiffness of composite components in this situation, the polymer chains are immobile. The storage modulus gradually decreases, especially at higher temperatures, as the polymer chains become more mobile in the rubbery state, which causes the elasticity of the composites to be reduced. The type of fibers and how they are stacked affect a composite’s capacity to transport polymer chains. The interweaving pattern and position of weak-strength kenaf and strong-strength glass fibers significantly impact the laminate’s dynamic mechanical properties [31].
Loss modulus of kenaf stacked hybrid composites
depicts the loss modulus curve of pure kenaf and its position in glass fiber stacked composites. The loss modulus of the pure kenaf base composite was lower than the other stacked composites. On the other hand, the loss modulus is higher for all glass-stacked layered polymeric composite ((S1, S2, S3, S4). As demonstrated, the glass layers enhance the loss modulus by restricting polymer chains. The S1 stacking layer of polymer composite had a maximum loss modulus compared to all other stacking layered polymer composite. The glass fiber’s exceptional reinforcing action allows for an excellent lossmodulus. Furthermore, the skin wire mesh design with glass and kenaf fiber (GKKKKG) successfully regulated molecular chain movement, increasing the loss modulus associated with the kenaf composites made of fibers. The more significant value shift is caused by glass fiber, a strong reinforcement in the layered stacking composite (Pothan et al. Citation2010). According to the testing results of the S3 stacking layered polymer composite, it has a lower loss modulus value than all glass stacking layered polymer composites (S1, S2, and S4).
Tan delta of kenaf stacked hybrid composites
Tan delta is the ratio of loss to storage modulus in viscoelastic materials. depicts the temperature dependence of the tan delta of pure kenaf and their hybrid kenaf/glass stacking composites. This figure shows that the pure kenaf composite shows the highest damping value (.6939). This increase is owing to a lack of stiffness and internal energy dissipations by natural kenaf fiber. All stacking composites’ damping values gradually reduced with rising temperature after Tg. As a result of stiffness relaxation by the free mobility of the polymer chains movement. Overall, the stacking position of glass fibers changed the Tg of composites to higher temperatures (Das et al. Citation2021). Moreover, Tan delta can be decreased in polymer composites with strong-stiffness fiber reinforcement. Fracture surface study demonstrated that too weak interfaces result in larger energy interior dissipation, hence damping values were increased for BS (KKKKKK) stacked designs.
Free vibration analysis of kenaf stacking layered hybrid composites
Damped natural frequency
The damped natural frequencies and damping for two vibration modes were obtained through an experimental study employing vibrations, as shown in and summarized in . From this figure, the damped natural frequency mode of point 1 for natural composites decreases as the base is a pure kenaf fiber composite (Florence et al. Citation2023). Besides, a small proportion of the glass layer enhanced the natural frequency of the composite. Compared to the hybrid stacked composite, the S1 composite obtained the highest natural frequency value for modes 1 and 2 at 149 Hz and 121 Hz, respectively. The first mode of vibration for all samples stacked in the composite ranges between 111–149 Hz. The S3 hybrid stacked sample had the lowest natural frequency at modes 1 and 2. The natural frequency was found that the higher for high strength of outer basalt layer and inner natural fiber layer composites (Ramraji, Rajkumar, and Sabarinathan Citation2020). The observation made by the natural frequency for various composite stackings of the second mode is in the range 85–121 (Hz) in . The figure also showed that the improvement in natural frequencies of point 2 vibration modes was greater in S4 stacked composite than S3. The hybrid stacked sample S3 in mode 2 has the lowest natural frequency (106 Hz). However, it was higher compared to pure kenaf composite due to the increased strength of synthetic glass fiber improved the natural frequency of the composites. Results indicated the stacked hybrid composites showed the highest natural frequency.
Table 2. Natural frequency and damping ratio of kenaf/glass fiber stacked composites.
Damping ratio
The damped natural frequencies for two vibration modes were obtained through an experimental study employing vibration damping, as shown in . The effects of epoxy-based stacked composite beams on damping values were explored experimentally. During mode 1, the stacked composite had nearly identical damping values in the .0287–.0572 range. Similarly, stacked composite has damping values in the .0586–.0741 range during mode 2. Compared to the other stacked hybrid composites, the S2 stacked composite had the highest damping value for mode 1 with a value of .0398. The outer layered glass-core layered kenaf fiber stacked composite (S3) has the highest damping compared to the damping peaks. Compared to the S1 composite, the S2 stacking composite showed improved damping (27.15%). The damping value of S4 was reduced by 9% compared to pure kenaf BS composite (Bennet et al. Citation2015). In stacking composites, S1 was a slightly higher damping value (.0313) than S4 (.0287) at mode 1. Similar results were observed in the second mode of vibrational damping. The typical photocopy natural frequency and corresponding damping ratio of the S1 kenaf hybrid stacked composite are shown in .
Conclusion
The current work aims of the current work is to create a new category of polymer laminate containing high strength glass fiber intertwined kenaf damping fiber. This study examines how the skin and core layers of glass/kenaf laminates affect their mechanical, dynamic mechanical, and free vibration properties. The result revealed that using glass fiber in the outer position and inner natural kenaf fiber (GKKKKG) showed maximum tensile strength. This is due to the high strength of glass fiber to hold the load transfer to the core layers. High thermomechanical properties such as storage and loss modulus were noted in a hybrid kenaf stacking composite consisting of glass as the outer layer and kenaf fiber as the inner layer. The storage modulus value of the S1 (7692.4 MPa) stacking composite improved by 40.1% when compared to S3 (5487.8 MPa) and 12.75% when compared to the twin glass outer S4 composite. According to the loss modulus results of S3 stacking layered polymer composite, it has a lower loss modulus value than all glass stacking layered polymer composites (S1, S2, and S4). However, pure kenaf composite and S1 stacking design increased the tan delta value. This is due to more natural fiber layers’ lack of stiffness and internal energy dissipation. The natural frequency was higher for glass outer layer composites (S1) at 149 Hz. The stacked hybrid composites’ inherent frequencies and damping levels improved the vibration behavior. The developed laminates can be employed as sandwich structural members, interior wall covers for aircraft, automotive, and soundproofing structures, coordinate measuring machine bases, and vibration isolators for machine components. The only thing that changes the properties of composites is how the polymer is processed. Thus, many processing techniques will be used in the future. Future research will examine how the orientation of glass fibers and kenaf affects mechanical, dynamic mechanical, and vibration properties.
Author contributions
K. Elangovan- Data collection, Project overview, R. Senthikumar – Data acquisition, Visualization, K. Ramraji - Investigation, Writing-Review & Editing, M. Rajesh- Material preparation, Supervision & Validation, E.S. Esakkiraj - Resources, Supervision, Project administration, K. Arunkumar - Visualization, Formal analysis. All authors read and approved the final manuscript.
Highlights
Kenaf and glass fiber stacked layered composites significantly affect tensile and dynamic properties.
Glass fiber outer layer that increased the tensile strength of the kenaf stacking composite.
Glass fiber increased the storage and loss modulus of the kenaf-stacked composite.
An outer glass layer and an inner kenaf fiber stacked composite enhanced the damped natural frequency.
Disclosure statement
No potential conflict of interest was reported by the author(s).
Additional information
Funding
References
- Aisyah, H. A., M. T. Paridah, S. M. Sapuan, R. A. Ilyas, A. Khalina, N. M. Nurazzi, S. H. Lee, and C. H. Lee. 2021. “A Comprehensive Review on Advanced Sustainable Woven Natural Fibre Polymer Composites.” Polymers 13 (3): 471. https://doi.org/10.3390/polym13030471.
- Amico, S. C., C. C. Angrizani, and M. L. Drummond. 2010. “Influence of the Stacking Sequence on the Mechanical Properties of Glass/Sisal Hybrid Composites.” Journal of Reinforced Plastics and Composites 29:179–13. https://doi.org/10.1177/0731684408096430.
- Bennet, C., N. Rajini, J. W. Jappes, I. Siva, V. Sreenivasan, and S. Amico. 2015. “Effect of the Stacking Sequence on Vibrational Behavior of Sansevieria Cylindrica/Coconut Sheath Polyester Hybrid Composites.” Journal of Reinforced Plastics and Composites 34 (4): 293–306. https://doi.org/10.1177/0731684415570683.
- Calabrese, L., V. Fiore, T. Scalici, and A. Valenza. 2019. “Experimental Assessment of the Improved Properties During Aging of Flax/Glass Hybrid Composite Laminates for Marine Applications.” Journal of Applied Polymer Science 136 (14). https://doi.org/10.1002/app.47203.
- Chaudhary, V., and F. Ahmad. 2020. “A Review on Plant Fiber Reinforced Thermoset Polymers for Structural and Frictional Composites.” Polymer Testing 91:106792. https://doi.org/10.1016/j.polymertesting.2020.106792.
- da Silva, T. T., P. H. P. M. D. Silveira, A. B.-H. D. S. Figueiredo, S. N. Monteiro, M. P. Ribeiro, L. D. M. Neuba, N. T. Simonassi, F. D. C. Garcia Filho, and L. F. C. Nascimento. 2022. “Dynamic Mechanical Analysis and Ballistic Performance of Kenaf Fiber-Reinforced Epoxy Composites.” Polymers 14 (17): 3629. https://doi.org/10.3390/polym14173629.
- Das, S. C., D. Paul, S. A. Grammatikos, M. A. B. Siddiquee, S. Papatzani, P. Koralli, J. M. M. Islam, et al. 2021. “Effect of Stacking Sequence on the Performance of Hybrid Natural/Synthetic Fiber Reinforced Polymer Composite Laminates.” Composite Structures 276:114525. https://doi.org/10.1016/j.compstruct.2021.114525.
- Duc, F., P. E. Bourban, C. J. G. Plummer, and J.-A. E. Månson. 2014. “Damping of Thermoset and Thermoplastic Flax Fibre Composites.” Composites Part A: Applied Science and Manufacturing 64:115–123. https://doi.org/10.1016/j.compositesa.2014.04.016.
- Florence, A., A. R. Jain, B. Tony, and K. Ramraji 2023. “Tailoring of Mechanical, Thermal and Vibration Behaviour of Hybrid Fibre with Core Honeycomb Energy-Absorbing Materials Reinforced Polymer Sandwich Laminates.” In Proceedings of the Institution of Mechanical Engineers, Part E: Journal of Process Mechanical Engineering. https://doi.org/10.1177/09544089231189609.
- Genc, G., and Y. Ozdemir. 2020. “Improvement of the Mechanical Properties of Plant Fiber-Reinforced Composites Through Hybridization.” Journal of Natural Fibers 19 (8): 2805–2812. https://doi.org/10.1080/15440478.2020.1821295.
- Jamshaid, H., R. Mishra, J. Militky, M. Pechociakova, and M. T. Noman. 2016. “Mechanical, Thermal and Interfacial Properties of Green Composites from Basalt and Hybrid Woven Fabrics.” Fibers and Polymers 17:1675–1686. https://doi.org/10.1007/s12221-016-6563-z.
- Jariwala, H., and P. Jain. 2019. “A Review on Mechanical Behavior of Natural Fiber Reinforced Polymer Composites and Its Applications.” Journal of Reinforced Plastics and Composites 38 (10): 441–453. https://doi.org/10.1177/0731684419828524.
- Kirubakaran, R., R. Kaliyamoorthy, R. Munusamy, and B. Annamalai. 2023. “Mechanical and Vibration Behavior of Surface‐Modified Titanium Sheet Interleaved with Woven Basalt/Flax Fiber Metal Laminates.” Polymer Composites 44 (12): 8442–8453. https://doi.org/10.1002/pc.27709.
- Kirubakaran, R., K. Rajkumar, and M. Rajesh. 2022. “Influencing Behavior Study of Natural Almond Shell Filler on the Tensile, Thermal, and Free Vibrational Properties of Flax Fiber Intertwined Vinyl Ester Composites.” Journal of Natural Fibers 19 (16): 12959–12970. https://doi.org/10.1080/15440478.2022.2080787.
- Mohanavel, V., S. Suresh Kumar, J. Vairamuthu, P. Ganeshan, and B. NagarajaGanesh. 2021. “Influence of Stacking Sequence and Fiber Content on the Mechanical Properties of Natural and Synthetic Fibers Reinforced Penta-Layered Hybrid Composites.” Journal of Natural Fibers 19 (13): 5258–5270. https://doi.org/10.1080/15440478.2021.1875368.
- Palanikumar, K., and V. Subbiah. 2019. “Bio Caryota Fiber Reinforced Polymer Composites: Mechanical Properties and Vibration Behavior Analysis.” Journal of Bionic Engineering 16 (3): 480–491. https://doi.org/10.1007/s42235-019-0039-y.
- Pothan, L. A., C. N. George, M. J. John, and S. Thomas. 2010. “Dynamic Mechanical and Dielectric Behavior of Banana-Glass Hybrid Fiber Reinforced Polyester Composites.” Journal of Reinforced Plastics and Composites 29 (8): 1131–1145. https://doi.org/10.1177/0731684409103075.
- Prabhu, P., B. Karthikeyan, R. Ravi Raja Malar Vannan, and A. Balaji. 2021. “Dynamic Mechanical Analysis of Silk and Glass (S/G/S)/Pineapple and Glass (P/G/P)/Flax and Glass (F/G/F) Reinforced Lannea Coromandelica Blender Hybrid Nano Composites.” Journal of Materials Research and Technology 15:2484–2496. https://doi.org/10.1016/j.jmrt.2021.09.068.
- Rajeshkumar, G., and V. Hariharan. 2014. “Free Vibration Characteristics of Phoenix Sp Fiber Reinforced Polymer Matrix Composite Beams.” Procedia Engineering 97:687–693. https://doi.org/10.1016/j.proeng.2014.12.298.
- Ramesh, M., K. Palanikumar, and K. H. Reddy. 2017. “Plant Fibre Based Bio-Composites: Sustainable and Renewable Green Materials.” Renewable and Sustainable Energy Reviews 79:558–584. https://doi.org/10.1016/j.rser.2017.05.094.
- Ramraji, K., K. Rajkumar, and P. Sabarinathan. 2020. “Mechanical and Free Vibration Properties of Skin and Core Designed Basalt Woven Intertwined with Flax Layered Polymeric Laminates.” Proceedings of the Institution of Mechanical Engineers, Part C: Journal of Mechanical Engineering Science 234 (22): 4505–4519. https://doi.org/10.1177/0954406220922257.
- Selvaraj, G., R. Kaliyamoorthy, and R. Kirubakaran. 2022. “Mechanical, Thermogravimetric, and Dynamic Mechanical Analysis of Basalt and Flax Fibers Intertwined Vinyl Ester Polymer Composites.” Polymer Composites 43 (4): 2196–2207. https://doi.org/10.1002/pc.26533.
- Shi, J., W. Zhang, G. Shaohua, S. Yuan, and J. Zhang. 2023. “Physical and Mechanical Properties of Bamboo Fiber/Glass Fiber Mesh Reinforced Epoxy Resin Hybrid Composites: Effect of Fiber Stacking Sequence.” Journal of Natural Fibers 20 (1): 1. https://doi.org/10.1080/15440478.2023.2167145.
- Sreenivasan, V. S., N. Rajini, A. Alavudeen, and V. Arumugaprabu. 2015. “Dynamic Mechanical and Thermo-Gravimetric Analysis of Sansevieria Cylindrica/Polyester Composite: Effect of Fiber Length, Fiber Loading and Chemical Treatment.” Composites Part B: Engineering 69:76–86. https://doi.org/10.1016/j.compositesb.2014.09.025.
- Yosr, B. M., J. Mounir, K. Foued, M. Slah, and B. Durand. 2022. “Characterization and Investigation of Tensile Properties of Kenaf Fiber Reinforced Polyester Composite Material.” Journal of Natural Fibers 19 (14): 8355–8366. https://doi.org/10.1080/15440478.2021.1964128.