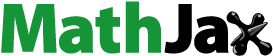
ABSTRACT
In this work, dyed samples were self-prepared from two methods including madder dyeing and mordant dyeing by madder combined with Al3+ at different temperatures, and their kinetics and isothermal adsorption were both discussed. Weber–Morris diffusion model and Freundlich model were found to be more suitable in dyeing for kinetics and thermodynamics. Through SEM, FTIR and fastness tests, the dyeing performances were displayed and the possible structure between madder dyes and the complex with Al3+ were illustrated. By molecular docking, madder dyes showed combination with glutamic acid predominantly. After introducing metal ion, the shortage of hydrogen bonds length was observed. It was initially employed to elucidate the potential binding modes between dyes and proteinaceous fibers. Overall, through experimental dyeing and curve fitting, this study provided insights into the dyeing mechanism of madder-associated dyestuffs on silk. Moreover, molecular docking elucidated the dyeing mechanism further, highlighting that the presence of metal ion enhances the firmness of the staining. The elucidation of dyeing mechanism not only provided valuable theoretical support for color restoration but also shed light on the practically modify of methods in obtaining ideal color. These results could serve as inspiration for conservators to maintain the vibrancy and longevity of dyed silks.
摘要
本工作采用茜草染色和茜草与Al3+在不同温度下进行媒染剂染色两种方法制备了染色样品,并对其动力学和等温吸附进行了讨论. Weber-Morris扩散模型和Freundlich模型在动力学和热力学方面更适合染色. 通过SEM、FTIR和牢度测试,展示了茜草染料的染色性能,并说明了茜草与Al3+配合物之间可能的结构. 通过分子对接,茜草染料主要与谷氨酸结合. 引入金属离子后,观察到氢键长度不足. 它最初用于阐明染料和蛋白质纤维之间的潜在结合模式. 总之,通过实验染色和曲线拟合,本研究深入了解了茜草类染料在丝绸上的染色机理. 此外,分子对接进一步阐明了染色机制,强调了金属离子的存在增强了染色的牢固性. 对染色机理的阐明,不仅为色彩恢复提供了宝贵的理论支持,而且也为实际改进获得理想色彩的方法提供了参考. 这些结果可以为保护人员保持染色丝绸的活力和寿命提供启发.
Introduction
Natural dyes have gradually won people’s attention in industries such as textiles and fashion since that aligns with the sustainable development goals established by the United Nation. They offer an environmentally friendly alternative to synthetic dyes, contributing to sustainable production practices. Madder (Rubia tinctorum L.) is a plant-based source widely used throughout history for its rich vibrant red color properties (Jahangiri et al. Citation2018) and good antibacterial activity (Güzel, Karadag, and Alkan Citation2020). Meanwhile, madder has been frequently preferred in textile coloring since it supports production with zero discharge of hazardous chemicals, which means that it can bring greater environmental price benefits through chemical pollution reduced (Nimra et al. Citation2023). Furthermore, this type of production would improve environmental standards and gain a competitive advantage in global markets by producing “green products” (Nimkar Citation2018). Based on that, the economic factor of madder shows great potential due to its properties of easy cultivation, less water usage in textile field, and further creation of employment (Ozdemir and Karadag Citation2022). When textiles are immersed into a madder dye bath, main dyestuff alizarin is into the fibers through diffusion and adsorption processes. The molecule then forms strong bonds with the fiber structure through hydrogen bonding or covalent interactions, and further understanding the mechanisms behind using natural sources like madder for fabric dyeing contributes positively toward achieving SDG targets related to sustainable consumption patterns and environmental protection within textile industries globally.
While the majority of such efforts center on recreating colors that match those in ancient records (Chahardoli, Berghe, and Mazzeo Citation2019; Li et al. Citation2020; Luca et al. Citation2017) including T’ien-kung k’ai-wu (Song Yingxing, the Ming Dynasty, AD 1368–1644), Qimin Yaoshu (Jia Sixie, the Northern Wei Dynasty, AD 533–544) and so on. Besides, researchers focus on studying dyed textiles made from wool, cotton products and other natural plants in order to gain other insight (Bukhari et al. Citation2023; Ltaief et al. Citation2023; Rym, Soufien, and Faouzi Citation2020) rather than investigating the dyeing mechanism and modifying staining parameters to obtain ideal colors of madder. Undertaking dyeing experiments without a clear understanding of the underlying mechanisms would inevitably result in increased consumption of energy and materials. Therefore, gaining a thorough understanding of the dyeing process is crucial to achieving optimal coloration. The process of dyeing could be described as the adsorption of dye molecules onto the surface of protein textiles, and the underlying adsorption kinetic and thermodynamic principles could be employed to elucidate this process further, which could aid researchers in achieving a more profound and comprehensive understanding of that phenomenon.
Molecular docking is a well-established method used to study and predict binding interactions between a macromolecule acting as the receptor such as DNA, protein, enzyme and so on, and a small molecule or another macromolecule as a ligand. In the docking process, the spatial and energy matching between the receptor and the ligand would be matched to obtain the best conformation, especially affinity. During the process of ligand and receptor binding, the binding site changes continuously until a stable binding is successfully established (Roche, Brackenridge, and McGuffin Citation2015). This method has been extensively applied in the field of drug design (Chavan and Das Citation2023; Sledz and Caflisch Citation2018) and provides valuable information regarding the various interaction forces involved, such as hydrogen bonding (Heidarian and Zahedi-Tabrizi Citation2021; Okpareke et al. Citation2020), hydrophobic effects (Hou et al. Citation2017; Yang et al. Citation2018), and salt bridges (Chellasamy and Watson Citation2022; Vasanthakumari, Pushpa, and Manoj Citation2019). These interactions could help researchers investigate more clearly the form of molecular binding at the microscopic level, and the strength of these interactions are crucial for assessing the affinity between the receptor and the ligand. Based on the form and strength of the interaction, it is possible to develop molecules that bind in a particular interaction. While in the field of textile and dyeing, there is not enough explanation of the mechanism of silk dyeing. For dyeing, small dye molecules could be used as ligands, and silk proteins could be used as receptors. During the dyeing process, pigment molecules will be impregnated onto silk proteins, but the binding site and the interaction force between the two are not clear. Molecular docking technology could help to explain the mechanism at the micro level and to elucidate the potential binding patterns between dye molecules and polymers. Applying this technology in the field of textiles could further provide evidence for the principle of dyeing adsorption from a theoretical perspective. It could also open up new research avenues to investigate the reasons for the better fastness of samples obtained through metal dyeing processes.
In this work, raw silks were dyeing with madder water extraction and mordant with Al3+, which is a commonly used metal mordant agent in dyeing as the format of KAl(SO4)2·12 H2O and also used without any restriction aligning with GOTS and NODS standards (Kakuee et al. Citation2019; Karadag Citation2023). Then the dyeing rate curves, color difference, kinetic and isothermal adsorption at various temperatures were investigated. Additionally, various models were tested to describe the dyeing process. Apart from that, SEM and FTIR were used to illustrate dyeing performance and possible structure change toward dye molecules, and fastness tests were employed to illustrate the dyeing effect from different dyeing methods. Moreover, the method of molecular docking was utilized to provide theoretical evidence for the potential binding pattern between dye molecules and protein fibers. This approach not only verified the applicability of the fitted model, but also provided additional structural information that shed light on the possible mechanism of dyeing adsorption at the molecular level.
Experimental
Materials
Madder (Rubia tinctorum L.) were purchased from Lei Yunshang Pharmaceutical Group Co., Ltd, Shanghai, China. Raw silks were supplied from Cultural Relics Protection Technology Centre of Shanghai Museum. Other experimental materials were acquired commercially including Aluminum potassium sulfate dodecahydrate Kalinite (KAl(SO4)2·12 H2O ≥ 99.5%, Shanghai Titan Scientific Co., Ltd, China), alizarin (≥98%, Shanghai yuanye Bio-Technology Co., Ltd), purpurin (≥98%, Shanghai Macklin Biochemical Technology Co., Ltd) and ultrapure water (HHitech Co., Ltd, China). All materials comply with GOTS and NODS standards.
Spectral studies
In the experiment of UV-Vis, 12.0 g madder was added into ultrapure water of 600 mL, heated at 90°C for 30 minutes to obtain madder extraction containing dye molecules. After filtration, the maximum wavelength was recognized through UV-Vis of madder extraction. Then, the extraction was diluted in various concentrations (0.01, 0.0067, 0.005, 0.004, 0.0033 g/mL) to record the absorbance through UV-VIS spectrophotometer, and the calibration curve was constructed using dye solution with different concentrations versus the absorbance of dye solution recorded at maximum wavelength.
Fourier transform infrared spectroscopy (FTIR) of alizarin, purpurin and the complex of those with Al3+, raw silk, madder dyed silk, mordant dyed silk was recorded to indicate the structure change of alizarin after combining with Al3+ by Nicolet iS50 FTIR spectrophotometer ranging from 2000 to 500 cm−1. All samples were grinding with KBr, which was used as internal standard.
Dyeing
66 g madder was added into 3.3 L water at 90°C to extract the red dye, then dye stock solution was obtained after filtration. Raw silks were soaked into dye stock solution directly without other treatments, and madder-dyed samples were obtained after rinsing with tap water to remove superfluous dye molecules and drying in the air. That process just dyeing with madder extraction was defined as madder dyeing, and sample got from this process was named as madder dyed sample. After adding 1% KAl(SO4)2·12 H2O into madder extraction, the dyeing bath containing madder combined Al3+ was obtained. Similarly, raw silks were immersed into solution to obtain mordant dyed samples and they were also rinsed and dried. Then the process just dyeing in solution containing madder combined with Al3+ was defined as mordant dyeing, and sample got from this process was names as mordant dyed sample.
Prepare of Alizarin-Al3+, Purpurin-Al3+
Alizarin and purpurin were, respectively, dissolved in hot water, and each 1 mL was taken and added to the petri dish by drops. Then 1 mL of aqueous solution containing 1% KAl(SO4)2·12 H2O was each added to those three colored solution in the petri dish by drops. Then, two drops were mixed evenly with a glass rod. Subsequently, the petri dish with mixture was put into the oven at 45°C to vaporize. The solid complex of Alizarin-Al3+ and purprin-Al3+ were remained in the petri dish to further test.
Kinetic studies and adsorption isotherm
For kinetic study, raw silks were cut into 66 pieces (each about 10 mg) for three groups. Each group of silks was soaked in the 50 mL madder extraction and madder-Al3+ solution with the same initial concentration (C0 = 0.02 g/mL) and dyed at 60°C, 75°C and 90°C for 5, 10, 20, 30, 45, 60, 75, 120, 180, 210 and 240 minutes, respectively. Furthermore, the raffinate’s absorbance (absorbance of the residual dyeing solution) and the adsorptive capacity of dyestuff on silks were calculated as below (Jiwalak et al. Citation2010):
Where qt (g/mg) represents the quantity of adsorption capacity of unit mass silk; C0 (g/mL) and Ct (g/mL) are the initial and final concentrations of dye in the solution, respectively; V (mL) is volume of dye bath; and M (mg) is weight of fabric.
Then, pseudo first-order model, pseudo second-order model and Weber–Morris interior diffusion model were fitted. The equations of the three models are as follows:
Pseudo first-order model (Balikram and Ojha Citation2016):
Pseudo second-order model (Salvestrini Citation2018):
Weber–Morris interior diffusion model (Baldermann and Stamm Citation2022):
Where k is the constant of each kinetic reaction rate model, qe is the equilibrium adsorption capacity for each model; C is the adsorption constant of the Weber–Morris interior diffusion model; t is time.
For adsorption isotherm study, dye solutions were prepared in five different concentrations (0.02, 0.012, 0.01, 0.005, 0.0025 g/mL). Three different groups of these solutions (50 mL according to liquor ratio 1:5 for 10 mg sample) were made and each group was conducted at desired temperatures of 60°C for the first set, 75°C for the second set, and 90°C for the third set of solutions. Raw silks were dipped in each 50 mL dyeing solution. After 1 h, the dyed samples were taken out and the raffinate’s absorbance (absorbance of the residual dyeing solution) and adsorptive capacity of dyestuff on silks were calculated.
Three adsorption isotherm models, namely Nernst, Langmuir, and Freundlich models, were fitted. The equations for these three models are as follows:
Nernst model (Sawada Citation2004):
Langmuir model (Revellame et al. Citation2020):
Freundlich model (Khayyun and MseerCitation2019):
where is the equilibrium constant concentrations; k is the adsorption constants for each model;
is the maximum equilibrium adsorption capacity calculated by the Langmuir model; and n is the heterogeneous factor for Freundlich model.
Color difference measurement for dyed samples
The current study aimed to investigate the dyeing effects on dyed samples and raw silks, which were evaluated using colorimetric parameters in the CIE (Commission Internationale de l´Eclairage) 1976 Lab* Uniform Color Space. The color differences (ΔEab*) were calculated according to equation: (2–1), where L* represents the lightness, a* represents the chroma ranging from red to dark green with positive values indicating redness, and b* represents the chroma ranging from yellow to blue with positive values indicating yellowness.
Fastness tests for madder dyed sample and mordant dyed sample
The rubbing fastness of all dyed samples underwent testing by affixing the fabric onto a panel and subjecting it to 10 strokes for both dry and wet rub fastness assessments in accordance with the GB/T 3920–2008 standard. Evaluations for washing fastness with soap involved mechanical mixing, followed by the measurement of stains on adjacent white cotton fabric following the guidelines outlined in GB/T 3921–2008. Additionally, assessments for fastness to light-induced yellowing were conducted by exposing the samples to a lamp emitting UV-A340 light for 6 hours, adhering to the specifications of GB/T 30,669–2014. The color fastness of the dyed samples was tested according to Chinese Textiles Test Specification based on ISO international standards (National Standard of the People’s Republic of China Citation2008a, Citation2008b, Citation2014).
Molecular docking and statistics analyzing
Alizarin and purpurin, the selected ligands, are prominent constituents of madder, and these chemical structures represent a typical profile of the madder family (Williams Citation2022). The corresponding macromolecule was retrieved from PDB database with code 3UA0, which corresponds to protein fibril (He et al. Citation2012). The present study employed AutoDock 4.2 to conduct in simulation of potential binding modes between silk and dyeing molecule (Morris et al. Citation2009). Curve fitting analysis was conducted using MATLAB R2022a, where the coefficient of determination (R2) was utilized to quantify the level of goodness-of-fit.
Instruments
The JE703C electronic balance (Mettler Toledo Co., Ltd, Swiss) was used for weighting different materials. The 1900i ultraviolet-visible (UV-Vis) spectrophotometer (Shimadzu Co., Ltd, Japan) was utilized to measure the absorbance values. The Nicolet iS50 FTIR (Thermo Fisher Scientific Co., Ltd, USA) was for characterizing the structure of alizarin, purpurin and combination of those with Al3+. The YS3060 Colorimeter (Shenzhen 3NH Technology Co., Ltd, China) was applied to measure color differences for all samples. The Scanning Electron Microscope (SEM, S-3400N, Hitachi, Ltd, Japan) was assessed for characterizing the staining results of the dyed samples.
Results and discussion
Calibration curve through UV-Vis spectrometry
The full scan spectrum showed only one peak in the region of visible light although the extraction of madder was a mixture solution, which meant all compounds in madder extraction solution shared similar characteristic groups and chemical properties. Therefore, mixed solution for dyeing might reflect the practical dyeing mechanism and the extraction was not further purified. Then, the maximum absorbance wavelength was achieved at 430 nm in Figure S1, which was consistent with previous study (Cuoco et al. Citation2009). The linear regression equation of the madder solution was also depicted, and its correlation coefficient R2 of the regression equation was found to be 0.9978, indicating a high degree of fitting. This implied that the obtained curve satisfactorily explained the relationship between the concentration of the madder solution and its corresponding absorbance. It was thus inferred that the proposed equation (y = 110.68×+0.1317) could be used to determine the concentration of the madder solution via absorbance measurements with a good degree of accuracy.
Study of kinetics and isothermal adsorption during dyeing
For dyeing rate curves, both curves of madder dyeing and mordant dyeing increased sharply at early stage owing to the quickly combination with molecule and silk as shown in . Moreover, the adsorption capacity under mordant dyeing was always greater than that under madder dyeing, which might be due to the complex formation between mordant Al3+, dyestuffs and fibers. The formation of complexes made it easier for more dye molecules to remain on the silk surface in the form of ligands, further increased the adsorption capacity (Bukhari et al. Citation2017). After 75 min, curves were still slowly rose while the gaps of quantity between different temperature were gradually obvious at the same time, which indicated the higher temperature led to higher swelling degree of silks, causing more combination positions on silk proteins exposed to dye molecules, helping molecules diffuse into the interior of fibers and to be adsorbed. As for the color difference in , about 180 min later, the ΔEab* was depicted a slight fluctuation, and the maximum ΔEab* of dyed samples with different methods was obtained at the final minute. At 90°C under two methods, ΔEab* was basically higher than that at other temperatures, which further presented the temperature played a significant role in dyeing process. Although the quantity of molecule was slowly increasing after that, the trend of color difference change was not positively correlated with adsorption capacity, which could be explained that the color difference of samples was jointly determined by squares of three factors: ΔL*, Δa*, Δb*, meanwhile their data were not showing a clear monotonically increasing trend in Table S1.
Figure 1. Scatter diagram of kinetic dyeing in silk: a) dyeing point at different temperatures and methods; b) color difference at different temperatures and methods.

The model fitting curves at 60, 75, and 90°C under madder dyeing and mordant dyeing were shown in , respectively. Models of pseudo first order, pseudo second order and Weber–Morris diffusion were applied to illustrate the adsorption behavior toward dyeing molecule and silk. In , it can be seen that R2 (Weber–Morris diffusion) > R2 (pseudo second order) > R2 (pseudo first order) in both methods, which indicated that the staining process was accord more with the Weber–Morris internal diffusion model (Jiang et al. Citation2020; Pandiarajan et al. Citation2018), while completely did not comply with pseudo first-order and pseudo second-order kinetic model. Furthermore, that presented the intermolecular pattern between the dye molecules and the silk fiber was a continuous process, dye molecules first adhered to the silk surface, at the moment, the liquid film diffusion resistance formed on the silk surface only works for a short time, and then gradually diffused to the inside of the silk randomly with the extension of time, and finally reached equilibrium (Leamkaew et al. Citation2022).
Figure 2. Kinetic models of dyeing in silk: (a) fitting curve of madder dyeing at 60°C; (b) fitting curve of madder dyeing at 75°C; (c) fitting curve of madder dyeing at 90°C; (d) fitting curve of mordant dyeing at 60°C; (e) fitting curve of mordant dyeing at 75°C; (f) fitting curve of mordant dyeing at 90°C.

Table 1. (і) Parameter values for dyeing rate curve fitting models of madder dyeing (іі) Parameter values for dyeing rate curve fitting models of mordant dyeing
The adsorption isotherms of silk dyeing with madder dyeing and mordant dyeing were shown in . At relatively low concentrations region, the equilibrium adsorption capacity demonstrated little variation at different temperatures, which was more obvious under madder dyeing. Subsequently, with further increasing concentration, the capacity at 60°C was significantly lower compared to that at 75°C and 90°C. Under madder dyeing, the difference in capacity between 75°C and 90°C was mildly pronounced, while under mordant dyeing, that difference was rapidly grown with concentration increase. It could be inferred that mordant molecules are more likely to enter silk at larger concentrations and temperatures. Overall, the equilibrium adsorption capacity boosted rapidly at the different temperatures meanwhile its rate gradually increased with the addition of dye concentration. As shown in , the results indicated that under isothermal adsorption conditions, the ΔEab* increased with rising equilibrium concentration, and the curves displayed nearly similar trends toward two different methods. In the whole process, the color difference at 90°C was consistently the highest value, followed by that at 75°C and 60°C. At the condition of same maximum equilibrium concentration while different temperature, the ΔEab* was basically the same, respectively.
Figure 3. Scatter diagram of isothermal dyeing in silk: (a) dyeing point at different concentration and methods; (b) color difference at different concentration and methods.

Then, adsorption isotherms were fitted using three different models, namely Nernst, Langmuir, and Freundlich, as depicted in . For madder dyeing fitting, the R2 values in (ⅰ) indicated that the process was more suitable for Freundlich model. For mordant dyeing, the R2 values obtained for the Nerst, Langmuir, and Freundlich models were very closer across varying temperatures while Freundlich model were found to be more applicable due to little higher than other R2 values, as evidenced in (ⅱ). These results indicated that the Freundlich model was more suitable for accurately describing the adsorption isotherm of dye molecules onto silk substrate, which meant that process was a multimolecular layer adsorption (Xu et al. Citation2012). At high concentrations, the equilibrium adsorption capacity increased which was consistent with the Freundlich model. Madder dyes were found to be coplanar and exhibited good affinity toward silk protein and hydrogen bonds could be formed with hydroxyl groups of amino acids, which was a property of non-localized adsorption (Jiang et al. Citation2020). Notably, this non-localized adsorption pattern demonstrated some similarity with the Langmuir model, that is because the non-localized adsorption model assumes the adsorption at the first layer is finite, and the Langmuir model also assumes that the adsorption at the solid surface is restricted. According to the equation (3–1), the calculated
was range from 0 to −20 kJ/mol, which indicated that process was physical adsorption (Wu, Luo, and Wang Citation2014) and referred to the non-covalent bonding force such as van der Waals force and hydrogen bond between dye molecules and silk surfaces.
Figure 4. Adsorption isotherms models of dyeing in silk: (a) fitting curve of madder dyeing at 60°C; (b) fitting curve of madder dyeing at 75°C; (c) fitting curve of madder dyeing at 90°C; (d) fitting curve of mordant dyeing at 60°C; (e) fitting curve of mordant dyeing at 75°C; (f) fitting curve of mordant dyeing at 90°C.

Table 2. (ⅰ) Parameter values for adsorption isotherm models of madder dyeing (ⅱ) Parameter values for adsorption isotherm models of mordant dyeing.
SEM of dyed samples and FTIR of dye molecules
The SEM of raw silk, madder dyeing sample and mordant dyeing sample displayed different performance as depict in . After dyeing, the interval between silk fibers were narrowed, which might be due to the swelling of single fiber of silk in the aqueous solution during the dyeing process. During SEM imaging, white and bright areas were lightly visible because of very poor electrical conductivity of silk, which led to charge store up and electrons were accumulated on the surface. After madder dyeing, the area of bright spots was reduced, which might be due to the presence of madder dye in partial ionic form on the silk surface. When mordant by Al3+, this phenomenon of bright spots was ameliorated with increasing dark areas, which demonstrated the metal cation was successfully stand on so that electrical conductivity of dyed sample was improved compared with madder dyeing samples.
Figure 5. SEM images of silk and dyed samples: (ⅰ) raw silk, (ⅱ) madder dyeing sample, (ⅲ) mordant dyeing sample; (b) FTIR of raw silk, madder dyed silk and mordant dyed silk.; (c) FTIR of alizarin and alizarin-Al3+ complex; (d) FTIR of purpurin and purpurin-Al3+ complex.

Raw silk, madder dyed silk and mordant dyed silk were shown in , and peak shape of the three samples was very similar. The C-O stretching vibration band appeared between 1630 and 1670 cm−1, probably overlapped with N-H bending in amide I band. The infrared curve of raw silk showed a peak at 1632 cm−1, but it disappeared in the dyed sample, which indicated that the amino or carbonyl group in the silk was involved in the dyeing process. It was seen that there was slight alteration of intensity and shift of peaks in C-N stretch of amide III bands at 1238 cm−1 and 1081 cm−1, which indicated the involvement of amine groups in the interaction between fiber, mordant, and dye molecules. Meanwhile, the sample mordanted with Al3+ showed apparent shifting of peaks with C-N stretch at 1156 cm−1, which was attributed due to the formation of complex between metal ions and silk fiber.
Similarly, considered that the compounds alizarin and purpurin were the main component in madder (Jiang et al. Citation2021), it together with the mordant complex were both characterized by FTIR to determine whether there were any structure changes. As shown in , in the spectrum of alizarin, 712 cm−1 was the out-of-plane bending vibration of C-H; 1295 cm−1 and 1198 cm−1 were C-O stretching vibration; 1349 cm−1and 1330 cm−1were in plane bending vibration of O-H; 1589 cm−1and 1454 cm−1were vibration of benzene skeleton, and 1664 cm−1, 1631 cm−1 were the stretching vibration of carbonyl group, while former was free carbonyl, another formed a intramolecular hydrogen bond with hydroxyl group as associative carbonyl to make frequency decreased. However, in the complex of alizarin and Al3+, the 1664 cm−1 was disappeared, which indicated that Al3+ was combined with free carbonyl of alizarin. depicted purpurin and purpurin-Al3+ with closely outlines to those of alizarin and its complex. Based on the fundamental anthraquinone structure, both 1652 cm−1 and 1621 cm−1 peaks corresponded to carbonyl group vibrations. A red shift was observed in comparison to the wave number of carbonyl groups in alizarin, which was attributed to the presence of a hydroxyl group at C4 of the anthraquinone ring. Upon combining with Al3+, the disappearance of the 1652 cm−1 peak indicated the binding of Al3+ with the carbonyl group of purpurin. The presence of carbonyl and hydroxyl groups in the rings of alizarin and purpurin, rich in electrons, facilitated electron transfer, making them prone to forming coordination bonds with metal ions.
Fastness properties of madder dyed sample and mordant dyed sample
The color fastness values of silk samples with madder dyeing and mordant dyeing were presented in . The color fastness to dry rubbing for both samples proved higher than that of wet rubbing, indicating potential shedding of dye molecules from silk surface in moist environment. The mordant dyed sample achieved level 5 in dry rubbing and level 4–5 in wet rubbing, while the fastness for the madder dyed sample arranged in level 4–5 in dry rubbing and were at level 4 in wet rubbing. Despite both samples undergoing the same test condition, the color fastness of the mordant dyed sample was only one level higher than that of the madder dyeing sample. This suggested the enhancement of fiber color fastness due to the presence of the metal mordant. Similarly, regarding the color fastness of dyed samples to washing and light yellowing, the madder dyed sample achieved level 4–5, while the mordant dyed sample attained level 4–5 and level 5. Typically, the color fastness of the mordant dyed sample was usually one step higher than that of the madder-dyed sample. Furthermore, the color staining fastness on cotton of dyed samples with a metal mordant to washing was higher than color change fastness, implying the potential release of dye molecules from the silk into the soap water. This observation suggested that water and chemical washing substances were not favor of retaining color in silks. However, on a positive note, the fastness of all samples was above level 4, indicating that madder showed promising potential for dyeing, whether using a mordant or not. (The rating scale was as follows: 1, very poor; 2, poor; 3, moderate; 4, good; 5, excellent)
Table 3. Color fastness of madder dyed silk and mordant dyed silk.
Molecular docking between dye molecules and silk protein
Molecular docking could be employed to simulation the potential binding patterns between macromolecule and ligand, which may involve the formation of hydrogen bonds, π-π interactions and π-cation interactions. As shown in , alizarin and purpurin acted as ligands forming two hydrogen bonds with residues of protein, respectively, which was produced by the bonding of hydrogen atoms with more electronegative atoms, such as oxygen in amino acids. Besides, there were Van der Waals force, Pi-Sigma force between amino acid and anthraquinone ring structure of alizarin, and since the existing of Pi-Sigma, it made the structure more stable and easier to form. Specifically, both hydrogen bonds with alizarin were formed between hydroxyl group of the small molecule and carbonyl group of Glu94 in chain B of silk protein, and distances toward these hydrogen bonds were measured to be 2.1 Å and 1.8 Å, respectively, which indicated that alizarin had a certain affinity with glutamic acid, and alizarin in the dyeing might bind easily with glutamic acid on the silk protein surface. As for purpurin combined with protein, two hydrogen bonds were formed in Glu94 in chain A with distances of 2.2 Å and 2.0 Å, which also indicated that purpurin could combine silk protein closely.
Figure 6. Possible binding configurations of madder and protein (PDB number: 3UA0) through molecule docking: (a) dyes combine with protein: (i) alizarin, (ii) purpurin; (b) complex combine with protein: (i) alizarin-Al3+, (ii) purpurin-Al3+ .

Moreover, Van der Waals force in simulation further verified physical adsorption in the dyeing process (Zhang et al. Citation2015), which was common in physical adsorption processes. The emergence of Pi-sigma force was based on small dye molecules containing π orbitals such as alizarin, which was more prone to conjugation through reducing the energy of the substance and stabilizing the structure (Shen et al. Citation2021), which could help the formation of the substance and the process of dyeing adsorption. Several interactions, such as Pi-Alkyl, Pi-Anion, and Pi hydrophobic, were observed in the combination of purpurin and protein, playing a role in stabilizing the structure. Pi-alkyl interaction involved the π-electron cloud of an aromatic group interacting with electron groups of alkyl groups found in amino acids (Clemilson et al. Citation2018). Pi-Anion interaction related to electrostatic forces and ion-induced polarization, particularly within aromatic rings, contributing to structural stabilization (Kan et al. Citation2018). Pi hydrophobic interaction involved the concentration of nonpolar atoms or groups, further enhancing structural stability (György and Miklós Citation2022).
However, when metal mordants were bonded to the carbonyl oxygen of alizarin and purpurin as shown in , the potential binding position was altered through molecular docking. The position of complex tended to combine with Asp89 in chain B for alizarin-Al3+. For purpurin-Al3+combination with protein, the complex showed a tendency to bind with Gln65 and Glu28 in chain B. These results suggested that the introduction of metal mordants would alter the binding patterns between molecules and protein in comparison with that without mordants. Additionally, the analysis showed a decrease in the number of hydrogen bonds to one between the molecule and Asp89 in the alizarin-Al3+ complex, while the purpurin-Al3+ complex displayed an increase to three bonds. That indicated the role of metal mordants in modifying the binding patterns between dye molecules and protein. This was likely due to the coordination geometries and charge densities of the metal ions, which led to different binding preference and strength (Grazia et al. Citation2011). Apart from that, the distance of hydrogen bond between ligand and Asp89 was 1.6 Å upon metal mordant binding, which indicated a stronger bond was formed. Compared with the structure of alizarin-protein, the hydrogen bond length was shortened by 0.2–0.5 Å, which indicated that if the mordant sample and the dyed sample were rubbed to achieve the same degree, the mordant sample needed more energy to break the hydrogen bond with more severe external condition such as stronger light, stronger friction, and so on. In other words, under the same fastness test condition, the performance of the mordant sample would show better than that with the dyed sample. The reduction in bond length and increase in bond energy was likely to contribute to the increased fastness of the mordant dyed samples.
Conclusion
In this study, the assessment of color difference and adsorption capacity toward madder dyeing and mordant dyeing with raw silk presented the greatest disparity under elevated temperatures, signifying the prominent influence of temperature in the dyeing procedure. The Weber–Morris diffusion kinetic model was appropriate for characterizing the dyeing rate behavior in the processes of madder dyeing and mordant dyeing. Calculated thermodynamic parameter ΔG0 revealed that both dyeing processes were physical adsorption and the Freundlich model was proved to be superior in characterizing the isothermal adsorption. Meanwhile, SEM was applied to character dyeing performance for madder dyed sample and mordant dyed sample, which displayed the change of gap between silk fibers and conductivity property. Subsequently, FTIR analysis was used to illustrate the possible structural change between alizarin, purpurin and the complex of those with Al3+, revealing the free carboxyl group was combined with Al3+. From the point of view of fastness, the metal mordant Al3+ improves the color fastness of the sample. Then from the simulation perspective, the results of molecular docking provided a potential binding mode between dyeing substances and protein fibers. Simultaneously, the hydrogen bonds, Van der Waals force were observed between alizarin, purpurin, complex and protein, which further confirmed the validity of the adsorption model fitting. Following the introduction of metal ion, results offered theoretical evidence that metal has the ability to alter the binding patterns and metal might enhance the binding affinity between dye molecules and protein fibers.
Elucidating the process of dyeing adsorption from kinetic and thermodynamic perspectives could aid researchers in understanding the mechanisms of dyeing. Molecular docking also offers a novel approach for verifying suitable combination patterns for protein textiles. Besides, our proposed hypothesis requires further experimentation and evidence to support it, which also provides a clear direction for our future research.
Highlights
Weber–Morris diffusion model was suitable with higher R2 for adsorption kinetics toward madder dyeing with madder extraction and mordant dyeing with Al3+ on silk.
Freundlich model was applicable with higher R2 for isothermal adsorption of madder dyeing with madder extraction and mordant dyeing with Al3+ on silk.
Molecular docking was initially employed to elucidate the potential binding modes between dyes and protein fiber.
Glutamic acid showed predominant combination trend with madder-associated dyestuffs. After introducing Al3+, the length of hydrogen bonds was shortened, which might be cause of improvement of fastness with mordant dyed samples.
Author contributions
Y.J. Guo and W.Q. Zhang conceived the conceptualization. W.Q. Zhang provided all the resources. Y.J. Guo conducted the dyes extraction, dyeing process and color differences measuring and characterize of SEM. Y.J. Guo conducted the molecular docking dependently. Y.J. Guo and X.D. Zhou interpreted the data. Y.J. Guo and X.D. Zhou drew all figures. Y.J. Guo wrote the original draft. M.Q. Wu, L.F. Zhang, X.Y. Luo as well as W.Q. Zhang co-wrote the paper in review and editing. All authors had given approval to the final version of the manuscript.
Acknowledgments
The authors thanked the Research Centre of Analysis and Test of East China University of Science and Technology for help on data and characterization.
Disclosure statement
No potential conflict of interest was reported by the author(s).
Additional information
Funding
References
- Baldermann, A., and F. M. Stamm. 2022. “Effect of Kinetics, pH, Aqueous Speciation and Presence of Ferrihydrite on Vanadium (V) Uptake by Allophanic and Smectitic Clays.” Chemical Geology 607:121022. https://doi.org/10.1016/j.chemgeo.2022.121022.
- Balikram, A., and K. Ojha. 2016. “Studies on Kinetics and Thermodynamics of Cr(vi) Adsorption Onto Sand to Check Feasibility of Its Use as Tracer in Oilfields.” Asia-Pacific Journal of Chemical Engineering 11 (6): 1060–16. https://doi.org/10.1002/apj.2046.
- Bukhari, M. N., S. Islam, M. Shabbir, L. J. Rather, M. Shahid, U. Singh, M. A. Khan, and F. Mohammad. 2017. “Dyeing Studies and Fastness Properties of Brown Naphtoquinone Colorant Extracted from Juglans Regia L on Natural Protein Fiber Using Different Metal Salt Mordants.” Textiles and Clothing Sustainability 3 (1): 3–11. https://doi.org/10.1186/s40689-016-0025-2.
- Bukhari, M. N., M. A. Wani, M. Fatima, J. S. Bukhari, M. Shabbir, L. Jameel, and R. F. Mohammad. 2023. “Dyeing of Wool with Sappan Wood Natural Dye Using Metal Salts for Enhancement in Color and Fastness Properties.” Journal of Natural Fibers 20 (2): 2208890. https://doi.org/10.1080/15440478.2023.2208890.
- Chahardoli, Z., I. V. Berghe, and R. Mazzeo. 2019. “Twentieth Century Iranian Carpets: Investigation of Red Dye Molecules and Study of Traditional Madder Dyeing Techniques.” Heritage Science 7 (1): 57–68. https://doi.org/10.1186/s40494-019-0288-4.
- Chavan, G., and D. Das. 2023. “Design and Characterizations of pH-Responsive Drug Delivery Vehicles Using Molecular Docking.” Materials Technology 38 (1): 2196490. https://doi.org/10.1080/10667857.2023.2196490.
- Chellasamy, S. K., and E. Watson. 2022. “Docking and Molecular Dynamics Studies of Human Ezrin Protein with a Modelled SARS-CoV-2 Endodomain and Their Interaction with Potential Invasion Inhibitors.” Journal of King Saud University – Science 34 (7): 102277. https://doi.org/10.1016/j.jksus.2022.102277.
- Clemilson, B. J., P. S. Ana, C. F. Andrea, B. G. Jones, P. C. Denise, S. Paula, C. R. Nelilma, and M. A. Leandro. 2018. “Unraveling Molecular Targets of Bisphenol a and S in the Thyroid Gland.” Environmental Science and Pollution Research 25 (27): 26916–26926. https://doi.org/10.1007/s11356-018-2419-y.
- Cuoco, G., C. Mathe, P. Archier, F. Chemat, and C. Vieillescazes. 2009. “A Multivariate Study of the Performance of an Ultrasound-Assisted Madder Dyes Extraction and Characterization by Liquid Chromatography-Photodiode Array Detection.” Ultrasonics Sonochemistry 16 (1): 75–82. https://doi.org/10.1016/j.ultsonch.2008.05.014.
- Grazia, C., C. Clementi, C. Miliani, and A. Romani. 2011. “Photophysical Properties of Alizarin and Purpurin Al(iii) Complexes in Solution and in Solid State.” Photochemical & Photobiological Sciences 10 (7): 1249–1254. https://doi.org/10.1039/c1pp05039g.
- Güzel, E. T., R. Karadag, and R. Alkan. 2020. “Durability, Antimicrobial Activity and HPLC Analysis of Dyed Silk Fabrics Using Madder and Gall Oak.” Journal of Natural Fibers 17 (11): 1654–1667. https://doi.org/10.1080/15440478.2019.1588827.
- György, G. F., and K. Miklós. 2022. “Contribution of Hydrophobic Interactions to Protein Mechanical Stability.” Computational and Structural Biotechnology Journal 20:1946–1956. https://doi.org/10.1016/j.csbj.2022.04.025.
- He, Y. X., N. N. Zhang, W. F. Li, N. Jia, B. Y. Chen, K. Zhou, J. H. Zhang, Y. X. Chen, and C. Z. Zhou. 2012. “N-Terminal Domain of Bombyx Mori Fibroin Mediates the Assembly of Silk in Response to pH Decrease.” Journal of Molecular Biology 418 (3–4): 197–207. https://doi.org/10.1016/j.jmb.2012.02.040.
- Heidarian, R., and M. Zahedi-Tabrizi. 2021. “Effect of the Intramolecular Hydrogen Bond on the Active Metabolite Analogs of Leflunomide for Blocking the Plasmodium falciparum Dihydroorotate Dehydrogenase Enzyme: QTAIM, NBO, and Docking Study.” Current Computer-Aided Drug Design 17 (3): 480–491. https://doi.org/10.2174/1573409916666200527133126.
- Hou, G. Y., R. Zhang, X. Y. Hao, and C. G. Liu. 2017. “An Exploration of the Effect and Interaction Mechanism of Bisphenol a on Waste Sludge Hydrolysis with Multi-Spectra, Isothermal Titration Microcalorimetry and Molecule Docking.” Journal of Hazardous Materials 333:32–41. https://doi.org/10.1016/j.jhazmat.2017.03.018.
- Jahangiri, A., S. M. Ghoreishian, A. Akbari, M. Norouzi, M. Ghasemi, M. Ghoreishian, and E. Shafiabadi. 2018. “Natural Dyeing of Wool by Madder (Rubia Tinctorum L.) Root Extract Using Tannin-Based Biomordants: Colorimetric, Fastness and Tensile Assay.” Fibers and Polymers 19 (10): 2139–2148. https://doi.org/10.1007/s12221-018-8069-3.
- Jiang, H. Y., X. D. Hu, A. Khan, J. B. Yao, and M. T. Hussain. 2020. “Dyeing Mechanism and Photodegradation Kinetics of Gardenia Yellow Natural Colorant.” Textile Research Journal 91 (7): 1–12. https://doi.org/10.1177/0040517520958483.
- Jiang, H. Y., X. D. Hu, J. J. Zhu, J. Wan, and J. B. Yao. 2021. “Studies on the Photofading of Alizarin, the Main Component of Madder.” Dyes and Pigments 185:108940. https://doi.org/10.1016/j.dyepig.2020.108940.
- Jiwalak, N., S. Rattanaphani, J. B. Bremner, and V. Rattanaphani. 2010. “Equilibrium and Kinetic Modeling of the Adsorption of Indigo Carmine Onto Silk.” Fibers and Polymers 11 (4): 572–579. https://doi.org/10.1007/s12221-010-0572-2.
- Kakuee, O., V. Fathollahi, P. Oliaiy, and S. Mesbahi. 2019. “Investigation of Mordants for Dyeing of Yarns in Ancient Persian Carpets (15th–17th Century) by IBA Methods.” Nuclear Instruments and Methods in Physics Research Section B: Beam Interactions with Materials and Atoms 450:294–298. https://doi.org/10.1016/j.nimb.2018.05.030.
- Kan, X. N., H. Liu, Q. Y. Pan, Z. B. Li, and Y. J. Zhao. 2018. “Anion-π Interactions: From Concept to Application.” Chinese Chemical Letters 29 (2): 261–266. https://doi.org/10.1016/j.cclet.2017.08.042.
- Karadag, R. 2023. “Establishing a New International Standard for Natural Dyed Textile Goods [Natural Organic Dye Standard (NODS)].” Journal of Natural Fibers 20 (1): 2162187. https://doi.org/10.1080/15440478.2022.2162187.
- Khayyun, T. S., and A. H. Mseer. 2019. “Comparison of the Experimental Results with the Langmuir and Freundlich Models for Copper Removal on Limestone Adsorbent.” Applied Water Science 9 (8): 9–16. https://doi.org/10.1007/s13201-019-1061-2.
- Leamkaew, V., P. Thongsamai, B. J. Bremner, and M. Chairat. 2022. “Adsorption kinetics of Lac Dye On Eri Silk Yarn.” The Journal of the Textile Institute 113 (11): 2480–2490. https://doi.org/10.1080/00405000.2021.1992912.
- Li, Y. F., S. Y. Wei, Q. J. He, and M. Fu. 2020. “Multi-Analytical Techniques Used for the Identification of the Dyeing Techniques of Several Textile of Ancient China.” Microchemical Journal 156:104790. https://doi.org/10.1016/j.microc.2020.104790.
- Ltaief, S., M. Jabli, S. B. Abdessalem, C. Joyeux, and O. Harzallah. 2023. “Sustainable Dyeing of Cotton Fibers Using Aqueous Extract of Citrullus Colocynthis Leaves: Chemical Characterization, and Dyeing Optimization Process Using Response Surface Methodology.” Journal of Natural Fibers 20 (2): 2198273. https://doi.org/10.1080/15440478.2023.2198273.
- Luca, E. D., G. Poldi, M. Redaelli, C. Zaffino, and S. Bruni. 2017. “Multi-Technique Investigation of Historical Chinese Dyestuffs Used in Ningxia Carpets.” Archaeological and Anthropological Sciences 9 (8): 1789–1798. https://doi.org/10.1007/s12520-016-0334-z.
- Morris, M. G., R. Huey, W. Lindstrom, M. F. Sanner, K. R. Belew, S. D. Goodsell, and J. A. Olson. 2009. “Autodock4 and AutoDocktools4: Automated Docking with Selective Receptor Flexibility.” Journal of Computational Chemistry 16 (16): 2785–2791. https://doi.org/10.1002/jcc.21256.
- National Standard of the People’s Republic of China. 2008a. GB/T 3920-2008, Textiles-Tests for Colour Fastness-Colour Fastness to Rubbing. Beijing: China Standard Press.
- National Standard of the People’s Republic of China. 2008b. GB/T 3921-2008, Textiles-Tests for Colour Fastness-Colour Fastness to Washing with Soap or Soap and Soda. Beijing, P. R. China: China Standard Press.
- National Standard of the People’s Republic of China. 2014. GB/T 30669–2014, Textiles-Tests Colour Fastness-Color Fastness to Light Yellowing. Beijing, P. R. China: China Standard Press.
- Nimkar, U. 2018. “Sustainable Chemistry: A Solution to the Textile Industry in a Developing World.” Current Opinion in Green and Sustainable Chemistry 9:13–17. https://doi.org/10.1016/j.cogsc.2017.11.002.
- Nimra, A., A. Shahid, R. Fazal, and N. A. Muhammad. 2023. “Environmental Friendly Utilization of Plant Wastes in Combination as a Source of Natural Colorants for Binary Mordanted Silk Dyeing.” Environmental Science and Pollution Research 30 (52): 112825–112835. https://doi.org/10.1007/s11356-023-30162-y.
- Okpareke, C. O., W. Henderson, R. J. Lane, and N. S. Okafor. 2020. “Synthesis, Structure, Computational and Molecular Docking Studies of Asymmetrically di-Substituted Ureas Containing Carboxyl and Phosphoryl Hydrogen Bond Acceptor Functional Groups.” Journal of Molecular Structure 1203:127360. https://doi.org/10.1016/j.molstruc.2019.127360.
- Ozdemir, M. B., and R. Karadag. 2022. “Madder (Rubia Tinctorum L.) as an Economic Factor Under Sustainability Goals in the Textile Dyeing.” Journal of Natural Fibers 20 (1): 2128968. https://doi.org/10.1080/15440478.2022.2128968.
- Pandiarajan, A., R. Kamaraja, S. Vasudevan, and S. Vasudevan. 2018. “OPAC (Orange Peel Activated Carbon) Derived from Waste Orange Peel for the Adsorption of Chlorophenoxyacetic Acid Herbicides from Water: Adsorption Isotherm, Kinetic Modelling and Thermodynamic Studies.” Bioresource Technology 261:329–341. https://doi.org/10.1016/j.biortech.2018.04.005.
- Revellame, D. E., D. L. Fortela, W. Sharp, R. Hernandez, and M. E. Zappi. 2020. “Adsorption Kinetic Modeling Using Pseudo-First Order and Pseudo-Second Order Rate Laws: A Review.” Cleaner Engineering and Technology 1:100032. https://doi.org/10.1016/j.clet.2020.100032.
- Roche, D. B., D. A. Brackenridge, and L. J. McGuffin. 2015. “Proteins and Their Interacting Partners: An Introduction to Protein–Ligand Binding Site Prediction Methods.” International Journal of Molecular Sciences 16 (12): 29829–29842. https://doi.org/10.3390/ijms161226202.
- Rym, M., D. Soufien, and S. Faouzi. 2020. “UV Protection and Dyeing Properties of Wool Fabrics Dyed with Aqueous Extracts of Madder Roots, Chamomiles, Pomegranate Peels, and Apple Tree Branches Barks.” Journal of Natural Fibers 19 (2): 610–620. https://doi.org/10.1080/15440478.2020.1758280.
- Salvestrini, S. 2018. “Analysis of the Langmuir Rate Equation in Its Differential and Integrated Form for Adsorption Processes and a Comparison with the Pseudo First and Pseudo Second Order Models.” Reaction Kinetics, Mechanisms and Catalysis 123 (2): 455–472. https://doi.org/10.1007/s11144-017-1295-7.
- Sawada, K. 2004. “Evaluation of the Dyeing Mechanism of an Acid Dye on Protein Fibers in Supercritical CO2.” Dyes and Pigments 63 (1): 77–81. https://doi.org/10.1016/j.dyepig.2004.01.008.
- Shen, B. Y., X. C. Shangguan, Z. P. Yin, S. F. Wu, Q. F. Zhang, W. W. Peng, J. G. Li, L. Zhang, and J. G. Chen. 2021. “Inhibitory Effect of Fisetin on α-Glucosidase Activity: Kinetic and Molecular Docking Studies.” Molecules 26 (17): 5306–5316. https://doi.org/10.3390/molecules26175306.
- Sledz, P., and A. Caflisch. 2018. “Protein Structure-Based Drug Design: From Docking to Molecular Dynamics.” Current Opinion in Structural Biology 48:93–102. https://doi.org/10.1016/j.sbi.2017.10.010.
- Vasanthakumari, D., V. L. Pushpa, and K. B. Manoj. 2019. “Cyclin Dependent Kinase 4 Inhibitory Activity of Thieno [2,3-D] Pyrimidin-4-Ylhydrazones – Multiple QSAR and Docking Studies.” Journal of Molecular Structure 1183 (1183): 263–273. https://doi.org/10.1016/j.molstruc.2019.01.089.
- Williams, T. N. 2022. “Mad about madder.” Nature Chemistry 14 (8): 966. https://doi.org/10.1038/s41557-022-01015-x.
- Wu, Y., H. J. Luo, and H. Wang. 2014. “Efficient Removal of Congo Red from Aqueous Solutions by Surfactant-Modified Hydroxo Aluminum/Graphene Composites.” Separation Science and Technology 2014 49 (17): 2700–2710. https://doi.org/10.1080/01496395.2014.942741.
- Xu, P., G. M. Zeng, D. L. Huang, C. Lai, M. H. Zhao, Z. Wei, N. J. Li, C. Huang, and G. X. Xie. 2012. “Adsorption of Pb(II) by Iron Oxide Nanoparticles Immobilized Phanerochaete Chrysosporium: Equilibrium, Kinetic, Thermodynamic and Mechanisms Analysis.” Journal of chemical engineering 203 (5): 423–431. https://doi.org/10.1016/j.cej.2012.07.048.
- Yang, H., L. Zhang, G. Y. Hou, and C. G. Liu. 2018. “Insights into the Effect and Interaction Mechanism of Bisphenol S on Lipids Hydrolysis in Sludge Through Multi-Spectra, Thermodynamics, and Molecule Docking Analysis.” Environmental Science and Pollution Research 25 (8): 7834–7843. https://doi.org/10.1007/s11356-017-1107-7.
- Zhang, F. X., C. Chen, G. X. Zhang, L. Zhong, Y. S. Zhang, and S. Tan. 2015. “The Accelerating Effect of a Small Cationic Quaternary Ammonium Compound and the Adsorption Kinetics in the Dyeing of Silk with Reactive Dyes.” Coloration Technology 131 (3): 259–267. https://doi.org/10.1111/cote.12150.